Fig. 13.1
CT scan sagittal view showing C6-C7 somatectomies and tri-cortical iliac strut graft and anterior plating (a). Postoperative posteroanterior X-ray view of the same patient (b)
Bone graft in anterior cervical surgery is used to achieve several goals. Structural grafts are used to reconstruct anterior column defects and restore the load-bearing capacity of the cervical spine. Bone grafts may be shaped to restore the normal lordotic posture of the cervical segment. Bone graft also performs a biologic role in promoting a bony fusion, which spans the spinal defect and achieves long-term stability. To be successful, bone grafts must be able to fulfill successfully the dual role of providing structural support and achieving a solid fusion [26].
Various materials have been used for interbody grafts in anterior cervical fusion [27]. To supplement the bone graft, a number of fusion devices have been developed over the past decades for stand-alone use or in conjunction with anterior or posterior instrumentation (Fig. 13.2a, b).
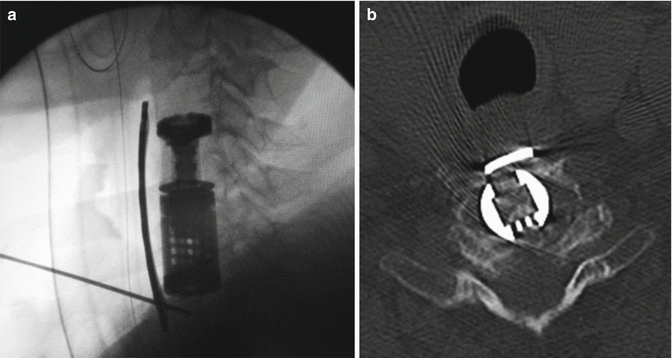
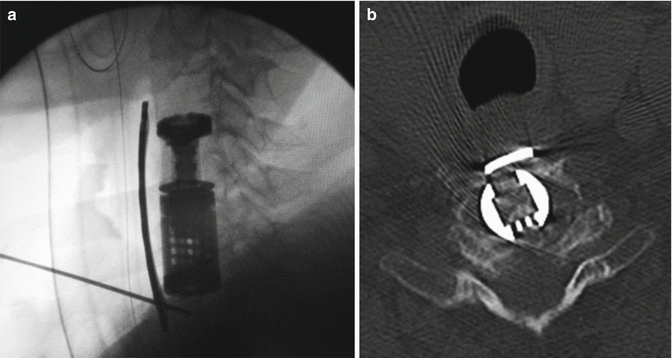
Fig. 13.2
Intraoperative X-ray image showing anterior plating after multilevel somatectomies and positioning of expandable vertebral bodies substitute (a). CT scan axial view showing mature bony trabeculae inside the expandable device of the same patient (b)
Cervical Spinal Devices
The objective of these spinal devices is to immobilize the unstable degenerated motion segment so that bony fusion can occur. Currently three types of spinal fusion devices are available: horizontal cylinders, vertical rings and open box cages (Fig. 13.3). We evaluated different fusion material devices: carbon fiber, polyetheretherketone (PEEK) and titanium [14, 28, 29].
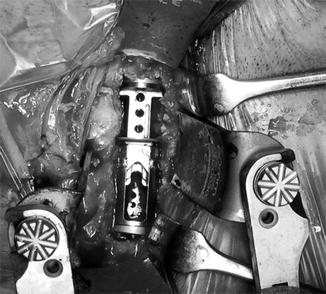
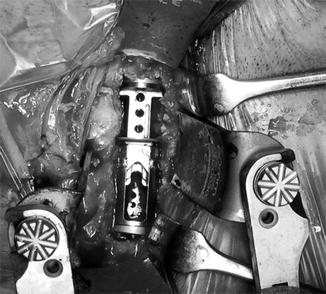
Fig. 13.3
Intraoperative picture showing expandable vertebral bodies substitute inserted into the corpectomy defects
Carbon fiber implants seem to present better osteointegration in comparison to metallic materials. The elasticity of carbon fiber implants , almost equal to that of cortical bone, reduces the so-called phenomenon of “stress protection .” In other words, it allows distribution of the physiologic load to the bone graft inside the implant, thus stimulating bone formation and improving the quality of fusion [30, 31] with less involvement of stress the adjacent vertebral segments. The advantages are obvious: radiolucency to assess fusion, a more compatible modulus of elasticity compared to titanium, and the ability for precision design and mass production. Potential drawbacks include fracture as a standalone device without anterior plating or a reactive inflammatory response to carbon [32]. Carbon debris does not seem to be problematic with the interbody space, as it is not exposed to a synovial joint. Carbon can fracture with loading, so it remains unclear as to the requirement of an additional anterior osteosynthetic support structure, such as a plate [33].
