16 Tumors of the Third Ventricle
Abstract
The third ventricle and its surrounding structures represent one of the most complex and intricate areas in the human brain. The area is the vital center of the human cerebrum, responsible for housing our critical neurocognitive functions. In addition, many of the tumors affecting this region are unique to this area. Thus, surgical management of the third ventricular region requires an organized and thorough understanding of the intricate underlying anatomy and a constructive understanding in accessing pathology. The pioneering work of Walter Dandy, Gazi Yaşargil, Michael Apuzzo, and Albert Rhoton Jr. paved the way for a better anatomical understanding of the third ventricle and how to access pathologies affecting this region both safely and effectively. Detailed knowledge of the intrinsic anatomy and its regional constraints is of vital importance in understanding surgical management of the third ventricle. In this review, in an effort to better conceptualize this region, we have organized the third ventricle and built a radial architecture system (outer radial corridor and inner radial corridor) based on the relative position of critical vascular, neural, and cisternal (cerebrospinal fluid–containing) structures. Next, on the basis of this architectural schema, we have highlighted the common pathologic entities affecting this region and integrated our newly structured anatomical concept to discuss perioperative and surgical management, with a focus on choosing the most appropriate surgical corridor using a decision-making algorithm needed to access this region. We hope that this chapter will provide the operator with a more organized and applicable framework in determining which surgical corridor to use in accessing this intimate space.
Introduction
The third ventricle should not be considered as an inert cerebrospinal fluid (CSF)–filled cavity but rather as a part of the central core of the human cerebrum that harbors critical structures responsible not only for our survival but also for our awareness. It is located at the very epicenter of the brain with an intricate geometry, housing a treasure trove of both limbic and neocortical pathways, imperative for survival functions. At the same time, the third ventricle is truly making us individuals by subserving memory, emotion, and even the seat of consciousness.
The third ventricle is unique in that its constituents can generate and harbor a range of pathologies beyond those seen in most other regions of the brain, including inflammatory, neoplastic, vascular, and congenital pathologies. These pathologies can represent conditions varying from sarcoidosis to teratomas. Fortunately, many require only tissue diagnosis, which will guide a variety of precision-based medical and radiotherapies. Surgery for lesions within the third ventricle is inherently challenging because of the depth of the ventricle and the regional neuroanatomy, including the shielding limbic and neocortical white matter tracts. By maximizing the available technology at the time, pioneering neurosurgeons like Walter Dandy, Gazi Yaşargil, Michael Apuzzo, and Albert Rhoton Jr. devised ingenious approaches to the third ventricle. Recently, technological innovations in imaging, optics, white matter tractography, planning software, and robotics have been harmoniously integrated. As a result, these innovations have further enhanced corridor-based surgical approaches. Coupled with rapid advancements in molecular and genetic analysis, the indications for and goals of surgery for third ventricular pathology are being redefined. However, a sound anatomical understanding remains a foundation of treating these conditions irrespective of the modality.
In this chapter, we review these concepts beginning with an understanding of the complex three-dimensional (3D) architectural framework of the region. We devote the initial portion of the chapter to contructing this architecture by building the neural, vascular, and CSF framework, beginning centrally and then spanning out radially. This architecture is important in understanding the variety of pathologies, differential diagnoses based on regional considerations, corridors for access, and absolute limitations imposed by the anatomy.
Anatomical Considerations
The third ventricular region is guarded by a complex glandular or regulatory, neural, vascular, and cisternal architectural framework that must be understood both to appreciate the various pathologic conditions that can cause malfunction and to design safe corridors for access. At the outset, it is important to emphasize that, because of the protected location of the third ventricle, surgical intervention for third ventricular lesions often represents only one aspect of multimodality treatment in their overall management.
We find it useful to geographically divide the third ventricle into three anatomically distinct segments. This categorization forms the basis for a decision algorithm for corridor-based access. The surgical target within the appropriate third ventricle segment becomes the core from which a concentric or radial architecture is built ( Fig. 16.1, Fig. 16.2, Fig. 16.3 ). The next layer is the apertures (membranes and foramina) through which the third ventricle can be accessed. This is followed by an inner radial corridor (IRC) consisting of CSF spaces immediately surrounding the third ventricular segment (ventricles/cisterns) and vasculature. Finally, the outer radial corridor (ORC) consists of the neural structures (cortex, subcortical white matter tracts, and cranial nerves [CNs]) and outer CSF spaces (interhemispheric fissure and sulci).




