Fig. 4.1
(a) A 58-year-old female patient. Magnetic resonance imaging shows a contained central disk (C5–C6) herniation. Compression of the spinal cord is also due to a heavy inflammatory reaction swelling the epidural space (asterisk). In (b) imaging follow-up at 6-month post-coblation shows almost complete regression of both herniation and inflammatory epidural reaction; spinal cord is no longer compressed; patient is totally asymptomatic
4.4 Indications
Percutaneous decompression of disks has been shown effective in relieving radicular pain and to a lesser extent axial pain from contained disk protrusions. Patient selection criteria include the presence of a contained disk herniation documented by spinal imaging, causing radicular pain greater than axial pain. We do not treat patients for neck pain alone. Usually patients complain of cervical and upper limb pain, in most cases radiating down to the hand and often to the scapular, occipital, or auricular regions. Pain is most of the times accompanied by paresthesias radiating to the upper limb and hand. Preoperative clinical evaluation includes a neurologic examination, at which radicular deficits may be present, such as hyposthenia, hypoesthesia, dysesthesias, or hyporeflexia consistent with the radicular level compressed by the herniation. Also mild to moderate hypotropia of the muscular territory involved may be observed. Signs of moderate myelopathy, such as lower limb hyposthenia with pyramidal signs and sensory disturbances, do not contraindicate the treatment Fig. 4.2.