PEEK is a semi-crystalline polyaromatic linear polymer having a good combination of strength, stiffness, toughness and environmental resistance. PEEK cages are biologically inert and have high versatility and out-standing mechanical properties, including high strength in all directional planes, elasticity close to that of bone, impact and fatigue resistance [34]. In addition, PEEK is radiolucent, allowing a better radiographic assessment of bone fusion than titanium; the position of the cage can be assessed by radiopaque lines incorporated within the cage. Even if the data are still limited, PEEK cages seem to be MRI and CT compatible and cause no relevant artifacts that would reduce the clarity of imaging. Moreover, PEEK cages do not induce a corrosive reaction to contiguous vertebral bodies and show excellent properties at pull-out and mechanical compression tests [35]. Bone grows in response to applied stress and will be reabsorbed if a mechanical stimulus is lacking. Thus, stress shielding due to a high elastic mismatch between implant and bone can detrimentally influence bone growth, resulting in cortical thinning. In accordance with Williams et al. [36], these phenomena can be avoided by using cages made by pure PEEK possessing an elastic modulus close to that of bone grafting material.
The list of titanium benefits is lengthy. This makes it incredibly useful for a number of different industries, including the automotive, aerospace and architectural worlds. But because titanium resists corrosion, is biocompatible and has an innate ability to join with human bone , it has become a staple of the medical field, as well. From surgical titanium instruments to orthopedic rods, cages, mesh, pins and plates, medical titanium has truly become the fundamental material used in medicine. Titanium Ti 6Al–4 V and Ti 6Al–4 V ELI, alloys made of 6 % Aluminum and 4 % Vanadium, are the most common types of titanium used in medicine. Benefits of medical titanium are: strong, lightweight, corrosion resistant, cost-efficient, non-toxic, biocompatible (non-toxic and not rejected by the body), long-lasting, non-ferromagnetic, osseointegrated (the joining of bone with artificial implant), long range availability, flexibility and elasticity rivals that of human bone. Two of the greatest benefits of titanium are its high strength-to-weight ratio and its corrosion resistance. Couple this with its non-toxic state and its ability to fight all corrosion from bodily fluids and it’s no wonder titanium has become the metal of choice within the field of medicine.
Use of different devices (cages, mesh, plates) can help to get a primary mechanical stability of the segment operated but the fusion processes, which can’t ignore the stability worth the evolution in non-union, have as main actor bone graft whether autologous, eterologous, synthetic bone graft substitute or factor and cell–based approaches for bone graft substitutes.
Bone Grafts
Autograft
Cortico-cancellous bone harvested from the iliac crest is widely used for the cervical spine (Fig. 13.4). A systematic review of the literature reported autograft to have a mean arthrodesis rate of 77 % [26]. In one-level non-instrumented procedures, autograft fusion rates are a reported 83–99 % [37], but decreases with number of levels fused [38]. Autograft experiences relatively few incidences of graft complication, such as graft collapse or migration, and is biocompatible, poses no risk of disease transmission and is non-immunogenic [39]. For these reasons, autograft remains the standard of care for cervical spinal fusion. Unfortunately, the stipulation of a second surgical site not only increases operative time and blood loss but introduces significant donor site morbidity. Although the risk of harvest site morbidity has been suggested to be overstated, it is generally accepted within the literature to be of significant concern [40]. A retrospective study of one-level anterior cervical fusion found 26.1 % of patients suffered persistent pain and 15.7 % experienced numbness at the harvest site [41].
