Abstract
Neuroblastoma is a solid embryonal tumor of the sympathetic nervous system. The clinical course of patients with neuroblastoma is highly heterogeneous, from localized tumors and spontaneous regression to early metastasizing, rapid progression, and therapy resistance. This clinical heterogeneity can be attributed largely to the interaction of multiple genetic and epigenetic factors, including both sequence and copy number variants that occur in neuroblastoma cells. Recent methodological advances have enabled the detailed analysis of the neuroblastoma genome, leading to the identification of new prognostic markers and better patient stratification.
Amplification of MYCN gene and DNA ploidy have been used as the most reliable indicators of aggressive and treatment-resistant neuroblastoma, yet few of the high-risk tumors lack these features [1]. Candidate tumor suppressor genes have been identified on chromosomes 1p, 3p, and 11q that are deleted in some neuroblastomas. Despite extensive knowledge of somatically acquired genomic rearrangements in neuroblastoma and their association with the clinical tumor phenotype, very few data are available about the factors leading to these genetic events. While genetic alterations are almost impossible to reverse, epigenetic changes can dynamically respond to signals from physical, biological, and social environments [2]. New functional studies suggest that microRNAs regulate important genes involved in neuroblastoma pathogenesis [3].
Keywords
DNA ploidy, Epigenetic regulation, MYCN oncogene amplification, Neuroblastoma, Non-coding RNA
Introduction
Neuroblastoma is a sympathetic nervous system malignant tumor, which is derived from the neural crest cells and constitutes 7%–11% of the total number of childhood malignant tumors, taking fourth place in the structure of cancer morbidity after acute leukemia, central nervous system tumors, and malignant lymphomas. The incidence of neuroblastoma constitutes 0.85–1.1 cases per 100000 children under 15 years . The disease age distribution is heterogeneous; the frequency of tumor detection decreases with age, and 90% of patients are newborns and children up to 6 years. In children older than 14 years, neuroblastoma occurs rarely. Despite the comparatively low level of the disease, about 15% of pediatric cancer deaths are associated with neuroblastoma .
Unrestrained growth is the hallmark of the cancer cell. Carcinogenesis proceeds through the accumulation of genetic and epigenetic changes that allow cells to break free from the tight network of controls that regulate the homeostatic balance between cell proliferation and cell death . This property is caused by abnormal regulation of genes, which are involved in the control of cellular proliferation and cell death. Other disturbed cellular functions important for the evolution of cancer involve the control of DNA integrity, angiogenesis, and cellular senescence . It appears that the cause of these functional disturbances is mainly genetic rearrangements, although recently there is greater awareness of the possibility that stable epigenetic defects may contribute significantly to tumorigenesis, especially, in the case of childhood tumors of embryonic origin.
A characteristic feature of neuroblastoma is its clinical heterogeneity — from localized tumors to widespread forms and early hematogenous metastasizing. This high clinical heterogeneity reflects the complexity of genomic abnormalities characterized in neuroblastoma tumors . As a genetically complex cancer, neuroblastoma also displays significant genetic heterogeneity. That is why strikingly different outcomes are observed across tumor subtypes — from spontaneous regression without therapy to rapid progression and death due to the disease .
Individual tumors show great heterogeneity in their patterns of genetic alterations, epigenetic changes, and gene expression, even within homogenous histological groups . In addition, the fact that malignant phenotypes can be maintained solely by a tiny subpopulation of cells with stem cell properties , have proved that tumor heterogeneity is not merely a consequence of mutation acquisition, leading to a clonal expansion of mutated cells. Thus, it is now clear that there are multiple mechanisms by which cells can progress into malignancy, and that it is the concerted accumulation and functional cooperation between genetic and epigenetic changes, rather than their order of occurrence that drives carcinogenesis .
The role of MYCN gene amplification in neuroblastoma pathogenesis was first established in the early 1980s due to its association with high-risk tumors and low patient survival . Since then, several other genetic abnormalities were associated with neuroblastoma, including gains of whole chromosomes and a large number of large-scale chromosomal imbalances, such as loss of heterozygosity (LOH) at chromosome arms 1p, 3p, 14q, and 11q, unbalanced gain of 1q, 11p, and 17q and numerous mutations in key genes such as ALK, PHOX2B , and PTPRD .
