Neuromodulation is an essential part of functional neurosurgery and an important therapeutic modality for many patients suffering from medically refractory disorders. Deep brain stimulation (DBS), motor cortex stimulation (MCS), vagal nerve stimulation (VNS), spinal cord stimulation (SCS), and peripheral nerve stimulation (PNS) have become commonplace, as well as other devices such as phrenic nerve stimulators that are used in smaller numbers. As a result, the safety implications of implanting these devices must be well known to the neurosurgeon and communicated clearly to the patient and their caregivers. Every device has its own specific design characteristics and internal pulse generator (IPG) components and, given the variety of systems and manufacturers that exist, this chapter will focus on general safety concerns and limitations for neuromodulation devices as a whole. Where appropriate, circumstances specific to a particular device will be discussed. Finally, the field of neuromodulation is a rapidly evolving landscape with new innovations occurring at an ever-increasing pace. New devices as well as modifications to existing devices are constantly under development, so one must keep this in mind when considering the safety issues discussed here.
Patient activity and environmental concerns
Postoperative activity
Patient movement can cause device-related complications anytime after implantation. Lead migration due to patient movement is a well-described complication in SCS and PNS such as occipital nerve stimulators . These systems are particularly susceptible to lead migration for several reasons. First, they are placed in relatively mobile parts of the body, which exposes the leads and lead extensions to frequent and varied degrees of strain. Second, the ability to anchor the leads in a secure fashion to fixed, bony structures is limited. Finally, the move toward percutaneous placement has resulted in cylindrical electrodes as opposed to paddle electrodes, which have a wider profile and may resist ‘pullout’ more than cylindrical devices . However, even wider paddle electrodes are not immune to migration ( Fig. 18.1 ).

Authors and device manufacturers almost universally recommend having SCS patients limit bending, twisting, stretching, lifting objects greater than 5–10 pounds (2.3–4.5 kg) and other strenuous activities for upwards of 8 weeks after surgery. The goal is to give the system components time to scar into place as much as possible. Some recommend occipital nerve stimulator patients wear a cervical collar for 10 days after surgery to remind them to limit their neck movements . Sudden loss of efficacy and/or sudden appearance of a stimulation-induced adverse event such as paresthesias are clues that lead migration may have occurred. Plain films, particularly when compared to immediate post-implant baseline studies, are the best way to make the diagnosis.
Patients are not immune to movement-related complications beyond the immediate postoperative period. The same ‘scarring down’ that occurs in the first few weeks and months after surgery may make device migration less likely, but it also creates more strain on the components when sudden or significant movements are made. A DBS patient in our practice had been implanted for years with no device-related issues. He was swimming in a lake, and reached his arms up to be pulled out of the water into a boat by his friends. Although he noticed nothing unusual at the time, he gradually began to notice a loss of efficacy on one side of his body. Device interrogation suggested an open circuit, although plain rays were unrevealing. At surgical exploration he was found to have a fracture of one of the lead extension plugs where it entered the IPG ( Fig. 18.2 ).

‘Twiddler’s syndrome’
Twiddler’s syndrome occurs when the IPG rotates or twists within its subcutaneous pocket. There are two recognized forms of this phenomenon; spontaneous and external . In spontaneous twiddler’s syndrome, the IPG moves without external manipulation by the patient. Factors that predispose a patient to spontaneous twiddler’s syndrome include subcutaneous pockets that are too large, become filled with fluid such as seromas, or IPGs that are not anchored. In these circumstances, even normal patient activity can cause twiddling. External twiddler’s syndrome is caused by digital manipulation of the IPG by the patient, usually soon after implantation before the device has had time to scar down.