Somatotopic Organization: Building a Radial Architecture
Third Ventricle Segments
The third ventricle is a narrow, cylindrically shaped, mid-line cavity located in the subcallosal central core of the cerebrum, which is encased by a series of variably sized CSF cavities (i.e., basal cisterns or lateral ventricles [IRCs]). In the following section, we will start with the central core that forms the third ventricle itself (Fig. 16.2) and then work radially to construct a 3D structural and functional architectural model of the region. At its core, the third ventricle represents a dynamic, fluid-filled region that provides a critical pathway for pulsatile flow. Along its anterosuperior margin, it articulates superiorly with the lateral ventricles via the respective bilateral foramina of Monro, while the posteriorly located cerebral aqueduct of Sylvius provides singular outflow to the inferiorly located fourth ventricle.
As previously discussed, based on its subcallosal location, at the anterior and posterior sagittal boundaries, the cylinder becomes C-shaped, carefully guarded by the umbrella of the overlying genu/rostrum of the corpus callosum anteriorly and the splenium of the corpus callosum posteriorly. Next, within each of the anterior, middle, and posterior segments of the third ventricle, it is helpful to consider the boundaries of the cylinder as a floor, a roof, and two lateral walls extending between the two C-shaped borders of the cylinder (i.e., anteriorly being the optic recess and posteriorly being the pineal recess).
The third ventricle itself can be divided into three segments in the sagittal plane ( Fig. 16.2 ). If we draw a line along the anterior commissure and venous angle, which represents the confluence of the caudate, septal, and thalamostriate veins as they join to form the origin of the internal cerebral veins (ICV), we can consider all structures anterior to this as the anterior segment of the third ventricle. This region is well approximated by the foramen of Monro, and the anterior commissure is located directly anterior and slightly inferior to the foramen of Monro, which marks the venous angle.
Continuing posteriorly along the sagittal plane, the lateral wall of the third ventricle takes on the shape of the thalamus as it creates bilateral large almond-shaped impressions along the ependyma, with the massa intermedia or interthalamic adhesion extending across the ventricle. We define the middle segment of the third ventricle as the region between the anterior commissure (the foramen of Monro as a surrogate landmark) anteriorly and the massa intermedia posteriorly. Therefore, the posterior segment now consists of the region posterior to the massa intermedia, incorporating the pineal gland, including the suprapineal and pineal recesses posteriorly ( Fig. 16.2 ).
Anterior Segment
Within the anterior segment of the third ventricle, the critical neural framework moving radially outward along the lateral walls consists of the anterior portion of the hypothalamus, and the floor of the third ventricle is bordered rostrally by the optic chiasm and ventrally by the infundibular recess. These rostral and ventral borders are bounded by specific apertures that communicate with the anterior segment, namely, the lamina terminalis and infundibular recess ( Fig. 16.1, Fig. 16.2, Fig. 16.3 ). In addition, the anterior segment harbors critical communication pathways and glandular/regulatory mechanisms of the hypothalamic core consisting of the tuber cinereum and a infundibulum. The mammillary bodies are also located in this segment. The remainder of the neural architecture consists of the roof of the anterior portion of the third ventricle, which is guarded sequentially in a radial fashion by the interhemispheric fissure, the base of the caudate, and the genus of the corpus callosum. The vascular framework consists superiorly of the venous angle marking the posterior boundary and transition between the anterior and middle third of the third ventricle, anteriorly by the anterior communicating artery complex and the hypothalamic perforators, and inferiorly by the basilar apex and the respective posterior cerebral artery (PCA) perforators (see section on cerebrovasculature).
Middle Segment
There is a compact transitional zone between each of the segments that harbors critical structures. This is especially true for the zone between the anterior and middle segments. In keeping with the geometric theme of the corpus callosum, the fornix also bends along its anterior and posterior margins. The anterior arch consists of the fornicial bundles along the perimeter of the foramen of Monro in the transitional zone between the anterior and middle segments of the third ventricle. The remainder of the middle segment posterior to this transitional zone forms the body of the third ventricle. In this segment, the roof of the third ventricle is bordered bilaterally by the bodies and the crura of the fornices. Traversing posteriorly along the roof of the third ventricle, the two fornices diverge laterally, and the ensuing gap is spanned by the hippocampal commissure. Beneath it lie the two layers of the tela choroidea and the intervening space, the velum interpositum, which contains the ICV. As they course through the velum interpositum, the ICV receive tributaries from the thalamus, the fornix, and the walls of the third ventricle. 1 They will then exit the velum interpositum above the pineal body to enter the quadrigeminal cistern and join the great vein of Galen (see section on cerebrovasculature) ( Fig. 16.4 ).

