NC-ICA, MCA, AC/COM (%)
VB, PC/COM (%)
Cavernous carotid artery (%)
<7 mm group 1
0
2.5
0
<7 mm group 2
1.5
3.4
0
7–12 mm
2.6
14.5
0
13–24 mm
14.5
18.4
3
>24 mm
40
50
6.4
Pathogenesis
It was previously thought that aneurysms were the result of impaired smooth muscle and endothelial migration during embryonic development. It is now widely accepted that a cascade of inflammatory events result in both aneurysm formation and rupture [24]. It is not a coincidence that certain risk factors for atherosclerotic disease, namely, hypertension, overlap those of aneurysmal development as vascular injury, especially to the internal elastic lamina, occurs in both. Macrophages respond to this injury with the secretion of inflammatory mediators including interleukins and proteases, i.e., MMP. Macrophages also create a highly oxidative state injuring and killing the smooth muscle cells within the vicinity. There is ultimately loss of the structural integrity of the vessel wall with apoptosis of smooth muscle and endothelial cells, the breakdown of collagenous extracellular matrix (ECM), and disorganized and dysregulated vascular remodeling [25, 26]. This has been corroborated by evidence suggesting that macrophage-depleted mice have lower incidence of aneurysms. Moreover, mice with knockout genes for monocyte chemotactic protein-1 also have lower incidences of aneurysm formation [25].
The process is accentuated at artery bifurcations that are common locations for aneurysms. The multidirectional shear stress and turbulent flow applied to vessel walls result in nonuniform remodeling. Moreover, areas of persistently increased stress continue to undergo chronic disorganized remodeling. This results in segments of weaker structural framework between intimal pads—areas of thickened and inelastic tissue—predisposing aneurysms for rupture [26]. This predisposition is especially notable in cerebral arteries as these vessels lack an external elastic lamina and have only a thin internal lamina.
Pathologic evaluation of unruptured and ruptured aneurysms indicates a continuum of inflammatory changes from disorganized replacement of smooth muscle cells to unstable plaques [27]. Turbulent flow ruptures these plaques, triggering further inflammatory infiltration. This accounts for the consistent presence of leukocytes present in the peri-aneurysmal region. The end result is very fragile vascular walls prone to rupture. The lack of structural integrity is underscored by the leaky connections between smooth muscle and endothelial cells, where intercalated red blood cells are frequently identified [27]. The lack of adhesion results also acts as a potent apoptotic signal to the few remaining smooth muscle cells [28]. Ruptured aneurysmal walls frequently demonstrate the absence of smooth muscle cells and collagen type IV, with membranous hyaline replacement. Smooth muscle cells provide the plasticity and malleability of blood vessels to withstand changes in hemodynamic pressures. The loss of smooth muscle cells results in poor compliance and provides an explanation for the increased pulsatility of ruptured aneurysms [29].
Clinical Presentation
When a patient presents to the emergency room with the “worst headache ever,” a dilated pupil, and a focal neurologic examination, the diagnosis of subarachnoid hemorrhage is obvious. However, such a fulminant presentation does not always occur. Instead, patients frequently present with only a headache that may even be transient. This headache is unusually severe. However, this sensitive symptom has a very low specificity for aneurysmal rupture, accounting for 1 % of all nontraumatic headache visits to the emergency room [30–33]. Headache features that are more suggestive of aSAH include acute onset of a very severe headache that is different in quality from the patient’s usual headache pattern. An atypical and severe headache occurs in more than 75 % of patients [3, 34]. The positive predictive value increases when nausea, vomiting, and/or meningismus is also present [33, 35–37]. Their presence is noted in 61–77 % of patients [3, 34]. Nausea and vomiting result from elevated intracranial pressures; the degraded blood products also stimulate the area of postrema.
Sentinel symptoms, such as headaches, occurring days to weeks prior to fulminant aneurysm rupture, are present in 10–40 % of patients [38–40]. The onset of headache during exertion or a Valsalva maneuver may also suggest aSAH. Physical activity, defecation/voiding, and sexual intercourse may precipitate rupture by causing an abrupt rise in intracranial pressure [3, 41, 42]. Although helpful when present, the onset of symptoms during exertional activity occurs in less than half of patients [3].