Despite the extensive knowledge of the somatically acquired genomic rearrangements in neuroblastoma and their correlation with the clinical tumor phenotype, very little is known about the factors leading to these genetic events. Some changes in early embryogenesis or germ line are probably necessary for the development of neuroblastoma. A recent study has shown significant opportunities for epigenetic factors to promote carcinogenesis, especially in cases of childhood tumors with embryonic origin . The research on epigenetics has become increasingly visible because of the remarkable progress in our understanding of the critical role of epigenetic mechanisms in normal cellular processes and the abnormal events that lead to diseases, most notably, to cancer. Thus, genetic and epigenetic events represent two complementary mechanisms that are involved in every step of carcinogenesis, from responses to carcinogen exposures to progression into malignancy. However, we do not know what initiates this process in most cases, except in some inherited forms of cancer and some types of adult cancer in which environmental factors play a crucial role .
Epigenetics is defined as the study of heritable changes in the functioning of genes that occur without changes in the DNA sequence. Epigenetic modifications consist mainly of DNA methylation, histone modification, chromatin reorganization, and expression of non-coding RNA. Epigenetic modifications are well known as regulators of tissue-specific gene expression, genomic imprinting, and X-chromosome inactivation . In addition, the key role of epigenetic modifications during cellular differentiation, development, and organogenesis has been highlighted by the identification of many epigenetic biomarkers in human diseases, such as neuroblastic tumors .
The occurrence of many cancers is the result of the accumulation of genetic and epigenetic changes. While genetic alterations are nearly impossible to reverse, epigenetic changes can dynamically respond to signals from the physical, biological, and social environments . This characteristic confers the importance of epigenetic research in various cellular processes, particularly, in the regulation of gene expression. Although epidemiological data provide evidence that there is a direct interaction between epigenetic modifications and environmental influence on gene expression, the mechanism of epigenetic induced modulations of gene expression is still poorly understood .
Numerous studies have demonstrated that genomic and transcriptomic profiles can be predictive for clinical disease course, so that the combination of mRNA, miRNA, and comparative genomic hybridization are now being used to better define prognostic markers that could provide insight into the molecular basis of clinical heterogeneity in neuroblastoma . It is reflected in the International Neuroblastoma Risk Group (INRG) Staging System, which takes into account both clinical characteristics and tumor biology to identify clinical risk groups with statistically different survival rates. Independently prognostic baseline characteristics of this system included patient age, stage of disease, histology, grade of differentiation, DNA index, MYCN gene amplification, and presence of chromosome 11q copy number aberrations .
Genetic Alterations in Neuroblastoma Pathogenesis
In cancer cells, somatic mutations occur and accumulate at a significantly higher rate than in normal cells, a property referred to as “Mutator Phenotype.” This ability of cancer cells to accumulate mutations is critical for the development of cancer as well as for the rapid development of resistance to cytotoxic cancer treatments . The Mutator Phenotype can be caused by a number of mechanisms, such as defects in cell-cycle regulation, apoptosis, specific DNA repair pathways, or error-prone DNA polymerase, and it can have its source in inherited genetic defects that make the subjects prone to specific cancers.
Mutations in cancer cells cover a wide range of structural alterations in DNA, including changes in chromosomes copy numbers or chromosomal alterations encompassing millions of base-pairs such as translocations, deletions or amplifications, as well as smaller changes in nucleotide sequences such as point mutations affecting a single nucleotide at a critical position of a cancer-related gene . These different kinds of alterations often coexist within a single tumor.
While the origins of neuroblastoma tumorigenesis arise from the disrupted development of neural crest precursors, after the DNA and RNA sequencing of over 1000 cases, no single genetic or epigenetic mutation has been found to account for all cases of neuroblastoma . Likewise, structural genomic changes have not been linked to neuroblastoma tumorigenesis. For example, 1p deletion, MYCN amplification, or gain of 17q may identify subtypes of neuroblastoma and impact survival , yet there is no common neuroblastoma-specific genomic alteration, LOH, or genetic translocation uniformly ascribed to all high-risk neuroblastoma tumors. Thus, this extensive molecular heterogeneity supports the concept that neuroblastoma represents a spectrum of diseases. Clinically, this presents a challenge as tumors that are phenotypically and morphologically very similar can have highly disparate responses to treatment. Consequently, extensive efforts have focused on characterizing the transcriptomes and oncogenic pathways, which are active in the most aggressive and fatal subtypes . In addition to elucidating the genetic and epigenetic origins of neuroblastoma, these efforts are motivated by the potential to yield actionable therapeutic targets for this highly fatal cancer. Over the last three decades, many chromosomal and molecular abnormalities have been identified in patients with neuroblastoma. These biological markers have been evaluated to determine their value in assigning prognosis, and some of these have been incorporated into the strategies used for risk assignment.