Along the anterior transitional zone, the lateral walls of the third ventricle are intimately bordered on each side by the hypothalamus, which transitions into the anterior thalamus as one travels posteriorly along the body of the middle segment of the third ventricle. Of note, the internal capsule and genu of the corpus callosum are intimately located along the superolateral border. The massa intermedia, or interthalamic adhesion, travels across the third ventricle connecting the respective thalami, and marks the posterior boundary of the middle segment. The floor of the middle segment is made up of the mammillary bodies and the diencephalon, as well as the critical anterior and posterior circulation perforators supplying this vital portion of the brain stem (see section on cerebrovasculature).
Posterior Segment
There is an analogous posterior transition from the middle to the posterior segment that is most prominently formed by the posterior portion of the thalamus, located behind the massa intermedia. The roof of the posterior segment itself is formed by the relationship of the tela choroidea, velum interpositum, and ICV and their confluence with the great vein of Galen. The posterior segment harbors the habenula and body of the fornix, which is located just inferolaterally to the posterior margin of the thalamus.
The posterior segment has two key CSF spaces, the cerebral aqueduct inferiorly and the suprapineal recess superiorly. The habenular commissure, pineal recess and pineal gland, and posterior commissure are all located within this space. The posterior boundary of the cylinder, as previously noted, is C-shaped and located under the umbrella of the splenium of the corpus callosum. The pineal body projects from this posterior wall of the third ventricle into the quadrigeminal cistern, where it lies beneath the splenium of the corpus callosum and above the cerebellar vermis. Different from the anterior C-shaped boundary of the third ventricle, the lamina terminalis, the posterior C-shaped boundary is formed by a thin pineal stalk that extends from the thalamus to the overlying pineal gland. This is carefully guarded by vital structures that preclude it from being a meaningful aperture into the third ventricle. Knowledge of this difference is critical for understanding surgical corridors and their limitations.
Inner Radial Corridor: Cerebrospinal Fluid Spaces
The third ventricle is unique in that it represents a CSF space that is radially surrounded by other CSF spaces and cavities, which are then separated from the third ventricle by membranes (lamina terminalis, infundibular recess, and tela choroidea/velum interpositum) or foramina (foramen of Monro) and by the cerebral aqueduct of Sylvius ( Fig. 16.1, Fig. 16.2, Fig. 16.3 ). In constructing 3D architecture that extends radially and, in particular, in understanding access corridors, knowledge of this anatomy becomes imperative. Explicitly, the third ventricle cannot be accessed directly without going through a CSF cistern and its bounding membrane or a CSF cavity through its foramen ( Fig. 16.2 ):
Anterior segment: The anterior segment can be reached superiorly, anteriorly, and inferiorly.
Superior access from the lateral ventricle is through a foramen, the foramen of Monro.
Anterior access from the suprachiasmatic cistern is through a membrane, the lamina terminalis.
Inferior access, via an expanded endonasal approach (EEA) and pituitary transposition, allows access to the interpeduncular cistern and then, through a membrane, to the infundibular recess.
Middle segment: The middle segment can be reached anteriorly and superiorly.
Anterior access from the lateral ventricle is through a foramen, the foramen of Monro. Access is limited based on the dilation of the foramen of Monro.
Superior access from the lateral ventricle is through a membrane, the tela choroidea and velum interpositum.
Posterior segment: The posterior segment can be reached but surgical options are limited.
Superior access from the lateral ventricle is through a membrane, the tela choroidea and velum interpositum; however, the confluence of the ICV and massa intermedia preclude surgical application as the corridor becomes limited and constraining for bimanual dissection.
Posterior access from the quadrigeminal cistern, unless following an extrinsic lesion, is impractical because there is no meaningful access membrane or foramen. From a rostrocaudal direction, the great vein of Galen, the pineal gland, and the tectal plate of the midbrain create a formidable barrier to entry from the quadrigeminal cistern.
Inferior access from the fourth ventricle through a foramen, the cerebral aqueduct of Sylvius, is also surgically impractical.
Inner Radial Corridor: Regional Cerebrovasculature
While we have alluded to some key arterial and venous relationships in this region, it is imperative to understand them in detail in order to truly understand the 3D architectural framework that guards the third ventricle ( Fig. 16.4 ). The anterior cerebral arteries and the anterior communicating artery lie immediately anterior to the anterior boundary (optic recess) of the anterior segment of the third ventricle, marked by the lamina terminalis. The perforators from the anterior communicating artery and the distal A1 segments supply the anterior wall. Similarly, the posterior wall (pineal recess) is situated next to the posterior cerebral, posterior choroidal, pericallosal, and superior cerebellar arteries. The floor of the third ventricle provides a perforated and fissured corridor for critical vessels, including the posterior communicating arteries and the apex of the basilar artery. Both the anterior and posterior cerebral arteries supply the roof of the third ventricle. Finally, it is important to note that all major intracranial vessels, such as the anterior and posterior cerebral arteries, internal carotid, anterior choroidal, and anterior and posterior communicating arteries, provide perforators that feed into the walls of the third ventricle. 1 In effect, there is a rich radial plexus that supplies and irrigates this vital compact area.