Physical exam findings including neurologic deficits may help clinicians hone in on patients with the highest likelihood of aSAH. Nuchal rigidity results from intrathecal inflammation caused by degraded blood products in the subarachnoid space. Elevated systolic blood pressure greater than 200 mmHg is present in 32 % of patients [3]. This is likely a manifestation of the hyperautonomic state that immediately precedes rupture. The presence of either nuchal rigidity or hypertension may occur in roughly 40 % of patients [3].
An altered sensorium occurs in up to 40 % of patients [3, 34]. This results from impaired cerebral perfusion during periods of elevated intracranial pressure (ICP). Brief losses of consciousness, coma, and confusion or lethargy occur in 36 %, 17 %, and 28 % of patients, respectively [3].
Focal neurologic deficits are present in 10–13 % of patients [3, 34]. These deficits, when present, may be helpful in identifying the aneurysm’s location. For example, patients with diplopia and an unreactive large pupil are frequently due to ipsilateral posterior communicating and less commonly superior cerebellar artery aneurysms. Similarly, patients with facial-brachial weakness are likely to have aneurysms around the contralateral middle cerebral artery (MCA). Nonmotor signs including aphasia and visual spatial deficits may localize to the dominant and nondominant MCAs, respectively.
Diagnosis
Despite the poor specificity of a severe headache as the presenting symptom of aSAH, cost-benefit analyses favor early and prompt recognition with a diagnostic evaluation as this results in improved outcomes [33, 43]. This is a testament to the potentially devastating morbidity and mortality of delayed diagnosis of aSAH.
Once the decision to rule out aSAH is made, patients should undergo a complete evaluation. This begins with an emergent non-contrast computerized tomography (CT) of the head which has a sensitivity approaching 100 % if performed within the first 6 h of headache/symptom onset [33, 44]. Its sensitivity drops dramatically with time to 85, 50, and 30 % at 5, 7, and 14 days, respectively [33, 45, 46]. Conversely, the yield of lumbar puncture increases with time. In fact, the negative predictive value for SAH is 100 % when a lumbar puncture is performed from 12 h of symptom onset to 14 days [47, 48]. The diagnostic finding of xanthochromia, yellowish-colored cerebrospinal fluid (CSF), results from the oxidation of lysed erythrocyte hemoglobin. This oxidative reaction takes 12 h—accounting for its high sensitivity beyond this time period [33]. The absence of xanthochromia after the first 12 h excludes SAH [48]. CT of the head followed by spectrophotometric analyses of CSF is the gold standard for diagnosing SAH [48].
Lumbar punctures, however, are not very specific and may yield false-positive results for several reasons. Namely, CSF from a traumatic tap with red blood cells >10,000 may appear xanthochromic. The common teaching that a sequential decline in the number of RBCs from tube 1 to tube 4 as being consistent with a traumatic tap has a sufficient negative predictive value to exclude aSAH is incorrect. Heasley et al. reported that 25 % of patients may demonstrate this clearing despite having aSAH [49]. Moreover, if there is a delay in the spinning and analysis of CSF, oxidation of RBCs may occur in vitro resulting in xanthochromia [50]. Hospitals/institutions frequently rely upon visual inspection alone for reporting CSF xanthochromia as spectrophotometers are uncommon devices. This results in significant interobserver variability and misdiagnosis. Visual inspection of CSF for detection of xanthochromia cannot reliably exclude SAH [51]. Other imaging modalities aside from plain CT scan may also be used to evaluate patients with possible SAH. Magnetic resonance imaging (MRI) scans have received a great deal of attention. MRI has been studied for its utility in the hyperacute setting. Wiesmann et al. [52] found that in a cohort of 13 patients who underwent an MRI within 12 h of headache, SAH was detected in all cases with either FLAIR or proton density sequences. Similarly, in the acute time period (within the first 96 h), gradient echo and FLAIR series have been found to have sensitivities of 94 and 100 % [53, 54].