Ploidy
According to their DNA index, neuroblastomas can be divided into a group with a near-diploid nuclear DNA content (about 45% of neuroblastomas) and those with a near-triploid DNA content (about 55%). DNA index is a prognostic marker for patients younger than 2 years who have disseminated disease . This genetic subtype of tumor is frequently seen in patients of less than 1 year of age, where tumors are localized and have a good prognosis .
It was suggested that a near-triploid DNA content, which is found more often in localized or metastatic neuroblastomas, was because these tumors have a fundamental defect in mitosis leading to gains or losses of whole chromosomes. On the other hand, locoregional or metastatic tumors with a near-diploid DNA content have a fundamental defect in genome stability leading to chromosomal rearrangements such as unbalanced translocations .
Many neuroblastoma tumors display DNA diploid status and bear partial gains, losses, amplification, or other structural chromosome aberrations. Recurrent structural chromosomal alterations commonly associated with advanced stage of disease and poor outcome in neuroblastoma include MYCN amplification, deletion of chromosome arms 1p, 3p, 4p, and 11q, and gain of chromosome arm 17q .
MYCN
MYCN oncogene plays a major role in neuroblastoma tumorigenesis and defines an aggressive subset of tumors. MYCN oncogene (located on chromosome 2p24; ‘N′ stands for neuroblastoma-derived) was found to be amplified in 20%–25% of neuroblastomas, and is usually present in the form of double-minutes (chromosome fragments) or homogeneously staining regions . MYCN gene encodes a transcription factor that forms heterodimers with the MAX protein . Evidence for a direct involvement in the development of neuroblastoma was obtained through the construction of a mouse neuroblastoma model in which a human MYCN cDNA was placed under the control of a tyrosine hydroxylase promoter, leading to the development of this tumor . So, overexpression of MYCN alone was sufficient to initiate neuroblastoma formation in mice.
This transcription factor both activates and represses genetic targets (e.g., mRNA, miRNAs, lncRNAs) through direct DNA binding as well as indirect protein/protein interaction mechanisms . Both MYCN and MYCC (C-MYC) have well-described anti-p53, pro-proliferative functions, and pro-epithelial-mesenchymal transition (EMT) functions . During normal embryogenesis and neural crest development, MYCN is transiently expressed in the ventral-lateral migrating crest cells destined to become sympathetic ganglia . Thus, it is not surprising to find high levels of MYCN in a subset of poorly differentiated aggressive neuroblastomas . Numerous studies focusing on identifying the signaling pathways influenced by MYCN have established that high level of it enhances the expression of several genes involved in cell proliferation, and also represses expression of differentiation- and apoptosis-related genes either in a direct or indirect fashion . Targets directly induced by MYCN include the high mobility group A ( HMGA1 ) , the minichromosome maintenance complex component 7 , the Mdm2-p53 binding protein homolog ( MDM2 ) , p53 , and the multidrug resistance-associated protein ( MRP1 ) .
Despite this, MYCN status cannot predict all cases of poor survival in neuroblastoma, and 80% of neuroblastomas do not display MYCN amplification . Interestingly, high MYCN target gene expression is not restricted to MYCN amplified neuroblastomas but is also apparent in high-stage MYCN non-amplified tumors, indicating that common pathways are altered in high-stage tumors . A functional 157-gene signature in neuroblastoma consisting of relevant genes that are regulated by MYCN and predictive of outcome was revealed. Interestingly, a subgroup of the tumors displaying this signature and the poor outcome did not have MYCN amplification or high MYCN mRNA levels, but high nuclear MYCN protein levels . It suggests that the aggressive phenotype of MYCN might not only be associated with MYCN c opy numbers, but with other signals that regulate MYCN expressions, such as RNA binding proteins and microRNAs. However, many high-risk cases have a minimal MYCN expression, suggesting additional mechanisms for tumorigenesis independent of MYCN deregulation .