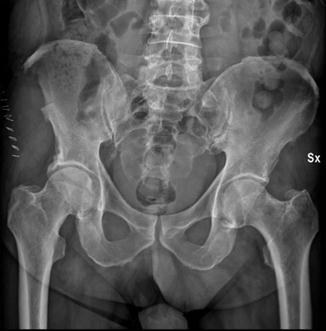
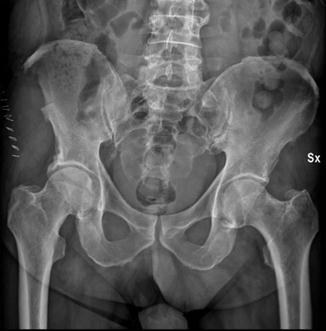
Fig. 13.4
Posteroanterior X-ray of pelvis after the harvest of tri-cortical iliac strut graft on the right side
Functional assessment revealed impairment in ambulation (12.7 %) and other daily activities. Many other complications have been observed including infection, hematoma, bruising, pelvic fracture, periotoneal perforation, hernia, gait, ureteral injury, reoperation and poor cosmesis [42, 43]. Rawlinson reported 31 % of patients felt donor site pain caused them to remain in hospital longer than if they had not had that procedure [44]. Finally, there are inherent limitations with supply and occasionally, autograft quality. Some authors have investigated the harvest of autograft from alternative locations, such as the fibula [45], cervical vertebrae [46], clavicle [47], and the manubrium [48], so as to retain the advantages of autograft whilst circumventing its associated morbidity, to varying success. Others have explored the effectiveness of iliac crest reconstruction using synthetic materials in alleviating postoperative pain, with mixed results [49, 50].
Allograft
Allograft is the most commonly used non-autogenous grafting material in spinal surgery, and 35 % of all bone transplantations involve the use of human allograft tissues [51]. Mineralized allograft is primarily osteoconductive, with weak osteoinductive capacity and no osteogenic potential because graft cells do not survive processing and transplantation. Allograft used for orthopedic applications is fresh frozen, freeze-dried, or demineralized. The method of preparation has significant effects on graft strength, immunogenicity, capacity for incorporation, and potential for disease transmission. Fresh-frozen allografts retain much of their original mechanical strength, while freeze-drying can reduce graft strength up to 50 % [52]. The freezing process also reduces immunogenicity of allografts [53]. The effect of immunogenicity in compromising graft incorporation may be significant [54–56]. Transmission of disease from donor to recipient is a problem with human allografts. The principle pathogens involved are human immunodeficiency virus (HIV) and hepatitis viruses B and C. The risk of disease transmission is determined by the rigor of screening procedures for donors and tissue, and the only cases of disease transmission in musculoskeletal allografts from the method of graft preparation to date have involved frozen, unprocessed grafts [57]. Tissue processing techniques include high pressure lavage to clear out marrow elements and donor cells and chemical treatments to eliminate viruses and reduce immunogenicity of the graft. The combination of donor screening, tissue testing, and tissue processing reduces the risk of viral transmission to less than one event per million grafts [58]. Allograft is available in many preparations. However, the majority are composed of primarily cancellous or cortical bone. Cortical allografts provide significant mechanical stability and structural support, while cancellous bone lends little mechanical stabilization on implantation but has a faster rate of incorporation. Cancellous allograft and particulate allograft preparations (cancellous or cortical) incorporate with new bone forming on the surfaces of trabeculae, with a large surface area available for new bone formation [54, 59]. In contrast, cortical incorporation occurs slowly via a process of periosteal new bone formation around the allograft as an external callus derived from the host bone [60]. Particulate and structural grafts demonstrate significant differences in the histology of incorporation. Particulate grafts demonstrate more rapid and complete revascularization than structural grafts. Particulate bone remodels completely with time, while cortical bone remains a mixture of necrotic and viable bone. The process of creeping substitution is also differs significantly between these forms of allograft, with new bone formation occurring appositionally followed by resorption in cancellous bone, which process is reversed in cortical allografts [61]. These differences in biologic capacity between graft types lead to significant differences in optimal clinical applications. The use of bone allografts in the spine has been reviewed previously by the senior author [62]. Structural cortical allografts are most useful in interbody arthrodesis of the lumbar and cervical spine, with low rates of graft tricortical allograft may be as effective as iliac crest in promoting anterior arthrodesis of the spine [63]. Crushed cortical or cancellous allograft may be useful as an autograft extender in posterior spinal fusion. In thoracolumbar deformity , cancellous allograft with instrumentation may give satisfactory results in the pediatric population but yields inferior results in adults. The conclusion from the senior author’s experience is that successful use of allograft bone in the spine is dependent on the type of allograft bone used, the anatomic site of fusion, and patient age. A review of other clinical applications of allograft compared with autogenous bone in spinal surgery is useful. In cervical spine, the use of allograft vs autograft has been debated since the first anterior discectomies and interbody fusions. Smith and Robinson used autogenous iliac crest graft and reported radiographic union in 18/21 patients [64]. Concurrently, Cloward reported resorption of only 3/46 grafts using his dowel technique with fresh-frozen allograft [4]. More recent reviews demonstrated similar fusion rates using autogenous and allogenous grafts in single level cervical surgery but significant differences in multilevel cervical fusions [65, 66]. In posterolateral arthrodesis of the lumbar spine, differences in function between allograft and autograft are more significant. In a prospective comparison of autograft and allograft preparations in posterolateral arthrodesis of the spine, differences in fusion mass were radiographically clearly apparent, reliable arthrodesis being achieved with autograft, followed by mixed autograft and allograft, and frozen allograft, and the least reliable graft material was freeze-dried allograft [67]. Similarly, a prospective evaluation of mineralized and demineralized allograft mixed with autogenous bone radiographically demonstrated fusion inferior to that from posterolateral arthrodesis with iliac autograft [68].