MRI is also very sensitive at detecting subacute SAH. In fact, the sensitivity of gradient echo MRI sequences at days 4–6 approaches 100 %, while that of CT is 45.5 % [53, 54]. This may preclude the need for lumbar puncture in the subacute setting.
At this time MRI is impractical as the study of choice for patients presenting with possible SAH as it takes significantly longer to perform and is poorly tolerated by claustrophobic patients. However, MRI can be utilized in cases where the initial workup is nondiagnostic or equivocal. For example, patients with erythrocytes in the CSF due to a traumatic tap who have a negative head CT could avoid an invasive cerebral angiogram if MRI demonstrates no abnormal signal on GRE and FLAIR. Furthermore, an MRA may be performed at the same time to evaluate for any aneurysms >5 mm as these have higher likelihood of rupture [55]. A normal MRA would provide further evidence to exclude SAH.
Angiographic Detection and Evaluation
Once subarachnoid hemorrhage has been diagnosed, the search for potential causes is undertaken. Typically this includes vascular imaging to assess for ruptured cerebral aneurysms that account for 85 % of nontraumatic SAH. Digital subtraction angiography (DSA) remains the gold standard for this as the accuracy and depth of diagnostic information it provides remain unsurpassed [56]. This minimally invasive procedure has a low but definite risk of complications including stroke, femoral artery injury, and acute kidney injury related to iodinated contrast. The risk of complications leading to permanent neurologic disability is typically cited between 0.06 and 0.33 % [56–58].
Interestingly, cerebral angiograms may fail to identify ruptured aneurysms in the first 14 days. Thrombus at the site of rupture or cerebral vasospasm may prevent their detection during the acute setting. Thus, an angiogram must be repeated in 3–4 weeks, especially in patients with an aneurysmal pattern of hemorrhage, when these have resolved. Repeat cerebral angiogram may detect 17 % of aneurysms that were undetected on the first angiogram [59].
Recently, noninvasive imaging modalities have been utilized in lieu of cerebral angiography. These include MRA and CTA. MRA allows assessment of cerebral vasculature without the risks of radiation and iodinated dye. However, the resolution is suboptimal at visualizing small aneurysms (<5 mm) [55]. Moreover, it fails to provide sufficient anatomical detail for surgical intervention. It has a limited role in the acute setting and serves better as a screening modality in patients at higher risk for aneurysms [60].
Computerized tomography angiography (CTA) has been more successful than MRA at not only detecting aneurysms but also providing surgeons with enough anatomical detail to proceed with definitive therapy without the need for DSA. While some studies have reported no discrepancies between CTA and DSA for surgical planning, others have provided examples illustrating its limitations. Anderson et al. [61] reported that preoperative CTA provided sufficient information to directly proceed to surgery in only 48 % of aneurysms—predominately those coming off the middle cerebral artery. While the anatomic details including size, shape, neck characteristics, and orientation were sufficient in these cases, bony artifact limited visualization of the posterior circulation. Anderson’s study also demonstrated CTA’s poor sensitivity to detect small (<4 mm) aneurysms as twenty-four such aneurysms (16 %) were missed by CTA. One patient underwent surgery for an asymptomatic aneurysm as the ruptured aneurysm (3 mm) was not visualized on CTA.
At our institution, CTA of the head/neck is performed when aSAH is suspected on the basis of CT scan or lumbar puncture. This provides two types of important information: It allows for early noninvasive detection of cerebral aneurysms with a sensitivity approaching 99.2 % and a specificity of 100 % [62]. If the culprit aneurysm is identified and its anatomy and configuration can be adequately discerned then these patients are taken to the angiography suite or operating room for treatment under general anesthesia. Secondly, the CTA of the neck offers the interventionists an opportunity to appreciate the proximal tortuosity and prepare for potential complications or difficulties related to vascular access. If, however, no aneurysm is detected on CTA or if the anatomy is not well defined, the patient is taken to angiography suite for a diagnostic angiogram under conscious sedation. If an aneurysm is then identified, the patient is subsequently intubated and the aneurysm subsequently treated if technically feasible. Thus, the benefits of this rapidly obtainable, noninvasive modality cannot be ignored. CTA may become the imaging modality of choice for SAH with DSA being performed in equivocal cases and in cases where CTA fails to identify a culpable source [63].