ALK
Familial neuroblastoma is a rare event, as it only accounts for 1%–2% of cases. Inheritance seems to follow an autosomal dominant pattern with incomplete penetrance . Inherited mutations in the homeodomain transcription factor, paired-like homeobox 2B ( PHOX2B ) and the anaplastic lymphoma kinase ( ALK ), have been reported to predispose to familial neuroblastoma .
Activating mutations of ALK are also implicated as oncogenic drivers of neuroblastoma . Mutations are found in almost all cases of familial neuroblastoma (<1% of total neuroblastoma cases) and between 6% and 10% of spontaneous cases . DNA amplification and protein overexpression, as well as activating point mutations of ALK , have been described in neuroblastomas . ALK is linked to sympathetic neuron development and survival of migratory neural crest cells . This gene is an important regulator of stem cell functions, including STAT3 dependent self-renewal, and as a transcriptional target of MYCN, high expression predicts poor outcome .
A recent study demonstrated that ALK F1174L, which is the most frequent and aggressive ALK mutation, was sufficient to promote neuroblastoma development in mice. In addition, when ALK F1174L and MYCN were coexpressed, a synergic effect was displayed in tumor development. Interestingly, these tumors had minimal chromosomal aberrations, suggesting that these two genes were sufficient to drive neuroblastoma formation .
PHOX2B
Germ line mutations of PHOX2B are found in a subset of familial neuroblastoma and about 4% of sporadic cases . PHOX2B and PHOX2A drive differentiation of neural crest precursors toward sympathetic neurons . Missense or frame-shift mutations in the homeodomain of PHOX2B were described in a rare subset of neuroblastomas with congenital central hypoventilation syndrome (CCHS). Recently, PHOX2B loss-of-function mutations have been shown to block neuroblastoma differentiation by disrupting calcium regulation . PHOX2B may also inhibit ALK expression in neuroblastoma ; although PHOX2B could give selective advantages to tumor cells, it is likely not sufficient to drive neuroblastoma pathogenesis.
Chromosome 1p Loss
Deletion of the short arm of chromosome 1 has been detected in approximately 25%–35% of primary neuroblastomas . This aberration is frequently associated with amplification of MYCN , is found in approximately 70% of aggressive neuroblastomas , and it has been reported that 1p LOH is independently associated with poor outcome in patients with localized tumors .
This finding suggests the presence of one or more tumor suppressor gene(s) in this chromosome region. In the past, many attempts were made to delineate the shortest region of deletion on 1p36 . It is currently defined to 1p36.31 (a region of approximately 2 Mb) . One of the most promising candidate neuroblastoma suppressor genes in this region is Chromodomain Helicase DNA binding protein 5 ( CHD5 ). This gene is a member of the chromatin remodeling family and is expressed mostly in the nervous system . The mouse Chd5 gene was found to control proliferation, senescence, and apoptosis through the p19Arf-p53 pathway and was therefore validated as a mouse tumor suppressor gene . Fujita et al. found that human CHD5 expression was low in neuroblastoma cell lines and in tumors harboring a chromosome 1p deletion. In addition, their data strongly suggested that inactivation of the second allele of CHD5 in neuroblastoma occurs by means of epigenetic silencing by CHD5 promoter hypermethylation. Based on the positive correlation between 1p LOH and MYCN amplification found in neuroblastoma tumors, the authors suggested that CHD5 promoter methylation could be an MYCN -mediated effect .
Recently, CAMTA1, a transcription factor mapping to 1p36, was also identified as a tumor suppressor gene in neuroblastoma . Expression of CAMTA1 induces the transcription of genes involved in neuronal differentiation and inhibits genes related to cell proliferation. In addition, subcutaneous inoculation of athymic nude mice with CAMTA1-inducible neuroblastoma cells resulted in a significant reduction of the tumors, demonstrating a role for CAMTA1 as a tumor suppressor in an in vivo model .
