Introduction of Technology
Present Practice and Standard of Care
Pedicle screws have been placed to enhance spinal fusion since at least 1959. In that year, Boucher published a report detailing the placement of screws into the vertebral body via the pedicle with generally excellent fusion results. These early pedicle screws passed from the lamina of the superior level through the pedicle of the inferior level, thus achieving a state of internal fixation with addition of cancellous bone graft for fusion. In 1970, Roy-Camille described the first closed system involving interpedicular screws and plates ; in that system, pedicle screws functioned as bone anchors for a transpedicular plate running longitudinally along the spine. Since these early days, pedicle screws have seen a remarkable array of transformations in their design, placement techniques, and compatible systems for spinal fixation. The use of robotics to place pedicle screws forms but the latest chapter in a rich and fascinating history of innovation.
Two main phenomena are responsible for the pace of innovation that has led to the introduction of robotics in spine surgery. First, the placement of pedicle screws carries a risk of neurovascular compromise while traversing the spinal canal. A persistent theme in pedicle screw literature pertains to accuracy of placement, namely avoiding breaches through cortical bone. Second, the open exposure required for pedicle screw placement historically and frequently necessitates extensive soft tissue manipulation, raising the risk for injury, perioperative morbidity, infection, and spinal destabilization. Both of these drawbacks have driven the development of computer assisted navigation and percutaneous techniques to increase accuracy and decrease the invasiveness of pedicle screw placement. Robotic-assisted pedicle screw placement relies upon and combines both of these techniques.
Percutaneous fixation was first described by Magerl in 1984 via the use of intraoperative fluoroscopy. In particular, the “owl’s eye” view in the oblique plane along the barrel of the pedicle was meant to aid the surgeon in the percutaneous placement of a pedicle screw without the wide exposure traditionally necessary. These percutaneously placed screws were combined with an external fixation system, because the techniques to appropriately place rods in a minimally invasive fashion were still in their infancy. It was not until Foley and Gupta developed the Sextant system in 2002 (manufactured by Medtronic Sofamor Danek) that major advances were made in both virtual intraoperative navigation (via the use of the virtual fluoroscope) and percutaneous rod insertion. The adoption of three-dimensional virtual fluoroscopy (commonly referred to as computer assisted navigation) was first reported in 1997, and the validation of its accuracy in pedicle screw placement has been well reported. Advances in percutaneous placement via accurate computer-registered intraoperative imaging allowed the entry of the robot onto the spinal fusion landscape.
A robot is a machine that can replicate certain human functions. Generally, three different robotic systems are used in surgery: tele-surgical systems (where the surgeon directly controls a robot), supervisory-controlled systems (where the surgeon plans a task, and a robot performs it), and shared-control systems (where the surgeon and robot share task completion). The use of robots in spine surgery is unique when compared to the adoption of robotics in other surgical theaters. Robotic surgery in fields such as general surgery, urologic surgery, and gynecologic surgery has its origins in different needs, such as the visualization of previously difficult-to-reach areas. The adoption of robotic surgery in these fields relied on the successful development of the endoscope, which led to tele-surgical systems, such as the well-known da Vinci systems (Intuitive Surgical). Such systems aim to improve surgical capabilities, and the advantage of the robot is achieved by increasing manual function in an enclosed body cavity.
Robotic Systems in Spine Surgery
Modern spinal fusion involves the repetitive, minimally invasive surgical (MIS) placement of hardware that traverses sensitive neurovascular structures. The theoretical advantages of robots within this paradigm are to automate repetitive tasks, minimize surgical exposures, and increase accuracy by eliminating human error. The robotic systems used in spine surgery today generally involve shared-control systems in which an intraoperative plan is conceived by a robot’s imaging software, verified and modified by a surgeon, and performed in a shared fashion by the surgeon, who creates a percutaneous exposure and places hardware positioned by a navigated robotic arm. The computer assisted navigation for image acquisition and registration allows the robot to know where its effector arm is positioned relative to desired targets both theoretically (on the preoperative image) and in real life (on the patient). Although many systems have developed unique image registration and merging software, the basic innovation of robotic systems for spine surgery is the automation of screw positioning in preparation for drilling. While the positioning of cannulas, drill guides, and screws is automated, the surgeon must still prepare the tissue via skin and fascial incisions and operate the taps, drills, and screwdrivers.