Acute Management
The patient’s neurologic status at time of presentation offers the best prognostic information in aSAH patients. This is not only important for these patients’ families but also in determining aggressiveness of care and therapeutic measures best fit for patients. The Hunt and Hess classification schema (Table 11.2) of aSAH patients provides a quick and accurate method for predicting outcome of patients with ruptured aneurysms [64, 65]. This 5-point scale has been validated for its reproducibility.
Table 11.2
Classification schema of aSAH patients
Grade | Description | Mortality (%) |
---|---|---|
I | Headache, no neurologic impairment | 30 |
II | Nuchal rigidity, drowsiness, mild impairment (i.e., forgetfulness), moderate to severe headache, cranial neuropathy | 40 |
III | Lethargy or confusion, mild impairment of power/tone/sensation | 50 |
IV | Unconsciousness with marked changes in tone and power | 80 |
V | Unresponsive | 90 |
The World Federation Neurosurgery (WFNS) scale is another scale that may be employed to help prognosticate. This scale utilizes both the Glasgow Coma Scale (GCS) and the presence of focal neurologic deficits, i.e., hemiparesis and/or aphasia, to predict longer-term outcomes. While utilized by some centers, its interobserver variability has prevented its wider acceptance. The major issue being the characterization of focal neurologic deficits; for example, some clinicians may score the presence of pronator drift with a GCS of 7–12 as grade 3, while others may fail to characterize the pronator drift as a major focal deficit and thus score the patient as grade 2.
Stabilization (See Chap. 3)
aSAH patients should be admitted to an intensive care unit to closely monitor for any deterioration (AHA). A majority of complications occur within the acute and subacute setting.
Airway management
In patients with higher-grade SAH, rapid sequence intubation and mechanical ventilation may be necessary [66]. Indications for this include aspiration, acute cardiopulmonary failure, and impaired mental status (GCS of 8 or less). Short-acting anesthetic agents should be utilized when possible to allow for frequent accurate neurologic checks. Thiopental and etomidate are preferred agents. Lidocaine and fentanyl may also be utilized for their potential to lower intracranial pressure (ICP). In very agitated patients, early intubation may be necessary to avoid precipitous rises in ICP which may increase the risk of rebleeding [67].
Blood pressure
Blood pressure is another parameter that needs to be closely monitored. The range is best determined by the physicians involved, as it needs to be tailored to patients’ medical problems and other comorbid conditions. As a rule of thumb though, normotension with systolic pressures less than 140 mmHg should be maintained prior to securing the aneurysm. Systolic pressures up to 200 mmHg may be tolerated, if necessary, after the aneurysm has been definitively treated [68].
Analgesia
Pain control should be managed with reversible short-acting agents. This would allow for frequent neurologic evaluations. Acetaminophen is typically favored. However, if this fails to provide adequate pain control, morphine or codeine may be administered [68].
Hydrocephalus
Obstructive hydrocephalus usually occurs within the first week. This risk significantly decreases with time. In fact, the risk may be as low as 3 % after day 3 of SAH if no evidence of ventricular enlargement was present on admission [69].
Obstructive hydrocephalus may occur in approximately 19 % of nontraumatic SAH patients, usually presenting with an acute deterioration of mental status [70]. Thus, patients who deteriorate in the hospital undergo emergent CT of the head to evaluate for hydrocephalus. If new/worsening hydrocephalus is present, an external shunt is placed.
The degree of hydrocephalus on CT has a poor clinical correlation with mental status and neurologic dysfunction. Thus, when incidental hydrocephalus is found in alert and awake patients, no interventions are undertaken. A converse situation frequently arises when patients present to the hospital with a high-grade aSAH with radiographic evidence of hydrocephalus. It is often unclear if the mental status changes are a direct result of the aSAH or due to the secondary hydrocephalus. Some authors recommend a period of monitoring for 24 h. If the patient does not improve, an external ventricular device (EVD) is placed; however, if the patient’s clinical status deteriorates, this is performed earlier. This delay in drain placement may not affect mortality [69]. However in our institution we favor early placement of a ventricular drain.