The zinc-finger transcription factor CASZ1, located on 1p36.22, has also been suggested to play a role in cell differentiation. Consistently, restoration of CASZ1 in neuroblastoma cell lines induced the cell differentiation, enhanced cell adhesion, and suppressed cell growth . Subsequent studies demonstrated that silencing of the second allele of CASZ1 was mediated by the aberrant upregulation of the polycomb protein histone methyltransferase EZH2 (enhancer of zeste homolog 2), which regulates differentiation in many tissues .
Chromosome 17q Gain
The gain of chromosome 17q is the most common genetic aberration, occurring in approximately 80% of neuroblastomas . Frequently, this aberration is caused by unbalanced translocations of segment 17q21-qter and the distal part of chromosomes 1p or 11q , although other chromosomes can also be involved in 17q gains . The involvement of chromosome 1 and chromosome 17 in the etiology of neuroblastoma was further reinforced by the identification of a constitutionally balanced t(1; 17) translocation in a patient with neuroblastoma . The chromosome 17q breakpoints are not confined to one cytogenetic band but are scattered throughout the long arm of chromosome 17 . Based on these findings, the presence of one or more dosage-sensitive genes on 17q implicated in neuroblastoma seems the most plausible hypothesis. Near-triploid tumors have often gained an extra whole chromosome 17, whereas unbalanced 17q gain is characteristic for near-diploid tumors.
Chromosome 17q gain was found to be the parameter for poor outcome, whereas whole chromosome 17 gain was associated with good prognosis . Numerous studies have reported that 17q gain is significantly associated with advanced stage of disease, increased patient age, 1p LOH, 11q LOH, and MYCN amplification . However, the independence of 17q gain as a prognostic factor is controversial. Whether an independent prognostic factor or merely a modifying factor, identifying the gene aberrations caused by 17q unbalance will be crucial for fully understanding the neuroblastoma progression.
Chromosome 11q Loss
Another common structural chromosome aberration associated with aggressive clinical behavior is 11q LOH, occurring in approximately 40%–45% of neuroblastoma cases . Although inversely correlated with MYCN amplification, a small subset of tumors display both an 11q LOH and MYCN amplification . Numerous studies suggest that MYCN amplified and 11q LOH represent two distinct subtypes of neuroblastoma tumors, both of which can be associated with poor clinical outcome . As a result, in 2009, aberrations of chromosome 11q were included in the INRG classification system .
Therefore, identifying genes on the 11q chromosome that contributes to neuroblastoma aggressiveness is crucial for understanding the pathways deregulated in these tumors. However, in spite of the intensive effort, only a few genes have been identified to date. In tumors with 11q LOH, the frequency of segmental aberrations is significantly higher than in MYCN amplified tumors . This fact was explained in part by the loss of the H2AFX gene, located in the 11q23.3 deleted region. This gene has been shown to play a role in genomic stability modification, and enhanced susceptibility to cancer in mice .
Other studies identified the cell adhesion molecule 1 ( CADM1 ), which transcribes a cellular adhesion protein involved in neural cell development, as a candidate tumor suppressor gene in the 11q23 deleted region. CADM1 was significantly downregulated in tumors with 11q LOH relative to 11q diploid tumors and significantly associated with the advanced stage of disease and poor survival . Overexpression of CADM1 revealed a significant inhibition of cell proliferation and colony forming ability in a panel of four different cell lines, demonstrating that CADM1 expression attenuates the malignant phenotype in cultured cells. No evidence for inactivating mutations of CADM1 or hypermethylation of its promoter was found, suggesting that other mechanisms such as haplosufficiency or posttranscriptional regulators of gene expression may be involved in the modulation of CADM1 expression .
p53/MDM2 Interaction
p53 is a tumor suppressor protein that plays a major role in the protection of genomic stability and prevention of tumor development by directly activating several genes, including miRNAs, which promote DNA repair, cell cycle arrest, and apoptosis. Inactivating mutations or deletions in the p53 gene are found in >50% of adult human cancers . However, in neuroblastoma, this gene is seldom mutated, occurring in <2% of cases at diagnosis, and ∼15% at relapse .