Currently, robotic systems from three manufacturers have US Food and Drug Administration (FDA) approval for use in percutaneous pedicle screw placement. A brief overview of these systems is provided below and in Table 9.1 .
Robot | Manufacturer | FDA approval | Prior generations | Unique features |
---|---|---|---|---|
Mazor X Stealth Edition | Medtronic (formerly Mazor Robotics) | 2018 |
| Optic camera to avoid collisions between robotic arm and tools |
ROSA One | Zimmer Biomet (formerly Medtech) | 2019 | ROSA Spine | Adaptation for orthopedic use |
Excelsius | Globus | 2017 | – | Registration via fluoroscopy or intraoperative computed tomography |
Mazor Systems
The first robotic system to gain FDA approval was developed by Mazor Robotics (Caesarea, Israel) and is named the SpineAssist. This system is patient-mounted by fixation to a spinous process (if an open approach is used) or to the iliac crest via Steinman pins (if a percutaneous approach is used). The basic steps involved in its operation consist of (1) attainment of preoperative computed tomography (CT); (2) preoperative planning with screw trajectories and screw size mapped out via the system’s software on the preoperative image; (3) attachment of the mounting frame to the patient and placement of fiducial landmarks on the patient; (4) attainment of fluoroscopic images of the patient’s spine and synchronization of these images with the preoperative CT using the system’s software; and (5) alignment of the robotic arms to planned locations and subsequent placement of Kirschner wires (K-wire), dilators, taps, and screws.
The subsequent models included the Mazor Renaissance (FDA approval in 2011) and Mazor X (FDA approval in 2016). Both of these robotic systems featured improvements to deal with reported issues of the Mazor SpineAssist, such as skiving of the drill on fascia or bone. The Mazor X also features an optic camera to register the operative field to avoid collisions between the robotic arm and intraoperative tools.
In 2018, Medtronic (Dublin, Ireland) acquired Mazor Robotics and integrated their preoperative stealth navigation software with the Mazor X robot, forming the Mazor X Stealth Edition, which received FDA clearance in 2018.
Rosa One
The ROSA spine robot was developed by Medtech SA (Montpellier, France) and received FDA clearance in 2016. It consists of a floor-mounted station with an attached flexible robotic arm and a separate floor-mounted camera for capturing patient-mounted fiducials. The system operates by obtaining an intraoperative CT scan as the robotic arm places a fiducial box overlying the area of interest on the spine. The CT images are used for operative planning by the surgeon on a touchscreen attached to the robotic arm base. The robotic arm then moves into this predefined position because it recognizes spine landmarks from the overlying fiducial grid. The instruments are tracked during surgery by the fiducial system, and appropriate dilators, taps, and screws are placed. As with the Mazor system, the robotic arm moves each tool into position, but the drilling and screwing are performed by the surgeon.
In 2016, Zimmer Biomet (Warsaw, Indiana) acquired Medtech SA, and in 2019, FDA clearance was received for the ROSA One spine system. This robotic system can also be used for cranial applications under the ROSA One brain denomination. A ROSA One knee application is available for orthopedic use.
Excelsius
The Globus (Audubon, PA) Excelsius system received FDA clearance in 2017. Similar to the other systems, it consists of a mobile base with a robotic arm and screen for operative planning. A separate, mobile base involves a camera for the registration of fiducials for image merging. To operate the system, the fiducials are placed in the posterior superior iliac spine, and a grid called a dynamic reference base is overlaid above the skin attached to the iliac spine. The system can function either by taking intraoperative fluoroscopic images and merging them with a preoperative CT scan, or by registering an intraoperative CT scan. The surgeon then plans each screw trajectory and performs screw selection on the screen. The robotic arm then moves into position, and sequential dilators are placed. The steps of screw placement are performed by the surgeon, while the trajectory is maintained by the robotic arm.