Either form of twiddler’s syndrome can lead to a number of device complications. The IPGs of many devices must face a certain way for external programmers to be able to interrogate them. If the IPG flips such that it is facing the ‘wrong’ way, the device cannot be accessed. More worrisome are fractures in the lead extensions or plugs that enter the IPG. This is particularly true in devices such as DBS systems with a dual channel IPG, where two lead extensions are running in close proximity. The wires can twist around each other and result in a tremendous amount of strain on the lead extensions ( Fig. 18.3 ). Fractures of the lead extension or plug can result in localized paresthesias, loss of efficacy, and/or current drain from an open circuit with rapid depletion of the IPG. Strategies to prevent twiddler’s syndrome include taking care when creating subcutaneous pockets, tacking the IPG to the wall of the pocket with a non-absorbable suture, and patient counseling. In the past few years, we have placed many of our DBS pulse generators under the pectoralis fascia. These subfascial pockets have much less potential space than subcutaneous pockets, and the incidence of IPG movement and seroma formation seems to have been reduced significantly.

Security and anti-theft systems
Security systems are common in airports, department stores, libraries and other public places, and are therefore a frequently encountered source of electromagnetic interference, (EMI). EMI is the disruption of normal function in an electronic device by the electromagnetic field created by a second electronic device. The likelihood of EMI is related to the amplitude and/or frequency of the external electromagnetic field, as well as its proximity to the patient. The EMI from security devices may be strong enough to turn the device on or off, and can even reset an IPG or change its settings in some instances. Unfortunately, it may be hard for patients to avoid these systems entirely, and they are frequently constructed such that it is impossible for the patient to maintain a safe distance from the system when passing through them. Recommendations are mixed on how to handle encounters with these devices. For VNS, security systems ‘should not’ affect the IPG, but it is recommended that patients keep the IPG at least 16 inches (40 cm) away . Other manufacturers advise patients to avoid security systems when possible by asking to be hand screened by security personnel, with a specific request not to use the hand-held metal detector directly over the IPG. If patients do walk through a security gate, they should temporarily turn the IPG off and pass through as close to the center point of the system as possible (i.e. equidistant from each side of the detector).
Other sources of EMI
Other sources of EMI exist in the environment but are less likely to cause problems for patients. High voltage power lines, microwave transmitters, citizen band or ham radio antennae and other communication equipment are potent sources of EMI; in almost all cases, these devices are fenced-off or have limited physical access to the general public in accordance with Occupational Safety and Health Administration (OSHA) and other governmental agency guidelines. As long as patients respect physical barriers and warning signs, these devices should pose no danger. Other unusual but strong sources of EMI include arc welders, resistance welders, and induction furnaces or burners; patients should avoid getting close to these devices. One case of a DBS patient experiencing symptomatic EMI when driving a hybrid car was recently reported . The symptoms resolved when the patient vacated the front seat of the vehicle or when the IPG was turned off. Such examples underscore the importance of teaching patients to use common sense when they suspect that EMI or some other source of interference is altering the function of their device. In these situations, patients should move away from the suspected source of interference and/or turn their IPG off temporarily.
Some sources of EMI are not necessarily strong but come in close proximity to an IPG during regular use. Examples include dental drills, ultrasonic probes or cleaners (also common in dental offices) and electrolysis wands. Because these sources of EMI are frequently encountered in a dental or medical office, patients are often lulled into a false sense of security that these devices are safe. One manufacturer specifically states that the IPG should be turned off when near these devices, and that a distance of at least 6 inches (15 cm) should be maintained between the device and the IPG . Finally, strong magnets can interfere with the function of some IPGs if they get close enough. Many older IPGs were designed with magnet switches in them, while others like the VNS device have a separate, magnet-induced mode of operation. Common household items that contain strong magnets include stereo speakers and the doors of some refrigerators or freezers. Putting an IPG up against such items is not recommended. Because magnets can change the stimulation mode in VNS, these patients should be specifically warned to stay at least 8 inches (20 cm) away from strong magnets, hair clippers, vibrating devices, anti-theft tag deactivators and loudspeakers .