Fig. 4.2
(a) Magnetic resonance imaging at baseline for a 34-year-old female patient who underwent percutaneous plasma radiofrequency-based diskectomy for a disk (C6–C7) herniation compressing the spinal cord and causing clinical signs of myelopathy. (b) A 7-week MR follow-up shows regression of both herniation and cord compression; the patient is almost asymptomatic. (c) An MR 9-month follow-up shows almost complete regression of the disc herniation; the patient is completely asymptomatic
Disk herniations are known to have a tendency to self-heal. Spontaneous regression of herniated lumbar disks in the lumbar region is well established [51, 52], but the same phenomenon is increasingly observed in cervical disks as well [53–56]. For this reason the patient must have failed conservative measures, including anti-inflammatory and analgesic medications and physical therapy, for at least 2 months or longer.
Imaging and clinical correlation is of utmost importance. The success of the procedure depends greatly on selecting the lesions to treat: the protruding nucleus pulposus must be at least partially contained by the external fibers of the disk, without a large extrusion or migrated fragments [57, 58]. Thus patients receiving plasma radiofrequency-based disk decompression may carry a bulging, protruding, or extruded disk, which is not sequestered or migrated, compressing the exiting nerve root, thecal sac, or spinal cord. To this purpose, all patients must undergo preoperative both CT and MR studies of the cervical spine, the latter for its much higher diagnostic value, in terms of both herniation visualization and clear definition, and also of evaluation of its effects on neural tissue. CT must essentially rule out large osteophytes and/or large calcifications of the herniation itself, conditions that contraindicate coblation.
The disk should have maintained at least 50 % of its height on imaging studies. Disks with more advanced degrees of degeneration are more difficult to access and are less likely to achieve much further pressure reduction [44].
Given the low morbidity and invasiveness of the procedure, it can be offered to a wider range of patients, such as the ones with partially uncontained prolapses, as an attempt to avoid surgery, or when the risks of open surgery are higher because of age, general medical conditions, or other contraindications. This typically applies to patients who have already undergone open surgery at the same or, more frequently, at a different disk level and to elderly people. In these cases the patient must be disclosed the lesser likelihood of clinical success of the procedure.
4.5 Anatomical Considerations
The day before the procedure, we perform a preoperative sonographic study of the neck to determine whether any normal or pathologic structures residing near the surgical pathway are in a position to increase risk for complications [8, 59]. We also previously described [8] intraoperative use of sonography for percutaneous cervical decompression, and such technique helped us in understanding the behavior of local anatomical structures during the surgical maneuvers.
In the anterolateral area of the cervical region, several major anatomical structures deserve attention during percutaneous diskectomy. The trachea and esophagus are well depicted with sonography: we verified that they are easily displaced from the midline by even mild pressure and consequently most of the initial worries regarding esophageal puncture, with possible diskitis or mediastinitis, decreased. Similar remarks apply to the neurovascular bundle (carotid artery, vagus nerve, and jugular vein); this can be detected easily with sonography and is easily palpable and thus readily displaced laterally, while being protected by the operators’ left fingers.
The sympathetic chain lies posterolateral to the anterior convexity of cervical disks, so it cannot be involved by the trajectory of the devices. Several upper cervical nerves describe anteriorly concave loops related to mobility of the head, the lingual, IXth, and XIIth nerves, among them. The lowest of these loops goes down to the C3–C4 level: the superior laryngeal nerve, pointing toward the larynx between the hyoid bone and the thyroid gland. When we perform a procedure on the C3–C4 disk (although in rare cases), the superior laryngeal nerve is easily shifted beyond the midline together with all the neighboring anatomical structures (particularly the hyoid bone) and so removed from the surgical trajectory. The hypoglossal nerve loop is close to the neurovascular bundle, so that the remarks about carotid arteries also apply here.
A consideration of which structures most hinder cervical disk puncture should begin with the thyroid gland. Its isthmus lies roughly at the C6–C7 level, while the lobes extend upward for the height of two vertebral bodies, diverging from the midline. The gland is surrounded by a rich venous network and has superior and inferior vascular pedicles. While a venous injury can be treated by compression, puncture of the thyroid arteries should be avoided. The superior one arises from the external carotid artery and joins the superior vein to form the superior vascular pedicle, while the inferior, coming from the thyrocervical trunk of the subclavian artery, runs medially and reaches the inferior pole of the gland separately from the vertically running veins. The superior vascular bundle can be displaced from the midline by the hand displacing the carotid artery, as can be confirmed by color Doppler. This maneuver creates a space between thyroid gland and trachea through which the probe can easily be inserted; while operating at the upper disks (C3–C4, C4–C5), the superior lobe of the gland, which lies far from the midline, is displaced laterally and the instrument passes medial to it. The middle part of the thyroid gland is less mobile and closer to the midline but probably can safely be needled and probed because of the absence of important arteries. The inferior third of the gland cannot be pulled far laterally because of the isthmus; insertion of instruments lateral to it is risky, since the inferior thyroid artery crosses the inferior pole of the gland, running medially from the subclavian artery, and therefore lies at its lateral margin.
Near the inferior third of the thyroid lies another high-risk structure, the inferior (recurrent) laryngeal nerve, which originates from the vagus nerve in the upper thorax and runs along the trachea up to the inferior third of the thyroid, where it crosses the inferior thyroid artery Fig. 4.3. The inferior laryngeal nerve, injury to which causes a vocal cord paralysis, is detectable by sonography in a high percentage of cases [60], even in unfavorable intraoperative conditions; moreover, individual variations in the position of the nerve are exceedingly rare [59]. The considerable mobility of the inferior laryngeal nerve makes displacement from the trajectory of the instruments during manual displacement of the carotid artery and trachea probable. For these reasons, in our opinion, there is a “blind zone” at the inferior third of the thyroid gland, in which percutaneous approach to the intervertebral disk must be carefully considered. This zone is easily defined preoperatively by sonography; 3D-CT surface reconstruction of the neck may help preoperative planning by delineating the relationships between disks, vascular structures, and thyroid gland. The disk space most often related to the “shadow” of the inferior third of the thyroid gland is C6–C7. Yet we think that the protection of the nerve can be significantly increased by dilatation of soft tissues performed with coaxial mandrel with a trocar tip (see ahead), instead of a sharp needle. This mandrel does not have a sharp cutting effect, and structures are more likely to be deflected rather than resected. This feature limits the risk of vessel or nerve injuries. The use of intraoperative sonography can also help reduce the risks and rule out dangerous structures on the trajectory of the devices.