With respect to the venous plexus, the anterior and posterior transitional segments represent key confluences, specifically, the venous angle anteriorly with the ICV running along the body of the third ventricle, within the velum interpositum and toward its posterior confluence, the great vein of Galen, in the region of the suprapineal recess. The choroid plexus essentially runs along this venous framework and is intimately tied to it, particularly along the tela choroidea ( Fig. 16.4 ).
Outer Radial Corridor: Neural Structures
The ORC consists of a neural structure primarily composed of cortical structures, subcortical parenchyma, and CNs. The cortical structures are composed of the respective gyri, while the sub-cortical region consists of the underlying white matter fascicles. The largest white matter fascicle is represented by the corpus callosum, which represents the midline commissural fiber connecting the hemispheres. Again, consistent with the architectural theme of the midline structures, the corpus callosum is also divided into thirds (anterior, middle, and posterior). In addition to the corpus callosum, it must be noted that there is increasing evidence of the presence of eloquent and vital white matter fascicles, such as key association fibers (the superior longitudinal fasciculus and cingulum) and projection fibers (anterior limb of the internal capsule). These fibers are vital to neurocognitive, behavioral, and motor initiation pathways. Finally, the key CNs that form the ORC are represented by the optic apparatus, optic nerve, chiasm, and tract.
Outer Radial Corridor: Cerebrospinal Fluid Spaces
The CSF pathways in this ORC consist of key sulci, the most prominent of which is the interhemispheric fissure separating the two hemispheres. Analogously, there are many such smaller intervening CSF spaces between the gyri (i.e., the sulci). These sulci can provide similar access to the IRCs, which is analogous to the interhemispheric fissure providing access to the lateral ventricle.
Differential Diagnosis, Pathophysiology, and Natural History
A variety of congenital and acquired lesions can affect the third ventricle. Furthermore, these may be intrinsic, intrinsic and exophytic, extrinsic, or extrinsic and endophytic. There are also age and segmental predilections. The most common representative pathologies are summarized in Table 16.1 .
Congenital Pathology
The transitional zone between the anterior and middle segment is most commonly affected by congenital lesions, of which colloid cysts are the most prevalent. Colloid cysts are round, well-defined, fluid-filled cysts that can range in size from several millimeters to 3 cm. The behavior of these cysts varies, as 90% are reported to be asymptomatic and stable, and 10% tend to enlarge and cause obstructive hydrocephalus. 2 In fact, de novo cysts in adults are rare, and they are often dormant congenital remnants that eventually grow. However, the trigger for their sudden growth in adulthood remains unknown. 3 , 4 , 5
We have classified colloid cysts into two types based on their primary site of attachment. Colloid cysts are commonly attached to the lateral ventricle, then grow inferiorly through the foramen of Monro and into the transitional zone entering the third ventricle. We refer to these cysts as type I colloid cysts. However, less commonly they can be attached along the roof of the third ventricle in the transitional zone between the anterior and middle segments. This location is just posterior to the venous angle, where they then can grow superiorly from this pedicled attachment along the roof of the middle segment toward the foramen of Monro, causing intermittent obstruction. We refer to these as type II colloid cysts. Type II colloid cysts create intermittent compression by swinging forward from their pedicle and obstructing the CSF flow until the intraventricular pressure within the lateral ventricle creates enough pressure to dislodge them from the foramen of Monro, which forces them to swing back into their original position. In essence, this represents a “ball-valve phenomenon.” The clinical and surgical implications of type I and type II colloid cysts will be discussed subsequently.
Other congenital intraventricular cysts are rare but may also represent important causes of hydrocephalus, especially in the pediatric population. Their origin can be arachnoidal, endodermal, or neuroepithelial, and they can occur in any segment of the third ventricle, resulting in obstruction at the level of the acqueduct of Sylvius or the foramen of Monro. Ependymal cysts are often small, benign, and asymptomatic and can also be found in the lateral ventricles extending into the third ventricle. 2 , 6
Acquired Pathology
We have found that the segmentation scheme discussed above has also proven to be valuable because of the geographically dependent nature of acquired pathologies of or around the third ventricle. The differential diagnosis of extrinsic masses in the respective segment of the third ventricle should be considered on the basis of two factors: the age of the patient and the regional anatomy primarily affected.