Synthetic Bone Graft Substitutes
There are four characteristics that an ideal bone graft material should exhibit which include [69]:
I.
osteointegration, the ability to chemically bond to the surface of bone without an intervening layer of fibrous tissue;
II.
osteoconduction, the ability to support the growth of bone over its surface.;
III.
osteoinduction, the ability to induce differentiation of pluripotential stem cells from surrounding tissue to an osteoblastic phenotype; and
IV.
osteogenesis, the formation of new bone by osteoblastic cells present within the graft material.
Only autogenous bone graft satisfies all of these requirements. Allograft is osteointegrative and osteoconductive and may exhibit osteoinductive potential, but it is not osteogenic because it contains no live cellular component. Synthetic bone graft substitutes currently possess only osteointegrative and osteoconductive properties . Ideally synthetic bone graft substitutes should be biocompatible, show minimal fibrotic reaction, undergo remodelling and support new bone formation. From a mechanical point of view synthetic bone graft substitutes should have a similar strength to that of the cortical/cancellous bone being replaced. This needs to be matched with a similar modulus of elasticity to that of bone in an attempt to prevent stress shielding as well as maintaining adequate toughness to prevent fatigue fracture under cyclic loading. Synthetic materials that demonstrate some of these properties are composed of either calcium, silicon or aluminium [70].
Calcium Phosphate
The calcium phosphate family of synthetic bone grafts has both osteointegrative and osteoconductive properties. Osteointegration results from the formation of a layer of HA shortly after implantation. The Ca2+ and PO4 2− ions required to establish this layer are derived from the implant and surrounding bone. The pathways of both Ca2+ and PO4 2− ions have been traced in serum and urine without any significant elevation in serum levels from which it can be concluded they are handled as part of the normal body ion pool. They have an excellent record of biocompatibility with no reports of systemic toxicity or foreign body reactions [71].
Beta Tricalcium Phosphate
Beta tricalcium phosphate (βTCP ) was one of the earliest calcium phosphate compounds to be used as a bone graft substitute. In 1920 Albee and Morrison reported that the rate of bone union was increased when βTCP was injected into the gap of a segmental bone defect [72]. Beta tricalcium phosphate is available in porous or solid form as either granules or blocks. Structurally porous βTCP has a compressive strength and tensile strength similar to cancellous bone [73]. Like other calcium phosphate preparations it has been found to be brittle and weak under tension and shear, but resistant to compressive loads [74].
Typically it has been used in its granular porous form. Porous granules tend to migrate less than solid granules due to earlier fixation by fibrovascular ingrowth [75]. Beta tricalcium phosphate undergoes reabsorption via dissolution and fragmentation over a 6–18-month period. Unfortunately the replacement of βTCP by bone does not occur in an equitable way. That is, there is always less bone volume produced than the volume of βTCP reabsorbed [76]. For this reason the clinical use of βTCP has been as an adjunctive with other less reabsorbable bone graft substitutes or as an expander for autogenous bone graft.
Hydroxyapatite (HA)
The chemico-physical characteristics of the HA graft allow to induce a rapid and complete interbody fusion but also restore the physiological lordosis and maintain the intervertebral and foraminal height [77]. The HA ceramics are composed of hydroxylized calcium phosphate and are chemically identical to natural HA of bone. The process of mixing these materials leads to porous ceramics with high osteoconductive properties. The graft can be invaded by newly formed bone that grows directly into the pores [78]. Unlike other synthetic grafts, however, there is no interposition of fibrous tissue between implant and bone [73]. In experimental studies and, in particular, those in which electron microscopy observations are made, the authors have demonstrated the bioactive properties of HA grafts and their apparent ability to be directly bonded to bone, reproducing the natural bone-cementing mechanism [79].