Non–FDA-Approved Robots
Several robotic systems in use around the world have not been evaluated by the FDA for use in the United States. These include the Tianji Robot (Beijing Tinavi Medical Technology), which received clearance from the Chinese Food and Drug Administration in 2016. This system consists of two bases, namely a robotic arm base and a second base that contains an optical tracking camera for instrument tracking and registration, and a planning display. To operate it, the surgeon places a reference tracker on the spinous process, and a calibration screen is held by the robotic arm over the skin as two-dimensional C-arm images are obtained. These are registered with preoperative CT images. The surgical planning then proceeds using the system’s software where the surgeon views images of each level and selects screw trajectory, size, and length. The robotic arm then moves into the preselected position. Skin incisions are made, dilation is performed via sequential dilators, and cannulas are held in place by the robotic arm. Drilling and screw insertion are performed with tracked, navigated instruments that are controlled by the surgeon but guided by the guides held by the robotic arm.
Current Outcomes
A wealth of literature reports on the comparison between robotic systems, free-handed approaches, and navigated approaches in the placement of pedicle screws. A summary of the pros and cons of robotic, free-handed, and CT-guided screw placement techniques is provided in Table 9.2 .
Robotic | Free-hand | CT-guided | |
---|---|---|---|
Pros |
|
|
|
Cons |
|
|
|
Accuracy of Pedicle Screw Placement
The most widely used system for comparing the approaches for accuracy of pedicle screw placement in the literature on robotic systems is the Gertzbein-Robbins classification, which delineates five separate grades (A–E) based on whether the screw is entirely within the pedicle (A) or breaching the medial cortical wall by less than 2 mm (B), less than 4 mm (C), less than 6 mm (D), or greater than 6 mm (E). Grades A and B are generally clinically acceptable.
The safety of robotically placed pedicle screws has been well-established in a multitude of studies. Several caveats apply when examining the literature as it pertains to accuracy for robotically assisted spine surgeries: the accuracy criteria are heterogenous, purely percutaneous approaches are rare and often combined in studies with open approaches, indications are manifold, and studies usually examine only one robotic system. However, several generalizations are possible. The reported accuracy rates for purely percutaneous robotically placed pedicle screws generally range between 97% and 98%. An early, large, retrospective review of 3271 robotically placed pedicle screws found a “clinically safe” placement (Gertzbein-Robbins A or B) in 98.3% of screws. This compares favorably to reported accuracy rates of 90.7% and 90.6%, respectively, for more than 15, 358 pedicle screws placed in vivo using both nonnavigated and navigated assistance with no robotic control. When compared directly in one retrospective review, 93.4% of 410 robotically placed screws were Gertzbein-Robbins A or B versus 88.9% of 397 placed in a free-handed group, a result that was statistically significant ( P = .005).
Large meta-analyses corroborate the safety and even the superiority of robotic-guided screw placement. A comparison of publications examining robotic versus free-handed techniques found that robotically placed screws were more accurate when judged as being completely within the pedicle compared to free-hand screws (OR 95% CI: 1.38–2.07, P < .01). Another recent large meta-analysis of randomized controlled trials determined that screws placed using the Mazor Renaissance robot were no more accurate along Gertzbein-Robbins A criteria (RR = 1.00, 95% CI 0.96–1.05, P = .95) than were traditional free-handed techniques, but that those placed by the Tianji robot were more accurate (RR = 0.46, 95% CI 0.28–0.75, P < .01).
In one randomized controlled trial comparing 74 robotically placed pedicle screws to 82 placed with conventional, free-handed open techniques, there was no statistically significant difference in screw placement accuracy. However, robotically placed screws were less likely to violate the proximal facet joint than those placed free-handed. Another study with 30 patients each in one of two arms—a percutaneous robotic and open fluoroscopic arm—found no difference in accuracy of placement but found greater distance from the proximal facet in robotically placed screws. A detailed assessment of accuracy was undertaken in one study comparing purely percutaneous robotically placed screws and standard open fluoroscopic guidance for spinal fusion in patients with metastatic spine disease. Although the rates of clinically acceptable (Gertzbein-Robbins A or B) screw placements did not differ between the two groups (84.4% of 192 screws vs. 83.6% of 214 screws), in those screws that were malpositioned, medial errors prevailed with the robot, whereas lateral errors prevailed in the free-hand group.