Unlike many adult malignancies, most neuroblastomas do initially respond to chemotherapy, and it is likely that the presence of functionally active p53 is at least partly involved in this initial chemosensitivity. However, over half of previously responsive tumors eventually relapses with the chemoresistant disease and there is evidence for p53 inactivation at this stage in some cases . p53 is a potent transcription factor that positively and negatively modulates a large number of genes involved in apoptosis, metabolism, epigenetics, and cell cycle regulation . p53 is usually expressed at low levels in the cell, where it has a short half-life of only 30 min . Neuroblastoma is a p53 wild-type malignancy at diagnosis and repression of p53 signaling plays an important role in its pathogenesis. Chemotherapy induces apoptosis and tumor regression primarily through activation of p53-mediated transcription. Most previous observations confirm that wild-type p53 alleles are present in the vast majority of cases of newly diagnosed neuroblastoma, but that p53/MDM2/ARF responses to chemotherapy are repressed, in part due to unscheduled inhibition of p53 by MDM2. This suggests that downregulation of the p53 axis may underlie the treatment resistance typically seen in high-risk neuroblastoma . The expression, function, and stabilization of p53 are governed by a complex network, which includes its regulators p14ARF and MDM2 .
Originally identified as an amplified gene located on a double minute chromosome in a transformed mouse cell line, MDM2 was later shown to have a critical role in the process of cellular transformation. Thus, deregulation of MDM2 gene expression likely contributes to the pathogenesis of a wide range of human tumors. Amplification and overexpression of MDM2 is found in about 10% of all human tumors. In gliomas, for example, MDM2 amplification identifies a subset of high-risk patients that do not have p53 mutations . In many soft tissue sarcomas, MDM2 amplification and overexpression correlates with poor prognosis.
Additionally, p53 activates the MDM2 gene that encodes a p53 inhibitor forming an autoregulatory feedback loop that tightly controls expression of both p53 and MDM2 . MDM2 helps to target p53 for degradation through its E3 ubiquitin ligase activity.
Recent literature demonstrates that p53 is generally functional, accumulates in the nucleus in response to DNA damage and is efficiently degraded by MDM2 in neuroblastoma. There are reports that p53 function may be compromised as a consequence of aberrant MDM2 expression levels in neuroblastoma. For instance, a study examining etoposide induced p53 activity in a neuroblastoma cell line suggested an important role for elevated MDM2 expression levels in the regulation of p53 translocation .
Also, elevated MDM2 expression and the accompanying loss of p53 function is associated with multidrug resistance in some neuroblastoma cell lines. The activity of MDM2 is critical in neuroblastoma, as recent work demonstrates that MDM2 ubiquitin ligase activity is rate-limiting for p53 degradation in neuroblastoma . Taken together, these data show that the MDM2/p53 interaction is intact in neuroblastoma and suggest that deficiencies in p53 functions may be a consequence of aberrant MDM2 expression.
MYC oncogenes activate both proliferative and apoptotic cellular pathways and, accordingly, inhibition of p53-mediated apoptosis is a prerequisite for MYC-driven tumorigenesis . A critical negative regulator of the p53 tumor suppressor, MDM2, has been recently characterized in neuroblastoma cell lines as a transcriptional target of MYCN. Targeted inhibition of MYCN results in reduced MDM2 expression levels, with concomitant stabilization of p53 and stimulation of apoptosis in MYCN amplified neuroblastoma cell lines . Moreover, MDM2 has a p53-independent role in the regulation of both MYCN mRNA stabilization and its translation, suggesting that MDM2-mediated MYCN expression is one mechanism associated with the growth of MYCN -associated neuroblastoma and disease progression . In MYCN amplified neuroblastoma raised MDM2 expression had a reinforcing effect on the progression of the disease . MYCN also transcriptionally upregulates p53 expression in neuroblastoma and may be an important mechanism by which MYCN induces apoptosis .
The above evidence, taken together, suggests that inactivation of p53 in neuroblastoma can cause the deregulation of both protein-coding genes and miRNAs, resulting in the deregulation of neuroblastoma related-pathways.