Fig. 4.3
Posterior view of the anatomical relationships in the neck: recurrent laryngeal nerves (small arrows) and inferior thyroid arteries (arrowheads) cross at the inferior pole of the thyroid gland (large arrows)
No significant risks for the spinal cord or nerve roots are posed by the procedure: the anterolateral approach does not involve crossing nerve roots, as in the lumbar spine, and this fact would permit performance of the procedure under general anesthesia. Fluoroscopic and/or CT guidance should preclude any risk of posterior displacement of the probe with involvement of the spinal canal and the spinal cord itself.
4.6 Surgical Technique
Patients receive a prophylactic intramuscular injection of 2 g cephalosporin 1 h prior to conducting the procedure. The procedure is performed under fluoroscopy using a C-arm unit, with the patient placed in supine position. The procedure is usually conducted under general anesthesia, although it is also doable under local anesthesia with sedation as necessary, particularly in fragile patients. The plasma diskectomy procedure is performed using an anterior approach. The head and neck are slightly hyperextended to facilitate access to the cervical disks. For better visualization of the lower cervical disks, it is beneficial to stabilize the shoulders. In these cases, traction is achieved by tying a belt to the patient’s wrists, and the belt is fixed to the foot of the table and pulled if necessary during the procedure, to uncover the lower disks from the shoulders.
The entry point for the port cannula is placed off-midline toward the patient’s right side in order to make an anterolateral approach. Local anesthetic is administered using a 22-gauge needle, inserted as deep as the annulus fibrosus; the anesthesia needle is positioned using an extended holder to reduce X-ray exposure to the surgeon’s hands (Fig. 4.4). While palpating vital structures away from the surgical pathway (the trachea is pushed across the midline, while the neurovascular bundle, the carotid artery in particular, and sternocleidomastoid muscle are maneuvered laterally and protected manually) (Fig. 4.5), a 19-gauge cannula with an internal mandrel is positioned against the anterior surface of the annulus fibrosus. Because the esophagus resides to the left of the midline, a relatively more right-sided approach is deemed safer at the more caudal cervical levels. The C-arm is positioned to gain a lateral view of the surgical field, and the cannula and mandrel are advanced under fluoroscopic guidance into the disk. The mandrel is replaced with the Perc DC SpineWand (Smith & Nephew, London, UK). The active electrode at the tip of the probe is less than 2-mm long and gently angled (Fig. 4.6). With the radiofrequency controller set at “3,” the SpineWand device is activated and then rotated 360° to create a cone-shaped void. The plasma radiofrequency-based process removes a small amount of tissue within the nucleus pulposus to achieve disk decompression. After 8 s of ablation, the device is repositioned to a different part of the nucleus, with the placement depending on the topography of the herniation. For both left- and right-sided lesions, voids are created along two channels. On the left side, the first channel is made in an oblique direction, from the right anterolateral entry point toward the left posterolateral herniation; the other channel is made on the midline and directed toward the posterior profile of the disk. For a right-sided lesion, the first channel is directed obliquely toward the left, crossing the center of the disk; the second channel is directed toward the right paramedian, along the medial surface of the uncal process to reach the herniation in the posterior aspect of the disk (Fig. 4.7). With these maneuvers, a total of 4–8 voids are made in the nucleus pulposus and inside the herniation itself. The ablation portion of the procedure takes less than 5–8 min for a single diskal level. After completing the ablation procedure, the SpineWand is withdrawn from the disk. A three-way stopcock is connected to the cannula, still resting with its distal end in the center of the disk. To the stopcock are connected a 20 cc syringe to one way and a 3 cc syringe with 40 mg Depo-Medrol to the other one. Aspiration is applied for few seconds with the 20 cc syringe, then the stopcock is turned, so that Depo-Medrol is aspirated inside the disk space. No pressure is applied to the 3 cc syringe to inject Depo-Medrol more than that simply aspirated. The cannula is then removed and manual compression is applied for a few minutes on the surgical site to favor hemostasis.





Fig. 4.4
(a) The 19-gauge cannula with an internal mandrel is positioned against the anterior surface of the annulus fibrosus. The cannula is held by a surgical forceps to minimize X-ray exposure of the surgeon’s hand. (b) Cannula placement as observed under fluoroscopy. (c, d) The cannula is advanced into the disk, and the SpineWand device is introduced into the nucleus pulposus via the cannula

Fig. 4.5
A schematic drawing shows the entry route, with the clinician’s fingers pushing the trachea across the midline while protecting the neurovascular bundle

