M.A. Hayat (ed.)Tumors of the Central Nervous SystemTumors of the Central Nervous System, Volume 132014Types of Tumors, Diagnosis, Ultrasonography, Surgery, Brain Metastasis, and General CNS Diseases10.1007/978-94-007-7602-9_16
© Springer Science+Business Media Dordrecht 2014
16. Resection of Brain Tumors: Intraoperative Confocal Microscopy Technology
(1)
Department of Neurosurgical Oncology, Barrow Brain Tumor Research Center, Barrow Neurological Institute, St. Joseph’s Hospital and Medical Center, Phoenix, AZ 85013, USA
Abstract
The ability to diagnose brain tumors in vivo and reliably identify tumor margins in the course of resection are two innovations that could impact the neurosurgical oncologist’s ability to maximize resection and minimize morbidity. Recent advances in optical imaging and miniaturization have enabled the production of a hand-held intraoperative confocal microscope. We present a first-look feasibility analysis of the intraoperative confocal microscope as an adjunct for brain tumor resection. Intraoperative confocal microscopy is an emerging and practicable technology for the resection of human brain tumors. Our preliminary assessment indicates the reliability of this technique for a variety of lesions in identifying tumor cell populations, as well as the tumor-brain interface, in situ. Further refinement of this technology may depend upon the approval of tumor-specific fluorescent contrast agents for human use.
Introduction
Maximizing the extent of resection is the primary objective of most surgery for brain tumors. Yet, normal tissue can only be distinguished from abnormal tissue definitively on pathological analysis conducted after surgery. For some extraaxial lesions, the gross appearance of the tumor is sufficient to establish the tumor-brain tissue planes with microdissection techniques. Other lesions, however, are less easily distinguished from normal brain tissue, particularly in patients who have undergone prior treatment or in those with cerebral edema or microscopic infiltration. This problem is particularly true with gliomas and higher-grade meningiomas, where defining extent of resection on the basis of gross tissue characteristics is insufficient and neuronavigation can be unreliable because of brain shift. To overcome these persistent challenges in the resection of complex intra- and extraaxial brain tumors, recent work has been directed toward adapting routine postoperative neuropathology methods into a real-time intraoperative technique.
Beyond identifying tumor margins, the opportunity to define tumor grade and histologic subtype intraoperatively is critical, particularly for intracranial gliomas, where tumor grade cannot be predicted reliably with either preoperative magnetic resonance (MR) imaging (Kondziolka et al. 1993) or stereotactic biopsy (Muragaki et al. 2008). Likewise, intraoperative frozen-section analysis can be misleading or nondiagnostic, especially when tissue is disrupted mechanically from the resection process (Tilgner et al. 2005; Uematsu et al. 2007). Such diagnostic unpredictability is further complicated by the inherent heterogeneity of gliomas, which can contain high-grade cellular populations nested within a low-grade stroma (Dowling et al. 2001). Collectively, these complexities represent a significant challenge for neurosurgical oncologists. However, they could be overcome by multiple in vivo optical biopsies during the course of tumor exposure and resection.
Intraoperative Confocal Technology
Intraoperative confocal microscopy is an emerging technology that enables the cytoarchitecture of live tissue to be visualized with spatial resolution on a cellular level (Becker et al. 1996; Khoshyomn et al. 1998; Tadrous 2000). Until recently, the size of requisite apparatus limited the use of confocal microscopy to examination of excised tissue samples or isolated cells in a laboratory. The latest incarnation of this technology, however, features fiber-optic and microscopic miniaturization, substantially expanding its portability and in vivo applicability in a clinical setting (Delaney et al. 1994; Flusberg et al. 2005, 2008; Helmchen 2002). A single optical fiber can be used as both the illumination point source and detection pinhole to acquire high-resolution images and to combine them with miniaturized scanning and optical systems (Hoffman et al. 2006).
Consequently, this device has recently been integrated into the distal tip of conventional video endoscopes and combined with intravenous fluorophores to screen the gastrointestinal mucosa for cancer (Kiesslich et al. 2005; Polglase et al. 2005). The bladder mucosa, skin, and eye have also been studied with in vivo confocal microscopy (Brezinski et al. 1998; Bussau et al. 1998; Koenig et al. 2001; Papworth et al. 1998). We have recently used a glioma model to study the capacity of handheld confocal imaging to discern microvasculature, the grey-white junction, and tumor margins in the rodent cortex (Eschbacher et al. 2012). This initial feasibility analysis of intraoperative confocal microscopy establishes its potential value during the microsurgical resection of both intra- and extra-axial tumors.
Histological Resolution
Examination of tissue integrity during confocal visualization demonstrates excellent preservation of cellular and subcellular structures. In normal and tumor parenchyma, large and small vasculature is evident and intact, both on the tissue surface and as deep at 500 μm to the surface. Within this microvasculature, the systolic and diastolic flow of erythrocytic transit can be appreciated. Notably, areas of neovascularization from tumor-induced angiogenesis distinguish tumor from adjacent normal tissue.