Medical imaging
One of the most important safety considerations from a medical standpoint is imaging of the patient after the device is implanted. The need for imaging may arise from a desire to confirm the position of an electrode immediately after implantation, or to diagnose a migration or damage to the device after a patient has lost efficacy. The patient may also develop another medical condition that necessitates diagnostic and/or interventional studies. Because the need for imaging may occur emergently as the result of an accident or other acute event, it is important to educate not only the patient but also their caregivers about what type of imaging is safe and what could cause potential problems. If nothing else, they should be provided with emergency phone numbers for either the implanting team, the device manufacturer’s support line, or both. This can avoid device-related complications or withholding of imaging that is safe and would benefit the patient by diagnosing a serious or treatable condition.
Plain radiographs, standard fluoroscopy and computerized tomography (CT) are safe and do not interfere with the normal functioning of neuromodulation systems. Radiographs produce very clear, artifact-free images of the device itself, and are the study of choice for detecting a broken wire, possible disconnection or gross migration of a device component ( Fig. 18.4 ). One manufacturer’s labeling for SCS states that CT scanning can cause a momentary increase in the level of stimulation, which has been reported by patients as a transient electric shock or jolt-like sensation. They recommend turning the SCS off and programming the output to zero when performing CT .

Ultrasound is generally believed to be safe, although some manufacturers say that ultrasound done directly over system components may cause mechanical damage. We have used small, portable ultrasound units used by anesthesia in the operating room to locate the end of a DBS brain lead underneath particularly thick or edematous scalps with no damage to the device and no adverse events. Nuclear medicine studies are also quite common and do not appear to have risk in this patient population. Mammography in patients with IPGs in the chest is safe, but can pose difficulties with positioning the breast tissue in the imaging apparatus. The IPG can also obscure visualization of some of the breast tissue. Patients should warn the mammography technician about the presence of the device to avoid pain and potential damage to the hardware.
Magnetic resonance imaging
Magnetic resonance imaging (MRI) is more problematic for implanted electrical devices. MRI is generally considered the gold standard for intracranial imaging and most spinal imaging. For placement of DBS electrodes, postoperative MRI is extremely helpful in determining electrode location relative to the intended brain target; this facilitates programming of the device, as well as providing the implanting team with important feedback about their surgical technique. MRI is unique in that it uses static and pulsed gradient magnetic fields with pulsed radiofrequency (RF) energy to produce images. While this makes MRI biologically safer to tissues than the ionizing radiation used in plain radiography and CT, potential issues can arise when implanted hardware is present. Ferromagnetic attraction, the phenomenon that can cause sudden and strong pull on ferrous objects as they approach the magnet bore, tends not to be problematic; most modern neuromodulation systems are made of non-ferrous components and therefore are not subject to these forces. However, device screening to assure that it does not contain ferromagnetic material is still mandatory.
Device heating and interference with the normal functioning of the IPG are the greatest concern when performing MRI in these patients. Neuromodulation systems generally have an IPG that is located at some distance from the actual site of stimulation. The two are connected by an insulated wire or wires which, by design, are longer than they need to be to accommodate different anatomical considerations or pulse generator locations, provide some degree of strain relief, allow technical ease in placement and subcutaneous tunneling, etc. As a result, the redundant portion of the wire usually contains random turns and loops that can act as an antenna, locally focusing the energy of the RF pulses and producing heat along the metallic components of the wire and in tissue adjacent to the wires. Since the stimulating surfaces are non-insulated metal directly in contact with neural tissue, any heating that does occur is realized at the metal–tissue interface . Heating can also occur anywhere along the system, and electrical discontinuities such as a break in the lead or lead extension can be particularly problematic. In the brain parenchyma, temperature increases in the 5–7° Celsius range cause reversible tissue dysfunction; increases in excess of 8 °C may cause irreversible thermal injury depending on exposure duration . The most common method of measuring energy absorbed by the body during MRI is to calculate the specific absorption rate, or SAR. SAR is directly related to the RF energy used to excite spins during a given MRI pulse sequence. Some pulse sequences, such as gradient echoes, generally have a low SAR (approximately 0.1–0.5 Watts/kg). Other sequences, such as spin echoes or steady state acquisitions, have an inherently higher SAR (upwards of 3.0 Watts/kg). In general, the higher the SAR for a particular MR sequence, the more potential for heating.