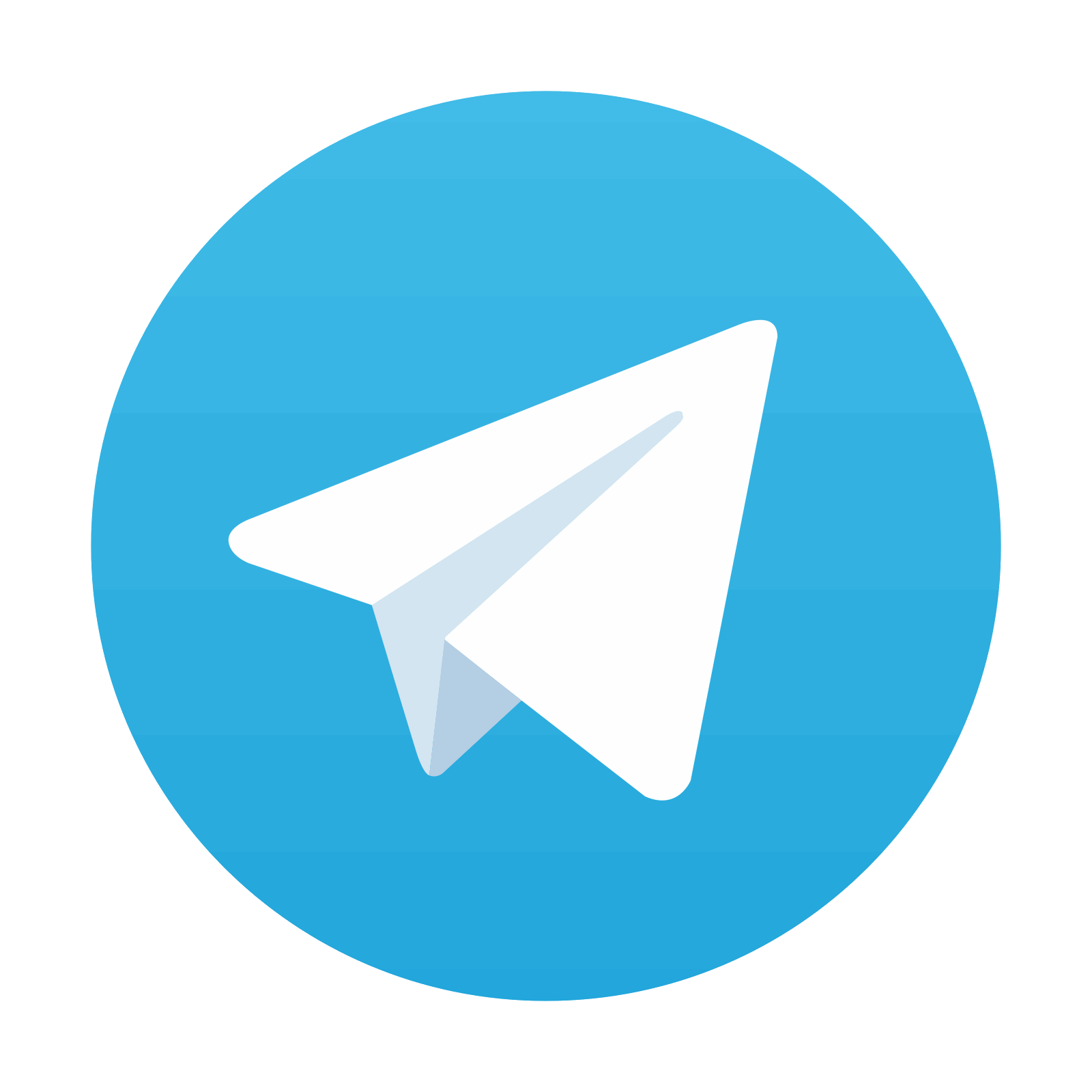
Stay updated, free articles. Join our Telegram channel

Full access? Get Clinical Tree
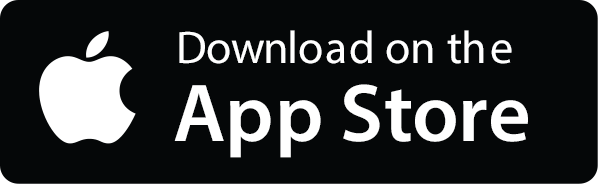
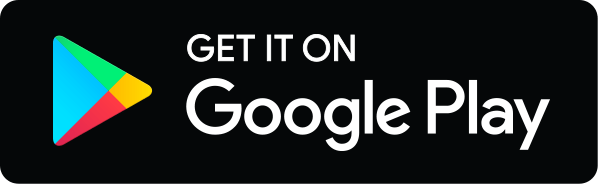