Glioma Visualization
Among World Health Organization (WHO) grade III and IV gliomas, vascular neoproliferation seen on confocal imaging enables both surgeon and neuropathologist to identify abnormal tissue reliably. In our experience, these areas of suspicion correlate well with both neuronavigational imaging and histological evidence of tumor. High-grade human glioma specimens are associated with specific confocal features, including neovascularization, dense cellularity, and irregular cellular phenotypes. Waves of varied density, likely corresponding to regions of necrosis, are also visible. Because, these features are evident to both surgeon and neuropathologist, the confocal microscopic findings can quickly be integrated into the operative plan.
Low-grade gliomas are also distinctive with intraoperative confocal microscopy. Although neovascular proliferation is less evident, distinctions in cellular density and morphology correspond with T2-weighted signal abnormalities on MR imaging. All specimens analyzed from regions where a confocal optical biopsy was obtained have demonstrated diagnostic patterns of low-grade histology on permanent sections. The pattern associated with pure oligodendrogliomas on confocal microscopy is distinct from other astrocytomas of comparable grade. However, mixed oligoastrocytomas do not appear to have a unique signature on intraoperative imaging and could not be reliably distinguished from low-grade astrocytomas.
Other Intra-axial Lesions
Other intraaxial lesions also have unique confocal signatures associated with their histologic phenotype. For central neurocytomas, in vivo imaging demonstrates uniform round cells organized in a honeycomb conformation, embedded against a background of arborized capillaries. Hemangioblastomas are similarly distinctive on confocal imaging and are identified by large stromal cells mixed with a dense capillary network. Within these tumors, both cystic changes and areas of microhemorrhage can be appreciated.
Meningioma Visualization
Meningiomas are common extraaxial lesions amenable to characterization with confocal microscopy. Fluorescein-enhanced confocal images of these tumors highlight fine histopathologic detail. The configuration of tumor cells in classic meningothelial meningiomas is largely uniform. The cells are organized as dense sheets with no evidence of whorls or psammoma bodies. In contrast, fibrous meningiomas contain cells with spindle-shaped morphology, which is easily distinguished from adjacent normal parenchyma. These tumors also contain fibrous bundles of matrix interlacing with adjacent tumor cells and dura.
Intraoperative Confocal Microscope: Emerging Applications
For a variety of tumor histologies, including gliomas, meningiomas, hemangioblastomas, and central neurocytomas, this handheld device generates a real-time, fluorescein-enhanced pathological image that is of sufficient resolution for a neuropathologist to establish a preliminary diagnosis. Mounting evidence supports the value of maximizing the extent of resection of many intracranial tumors, including gliomas (Sanai and Berger 2008). Consequently, several intraoperative techniques have been developed to help achieve this goal: intraoperative ultrasonography (LeRoux et al. 1989), intraoperative MR imaging (Alexander et al. 1997), optical spectroscopy (Toms et al. 2005), and fluorescent dyes such as 5-aminolevulinic acid (Stummer et al. 2006). However, none of these technologies can detect infiltrating tumor margins at a microscopic level. In contrast, our preliminary experience with intraoperative confocal microscopy not only suggests a correlation between imaging and tumor grade but has demonstrated a capacity to distinguish tumor margins from adjacent parenchyma.
Tumor heterogeneity and biopsy sampling error remain considerable sources of inaccuracy and primary causes of undergrading gliomas. The ease with which a handheld confocal microscope can generate numerous optical biopsies during the course of tumor exposure and resection may effectively neutralize such diagnostic imprecision. Furthermore, the ability of the device to scan as deeply as 500 μm through the visible tissue surface permits analysis of an even broader spectrum of tissue. This unique capability also raises the possibility of examining subependymal regions through an intraventricular corridor, allowing intraoperative confocal microscopy to detect pockets of subependymal tumor migration that are radiographically undetectable and remote from the primary tumor site. It has been postulated that subependymal spread is both a negative prognostic sign and a primary route of glioma migration (Lim et al. 2007). The ability to detect its occurrence at an early stage may permit anticipatory and focused interventions as well as lead to insights into basic mechanisms of gliomagenesis (Sanai et al. 2005).
Routine neuropathological diagnostic analysis depends on excision and processing of tumor tissue, which can be altered both mechanically and chemically during the analysis itself. Use of intraoperative confocal microscopy circumvents these limitations with real-time in situ visualization of tumor cytoarchitecture and microvasculature. The opportunity to observe active blood flow through tumor capillaries also provides a unique visual dimension to tissue assessment. The probe can quickly survey the visible extent of a tumor with the potential to detect disconnected, cell-dense islands that may portend worse histologic grade and heterogeneity. Future pilot studies will not only enhance our familiarization with this technique, but they may demonstrate a correlation with frozen section findings that could allow confocal microscopy to replace conventional intraoperative diagnostic methods. This modern approach to intraoperative diagnosis would also lend itself to remote, internet-based analyses, expanding the accessibility of diagnostic expertise beyond centers with dedicated tumor neuropathologists.