There is a theoretical concern of increased risk of rebleeding prior to securing the aneurysm in patients with early EVD placement. This is believed to be due to the rapid decrease in the intracranial pressure (ICP) immediately after the implantation of the EVD. This decline in ICP may result in a significant rise in the transmural pressure transmitted to the cerebral vasculature including the weak site of aneurysm rupture. This concern has not materialized in the scientific studies, however. The Mayo Clinic in 2002 found a trend toward less rebleeding in patients with preoperative ventriculostomy [71].
Vasospasm
Vasospasm accounts for approximately 20 % of the morbidity and mortality associated with subarachnoid hemorrhage [70]. There is evidence that vasospasm may occur within the first 48 h of rupture [72]. Angiographic evidence of vasospasm occurs in 70 % of aSAH, and clinical deterioration is seen in as many as two-thirds of these patients [73]. Signs and symptoms suggestive of this include decreased mentation and fluctuating neurologic deficits.
The pathophysiology of vasospasm is unclear; however, the presence of oxidized hemoglobin, oxyhemoglobin, is necessary [74, 75]. Free radicals formed during this oxidative process damage vascular membranes—eliciting an inflammatory response. This results in both decreased nitric oxide (NO) production and decreased sensitivity of its receptors. Potent vasoconstrictors including prostacyclins and cytokines such as endothelin-1 are also secreted [76]. These synergistically contribute to vasospasm [77, 78]. Histological changes also result from the inflammatory response. These include degeneration within the media and elastica layers, concentric thickening of the intima, and an abundance of myofibroblasts and type V collagen [79, 80].
Certain features are predictive of vasospasm, especially the amount of subarachnoid hemorrhage initially present on a CT scan of the head. Patients with more subarachnoid blood and those with thicker and larger cisternal clots tend to have more severe vasospasm than patients in whom the blood is minimal or distributed diffusely [81, 82]. Moreover, patients under the age of 20 and those who rebleed carry a higher risk for vasospasm [82]. The modified Fischer scale (Table 11.3) incorporates many of these imaging findings to predict prognosis and risk of DID. This scale uses a scoring system where each unit increase is associated with an incremental risk for vasospasm and stroke [83].
Vasospasm typically occurs during days 4–14 and usually peaks at days 7–8 [81]. It may result in permanent neurologic deficits and death due to delayed ischemic deterioration (DID). Patients are monitored very closely during this period as early detection and intervention are crucial for a favorable prognosis [66].
Angiography remains the gold standard in diagnosing vasospasm. However, its invasive nature prevents its daily use for vasospasm monitoring. Transcranial Doppler ultrasound (TCD) is frequently used to monitor for evidence of early vasospasm for this noninvasive bedside test can be performed without potential harm to patients. TCDs have good sensitivity and specificity for clinically significant vasospasm—equal to that of cerebral angiography in the detection of symptomatic spasm [84]. TCDs measure basal cerebral artery flow velocities as surrogates for vasospasm. This correlation, derived from Poiseuille’s equation, notes an exponential indirect relationship between vessel diameter and flow velocity. Frequent use during the peak period of vasospasm allows for monitoring of flow velocities and their trends (which is more sensitive for critical vasospasm) [85]. In so doing, treatment of vasospasm can be promptly initiated prior to clinical deterioration. This is important as TCD evidence of vasospasm may precede clinical deterioration by 24 h [84].
Effective prophylactic and therapeutic measures to treat vasospasm are very limited. In fact, prophylactic administration of oral nimodipine for 21 days remains the only treatment showing significant reduction in DID and poor outcome in a randomized double-blinded placebo-controlled study. Nimodipine reduces the risk of cerebral infarction and poor outcome at 3 months by 33 % and 40 %, respectively [86]. In addition to its potential for reducing cerebral vasospasm, nimodipine is known to inhibit other cellular-mediated processes that require calcium as cofactors, i.e., apoptosis. Administration of nimodipine is a class A recommendation by the American Heart Association (AHA) for aSAH [66].