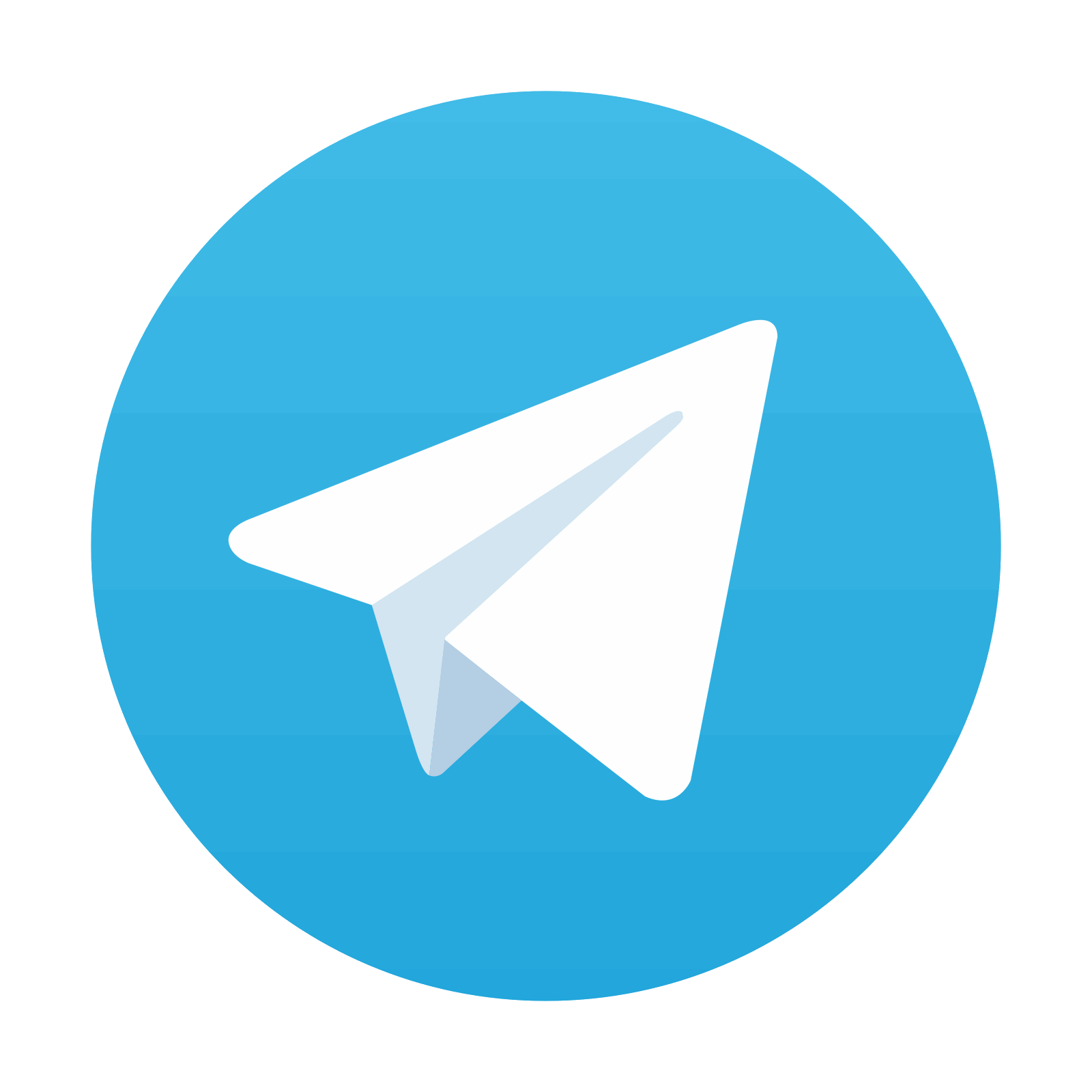
Stay updated, free articles. Join our Telegram channel

Full access? Get Clinical Tree
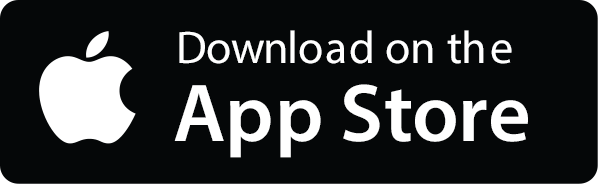
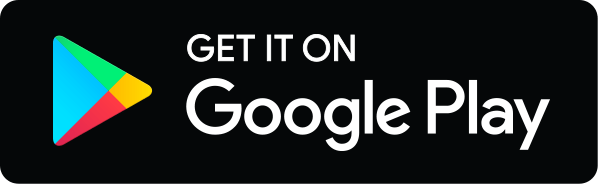