Fig. 4.6
A sketch of the distal active end of the coblation bipolar probe. The active electrode is less than 2-mm long and gently angled. The device is activated and then rotated 360° to create a cone-shaped ablation void. Between 2 and 4 voids are ablated in a linear direction to create a channel

Fig. 4.7
After the first channel into the disk is completed, the device is repositioned to a different part of the nucleus, with the placement depending on the topography of the herniation. For left-sided herniations, the first channel is made in an oblique direction, from the right anterolateral entry point toward the left posterolateral herniation; the other channel is made on the midline and directed toward the posterior profile of the disk. For a right-sided lesion, the first channel is directed obliquely toward the center of the disk; the second channel is directed toward the right paramedian, along the medial surface of the uncal process to reach the herniation in the posterior aspect of the disk
The procedure can be performed on an outpatient basis, although we prefer to keep patients in the hospital overnight, mostly because of general anesthesia. When discharged, patients received a prescription for a nonsteroidal analgesic anti-inflammatory drug (to be taken for 7–10 days) and for a rigid neck collar, recommended to be used during the daytime for 2–3 weeks.
The introducer cannula for the SpineWand device has a 19-gauge caliber, probably the narrower among nonchemical procedures, laser excepted, and it is smaller than the needles currently used for direct puncture of the carotid artery or for diagnostic and interventional angiography. This cannula is easily inserted by means of a coaxial mandrel with a trocar tip. This is an important safety feature, since such mandrel does not have a sharp cutting effect, and structures are more likely to be deflected rather than resected. This feature limits the risk of vessel or nerve injuries. A 19-gauge puncture of the thyroid is of no clinical relevance, as confirmed by the wide experience in thyroid biopsies and carotid angiography.
In addition, unlike bevels, the symmetric trocar point follows a straight trajectory, allowing more precise positioning easily, particularly when driving the instrument into a relatively rigid tissue structure like the annulus fibrosus. Nevertheless, a thorough knowledge of the anatomy of the underlying structures of the neck intersecting the treatment pathway and a good quality fluoroscopy system are mandatory. Alternatively, the surgeon could choose to position the cannula under CT guidance and switch to direct fluoroscopy for the remainder of the procedure. The use of CT allows a better control of the position of the coblation probe, particularly when it must be positioned inside the spinal canal or foramen for treatment of larger herniations (Figs. 4.8 and 4.9).



Fig. 4.8
(a) Bilateral disk herniation at C6–C7 encroaching the spinal canal. The intervention was conducted under CT guidance for positioning the SpineWand device, switching to direct fluoroscopy during the ablation procedure. This allowed activation of the plasma-field energy directly inside the herniation. (b, c) The active electrode is beyond the posterior limit of the vertebral body, inside the spinal canal. (d) The trajectory of the device and gas from tissue excision (arrows)