Triple-H therapy, which refers to combination of hypervolemia, hemodilution, and hypertension, has traditionally been utilized in the setting of vasospasm; however, only hypertension is now utilized. In fact, a recent meta-analysis of these treatments found no evidence to support the use of hemodilution [87]. A few studies demonstrating the efficacy of hypertension and triple H for therapeutic and prophylactic use in SAH patients, respectively, were cited. However, these studies used cerebral perfusion rather than clinical outcomes as primary endpoints [88, 89].
Early studies also seemed promising for magnesium. Magnesium may prevent vasospasm by competitively inhibiting calcium channels in smooth muscle cells. Moreover, magnesium may have neuroprotective effects. Recent meta-analysis failed to demonstrate a benefit from magnesium infusion in reducing DCI or in improving neurologic outcomes [90].
Endovascular treatment is effective in preventing DID in patients who have failed medical management of vasospasm. It is also beneficial in patients who are otherwise unable to tolerate these therapies. For example, patients with systolic dysfunction or those with neurogenic pulmonary edema would be precluded from hypervolemic therapy. In such cases, transluminal balloon angioplasty and intra-arterial infusion of vasodilators including nicardipine, verapamil, and verapamil and nicardipine have been found to be very effective. The complication rates of these procedures are low, and the reported outcomes have been promising, with 61 % of patients demonstrating a good outcome at follow-up [91]. Balloon angioplasty tends to have a longer-lasting and more robust effect as it may lead to permanent endothelial changes but may be associated with up to a 5 % risk of vessel rupture.
Table 11.3
Modified Fischer scale
Score | Description | Vasospasm (%) | Delayed infarction/poor outcome (%) |
---|---|---|---|
0 | No SAH or IVH | 0 | |
1 | Focal or diffuse, thin SAH, no IVH | 6 | |
2 | Focal or diffuse, thin SAH, IVH | ||
3 | Focal or diffuse, thick SAH, no IVH | ||
4 | Focal or diffuse, thick SAH, IVH |
Complications
Complications related to endovascular coiling in aSAH are mainly those related to rebleeding and/or ischemia related to the catheter or from thromboembolism. The former necessitates reversal of any anticoagulation, such as immediate protamine administration. The latter may necessitate permissive hypertension to maintain cerebral perfusion or thrombectomy in cases of thromboembolism [92].
The results of International Subarachnoid Aneurysm Trial (ISAT), a multicenter randomly controlled trial, suggested that coiling was superior to clipping in reducing mortality and short-term morbidity. Both treatment modalities had their benefits and risks. Coiling was associated with a lower risk of dependence and mortality at 1 year. This advantage has carried out to at least 7 years. The risk of epilepsy and vasospasm after coiling was significantly lower than in patients who had undergone clipping [93–96].
These results have been reproduced in subsequent studies. In Barrow Ruptured Aneurysm Trial (BRAT), the safety and efficacy comparing surgical clipping with endovascular coiling of acutely ruptured aneurysms found that at 1 year posttreatment, coil embolization resulted in significantly fewer poor outcomes [97]. At 3 years posttreatment, this trend favoring coil embolization, however, was not statistically significant [98].