Notably, several publications report the inferior accuracy of robotically placed screws. One early prospective randomized controlled trial found that the free-handed technique achieved superior rates of accurate screw placement compared to robotically assisted placement using the Mazor SpineAssist robot. In this study, 93% of 152 free-handed screws were classified as Gertzbein-Robbins A or B, versus only 85% for 146 robotically placed screws. The authors postulated that the especially low rate of accuracy in the robotic arm was due to the use of a bed-mounted robot and a postulated high risk of skiving, as the entry point is located on the downslope of the facet. One study directly compared 24 open versus 6 percutaneous robotic procedures and found a higher breach rate with percutaneous procedures, postulating that this may be due to the inability to grind down the facet at the screw entry site in percutaneous procedures.
Because of their early introduction, the Mazor and ROSA systems are the most studied. However, several recent publications testify to the similarly safe and highly accurate use of the Excelsius system, with reported breach rates of 1.6% of 3.4% in two separate retrospective studies. A summary of the prospective randomized controlled trials examining robotic placement of pedicle screws is provided in Table 9.3 .
Study, year | Robot | N (screws) | Intervention | Accuracy | Other Findings |
---|---|---|---|---|---|
Ringel et al., 2012 | Mazor SpineAssist | 146 robot, 152 free-hand | Lumbar and sacral screws | Robotic 85%, free-hand 93% (GR A or B) | Free-hand: less operative time |
Roser et al., 2013 | Mazor SpineAssist | 72 robot, 40 free-hand, 36 navigation | Lumbar screws | Robotic 99%, free-hand 97.5%, navigation 92% (GR A) | |
Hyun et al., 2017 | Mazor Renaissance | 130 robot, 140 fluoro | Lumbar screws | Robotic 97.7%, fluoro 95% (GR A) | Robotic: less radiation, shorter LOS, same operative time, same VAS and ODI scores |
Kim et al., 2017 | Mazor Renaissance | 158 robot, 172 free-hand | Lumbar screws | Robotic 93.7%, free-hand 91.9% (GR A) | Robotic: longer operative time |
Park et al., 2018 | Mazor Renaissance | 37 patients robot, 41 patients free-hand | Lumbar screws | Not reported | Both groups: significant improvement in VAS back VAS leg, ODI postsurgery |
Han et al., 2019 | Tianji TiRobot | 115 robot, 119 fluoro | Thoracic and lumbar screws | Robotic 95.3%, fluoro 86.1% (GR A) | Robotic: less blood loss, less radiation exposure, same operative time, same LOS |
Li et al., 2019 | Orthobot | 32 robot, 50 free-hand | Lumbar screws | Robotic 90.6% GR A, 9.4% B; free-hand 78% A, 20% B | Robotic: shorter radiation time |
Fan et al., 2020 | Tianji TiRobot | 186 robot, 204 fluoro | Cervical screws | Robotic 87.6%, fluoro 60.8% (GR A) | Robotic: less blood loss, shorter LOS |
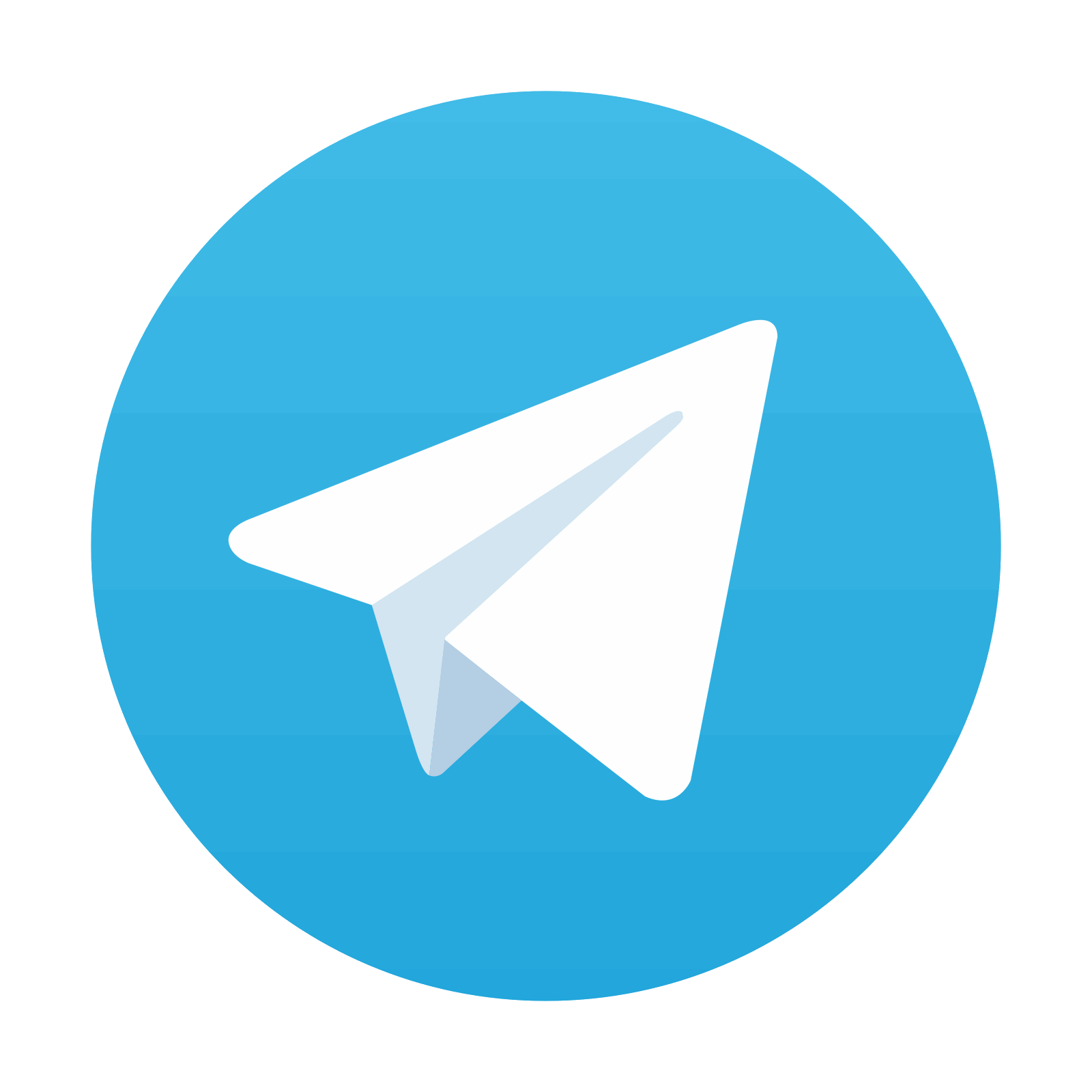
Stay updated, free articles. Join our Telegram channel

Full access? Get Clinical Tree
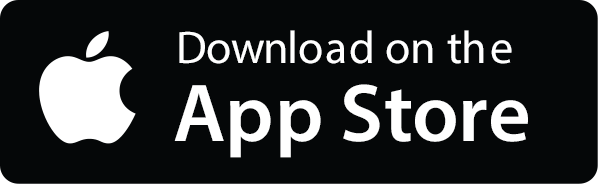
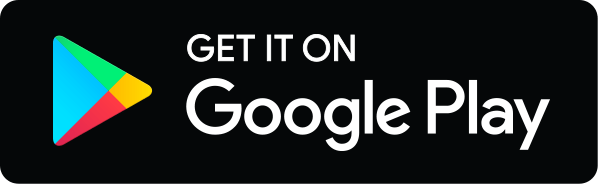