In 2005, Medtronic issued new safety guidelines for performing MRI in patients with implanted DBS systems based on two case reports of adverse events during MR imaging . Both of these adverse events occurred under very specific and unusual circumstances. The incidents occurred in 1.0 T scanners, which are uncommon and use a different radiofrequency wavelength than standard 1.5 T magnets. One case involved the use of a whole body RF coil for spine imaging in a patient with an abdominally placed IPG. Use of a whole body RF coil exposes more of the implanted hardware to RF energy, which can result in higher energy deposition into the device. Moreover, this patient had an abdominally placed IPG, which means that a longer lead extension was used; this may have provided a more robust ‘antenna’ for focal absorption of RF energy. Interestingly, many groups in the past have used whole body RF coils to perform spinal and other studies in DBS implanted patients at 1.5 T without incident . The other adverse event involved scanning a patient with externalized leads, also in a 1.0 T scanner. Again, other groups have scanned patients with externalized leads at 1.5 T without incident, and our group has been implanting DBS leads using interventional MRI since 2004, a technique that involves scanning with externalized leads . The exact causative factors that led to these two patient injuries are still not clear.
As of 2010, the Medtronic guidelines for MRI scanning of implanted DBS systems include using only horizontal bore 1.5 T MRI systems, using only head transmit/receive coils that do not extend over the pulse generator site in the chest, entering the correct patient weight into the MR console (so the SAR for a given sequence is calculated correctly), limiting the gradient field to 20 Tesla/s or less, and using exam parameters that limit the displayed average head SAR (or applied SAR if known) to 0.1 W/kg or less for all RF pulse sequences. MRI should not be performed in the presence of a known broken lead or lead extension. It is also recommended that the electrode array be changed to a bipolar configuration and that the IPG not only be turned off but the amplitude also turned to zero prior to scanning; it is well known that the IPG can be switched on and off during scanning, which is why it is not considered sufficient merely to turn the device off. Avoiding sedation during scanning is also advised so patients can report any untoward events. Finally, if scanning is done with externalized leads, it is recommended that they be kept straight, down the center of the head coil, out of contact with the patient and wrapped in an insulating material .
The labeling for VNS also warns against the use of body coils and spine MRI, although there is a published case report of cervical MRI done emergently for spinal cord compression in a VNS patient without incident . Cranial MRI with VNS is considered safe, provided that the following steps are followed as outlined in the device labeling:
- 1.
use only a head T/R coil
- 2.
set the outputs of the device (both regular output and magnet mode output) to zero
- 3.
use only MR scanners that are 2.0 T or less
- 4.
keep SAR less than 1.3 W/kg for a 70 kg patient
- 5.
keep the time-varying intensity less than 10 Tesla/s .
There is a recent phantom study that shows imaging VNS at 3 T may be safe as well . All manufacturers of SCS systems warn against the use of MRI with their devices, although Medtronic does state that cranial MRI with a head T/R coil is okay . One group has carefully studied patients with SCS undergoing spine MRI at 1.5 T and, although some patients reported sensations of stimulation in the same distribution of their usual SCS and two reported mild heating around the IPG, no permanent or serious adverse events occurred .