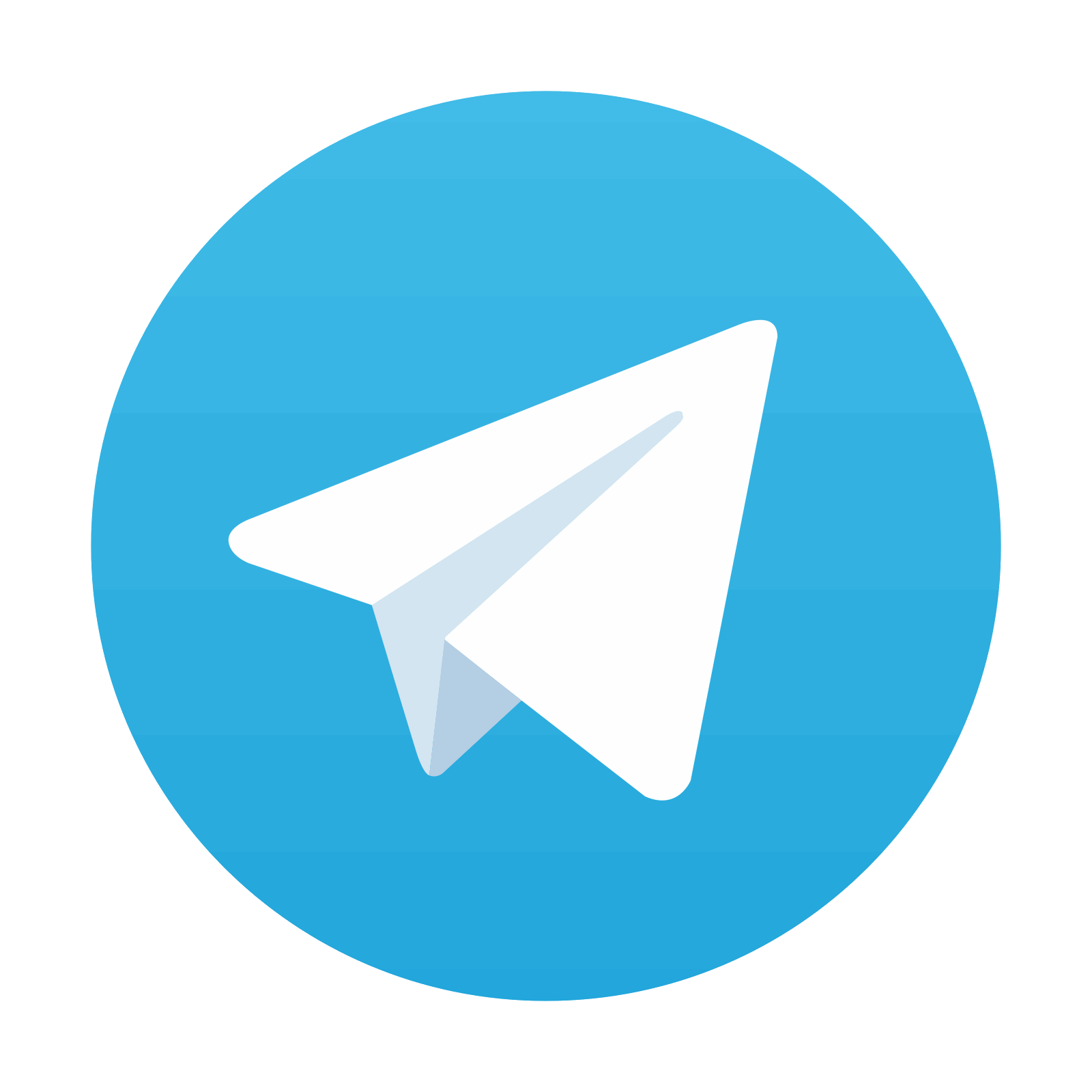
Stay updated, free articles. Join our Telegram channel

Full access? Get Clinical Tree
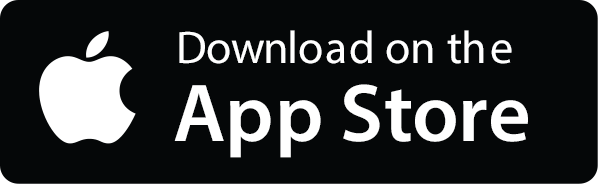
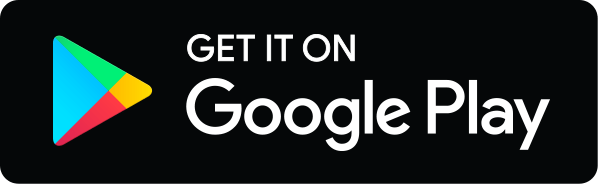