Fig. 4.9
(a) Lateral disk herniation compressing the nerve root. (b) The cannula and SpineWand device are positioned under CT guidance and then safely and precisely directed toward the herniation. (c) Note gas from tissue ablation diffusing inside the herniation itself. (d) A 4-month CT follow-up shows partial regression of the lesion; the patient reports a definite clinical improvement
4.7 Clinical Results and Evidence
In February 2003, we began a prospective study of percutaneous plasma radiofrequency-based diskectomy for treating patients with symptomatic cervical disk herniation [61]. At 2 months, outcomes were good or excellent in 44/55 (80 %) patients; the success rate was similar at 6 months, when 44 (85 %) patients (n = 52/55) had good or excellent results. Seven patients (14 %) never showed improvement. One clinically relevant complication (infectious diskitis) occurred, which was successfully resolved. Three patients with clinical myelopathy experienced regression of cord compression symptoms; in two of these patients, magnetic resonance imaging showed morphological evidence of reduction of cord compression.
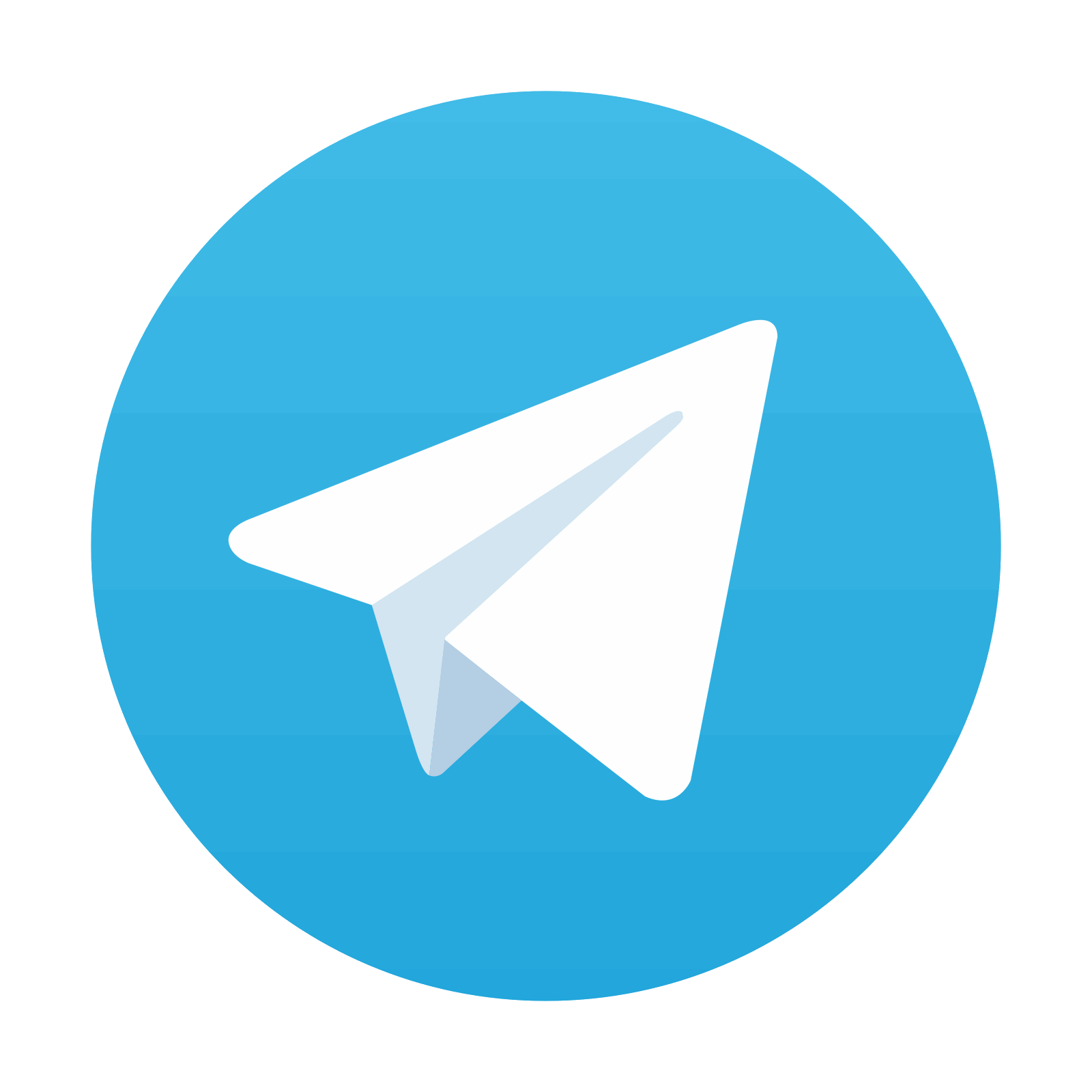
Stay updated, free articles. Join our Telegram channel

Full access? Get Clinical Tree
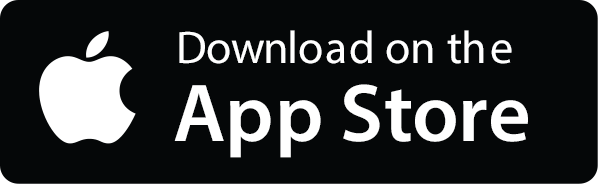
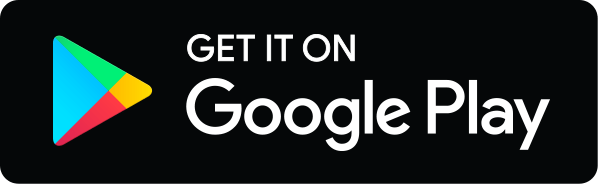