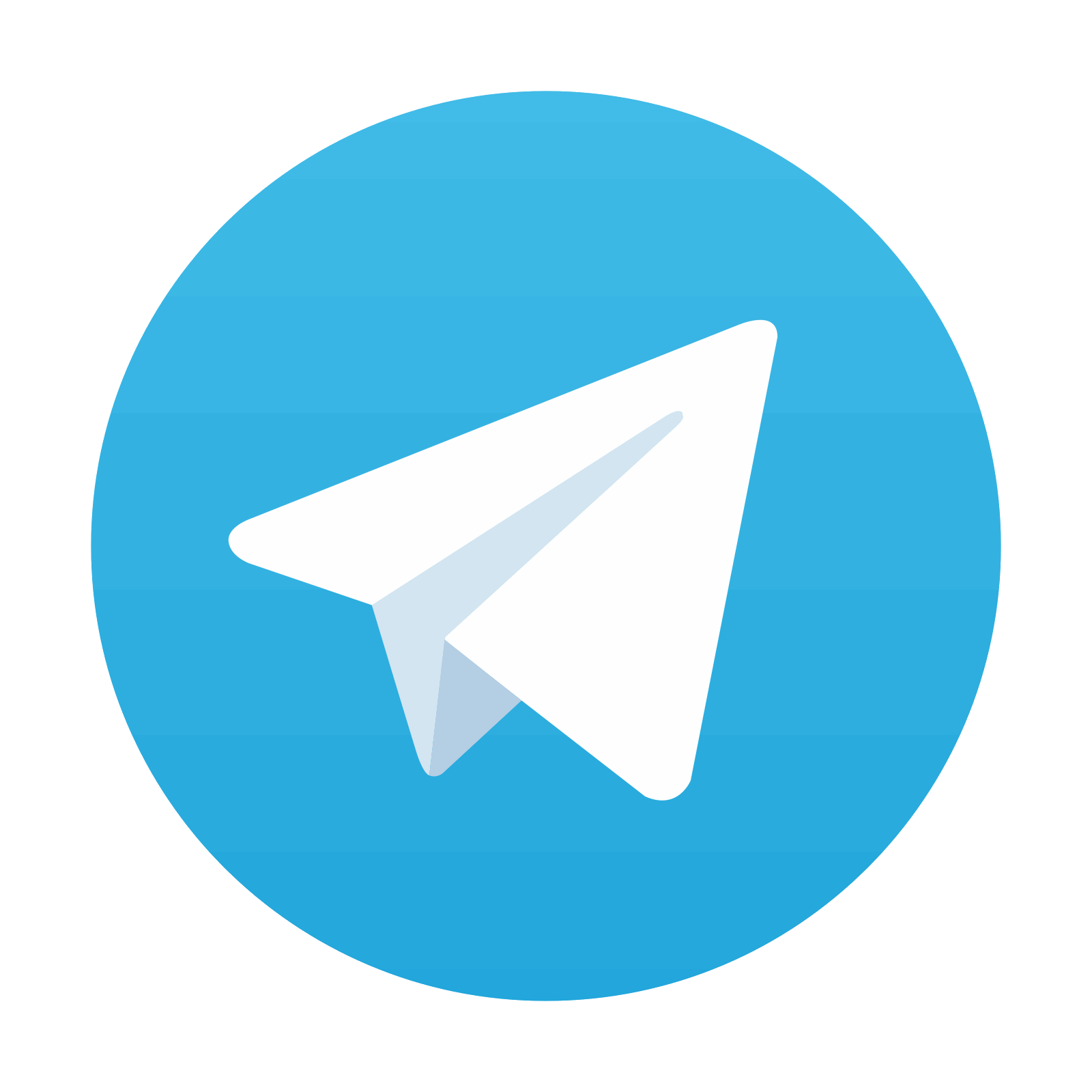
Stay updated, free articles. Join our Telegram channel

Full access? Get Clinical Tree
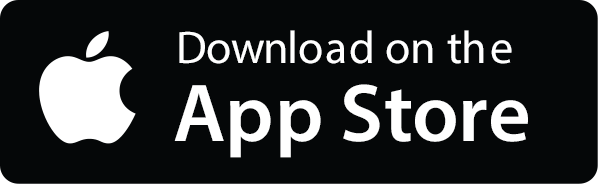
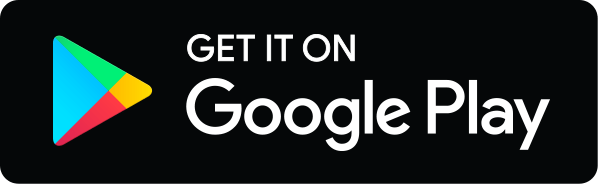