Future versions of the device may benefit from an auto-irrigation mechanism, similar to the endoscope. Furthermore, while fluorescein contrast allows detailed assessment of overall tissue cellularity, the ability to discern cellular cytoplasm remains limited, as does its specificity for nuclear morphology. Since tumor cell nucleus-to-cytoplasm ratios are critical to histopathological diagnosis, other contrast agents should be investigated. One candidate agent is 5-aminolevulinic acid (5-ALA), which allows highly specific localization of tumor cells. Currently, however, the technique is only available for clinical use in Europe.
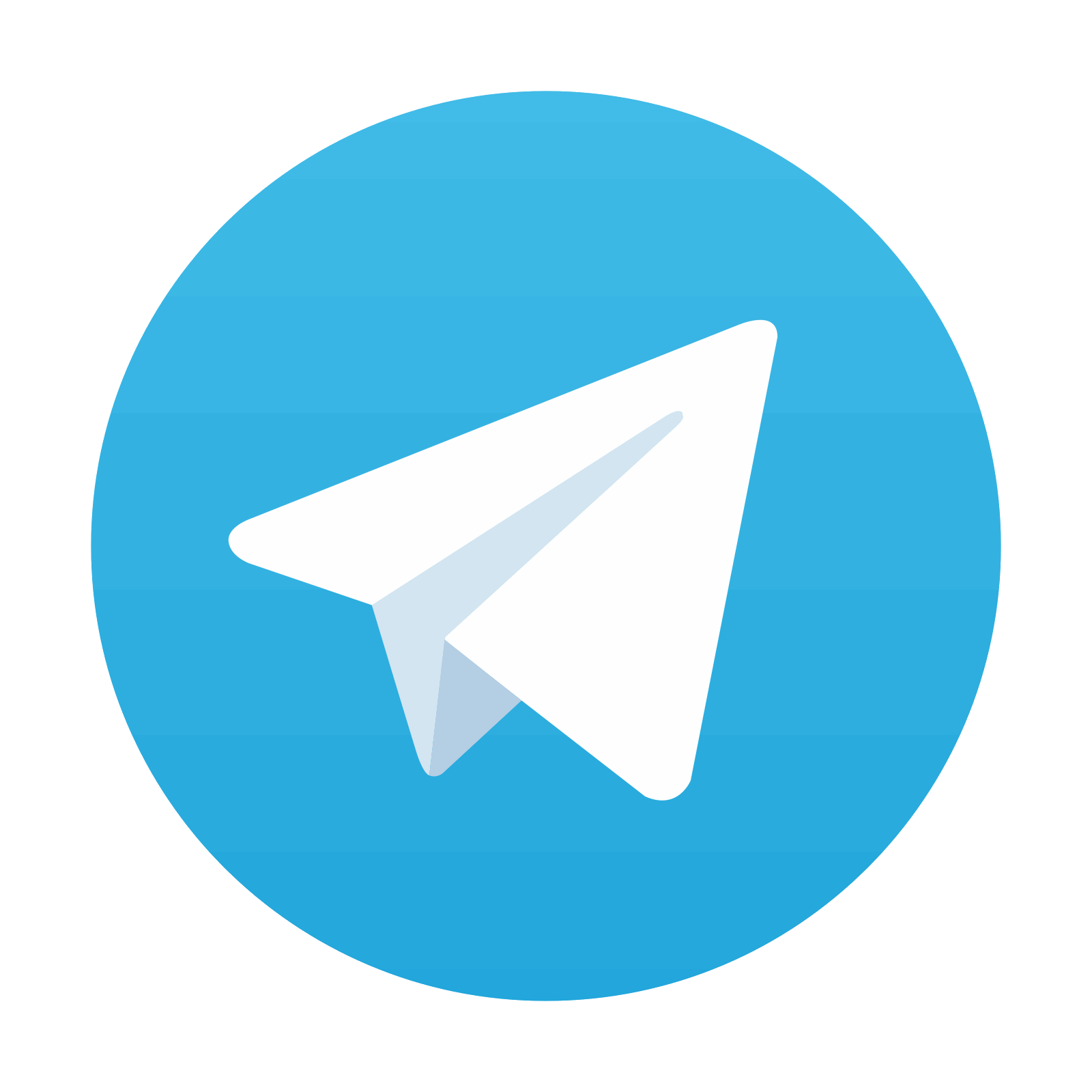
Stay updated, free articles. Join our Telegram channel

Full access? Get Clinical Tree
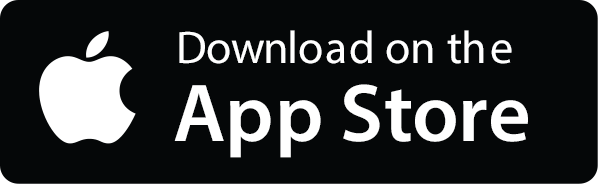
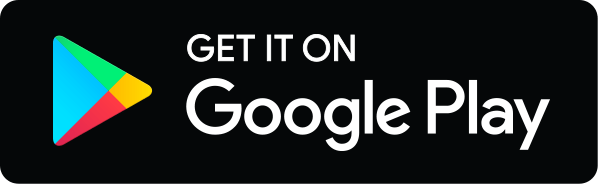