Anterior Segment
Extrinsic
The age of the patient significantly impacts the likelihood of pathology, which dramatically differs between the pediatric and adult populations. Tumors in the anterior third ventricle can be divided into those arising from structures along the floor (sellarsuprasellar) and those arising from the lateral and anterior wall (hypothalamic-chiasmatic). Tumors originating in the sellarsuprasellar space that then extend upward toward the anterior recess and cause extrinsic compression most commonly include pituitary macroadenomas, craniopharyngiomas, and meningiomas. In contrast, the hypothalamic and optic gliomas represent more common extrinsic lesions of the lateral wall and the anterior terminus of the region, respectively.
The age distribution is particularly important in considering these lesions. In the pediatric population, the most common extrinsic anterior mass among patients younger than 2 years is Langerhans cell histiocytosis. Hypothalamic-chiasmatic pilocytic astrocytomas and craniopharyngiomas tend to occur between the ages of 5 and 15 years. Craniopharyngiomas, in particular, tend to be heterogeneous in appearance, especially with the presence of calcifications. Independent of the nature of the specific lesion, they most often manifest physiologically with secondary impairment of the glandular and regulatory functions of the anterior third ventricle, such as diabetes insipidus and hypopituitarism, or secondary to mass effect on local structures, in particular the optic apparatus.
Intrinsic
Intrinsic lesions of the anterior third ventricle are much rarer. Germinomas and lymphomas are the most common intrinsic lesions to occupy the anterior segment of the third ventricle. The incidence of germinoma peaks at approximately 10 to 12 years of age while lymphomas more commonly arise in the third or fourth decades of life. Other lesions in the adult population that should be considered as originating primarily intrinsic to the anterior third ventricle segment are papillary craniopharyngiomas. We have previously described these as type IV craniopharyngiomas, and their surgical implications will be discussed subsequently. 7
While the site of origin may provide a clue in the case of extrinsic lesions, it can still be difficult to determine their nature; this is even more difficult in cases of intrinsic lesions. With respect to anterior masses affecting the third ventricle in the adult population, it can often be difficult to differentiate among the various potential pathologies to reach a final diagnosis. Mangetic resonance imaging (MRI) with gadolinium-enhanced sequences and, more recently, with cellular diffusion-weighted imaging may be of value. However, direct biopsy and CSF sampling are usually required to confirm clinical suspicion. Of the list of possible pathologies, pituitary macroadenomas and meningomas are the most common. Since these are slow-growing tumors, displacement, rather than invasion of the third ventricle, is the usual consequence.
Craniopharyngiomas represent a unique entity. We have previously classified them into four categories.
Type I: Suprasellar preinfundibular
Type II: Suprasellar transinfundibular
Type III: Interpeduncular retroinfundibular
Type IV: Anterior recess intrinsic third ventricular
While it can be difficult to determine the specifics on radiologic diagnosis, for the purposes of this chapter, the only relevant issue is whether the stalk and infundibular recess are normal. If so, the lesion is more likely to be type IV and intrinsic to the third ventricle, unlike other tumors that will impact the infundibulum, resulting in posterior displacement (type I), dilatation (type II), or anterior displacement of the stalk (type III). The surgical relevance will be discussed later but, in essence, if the stalk is normal (type IV), a ventral anterior endonasal route is not preferred and a transventricular route (dorsal anterior) is selected instead. Other pathologic processes, such as lymphomas, metastases, and granulomatous diseases, may have a similar appearance on computed tomography or MRI. Occasionally, parasellar and tuberculum meningiomas can extend far superiorly and thereby deform the anterior recess of the third ventricle. 8 , 9 , 10
In addition, the anterior portion of the third ventricle and the hypothalamus are occasionally involved with other intrinsic tumors, such as ependymoma, glioblastoma multiforme, and ganglioglioma. Metastatic tumors rarely occur intrinsically in the anterior segment of the third ventricle without involving the choroid plexus directly. 6 , 11 , 12 , 13
Transitional Zone (Anterior/Middle Segment)
Masses of the foramen of Monro frequently manifest with obstruction of the lateral ventricles. The most common pediatric lesion affecting the foramen of Monro is the subependymal giant cell tumor associated with tuberous sclerosis. Approximately 20% of patients with tuberous sclerosis have subependymal giant cell tumors, which typically occur in patients younger than 20 years of age. Subependymal nodules and hamartomas, which are key intracranial features of tuberous sclerosis, can occur anywhere along the ventricular walls. Subependymomas (World Health Organization [WHO] grade I), which are closely related to SGCTs, more often occur in the fourth ventricle in middle-aged to elderly patients. However, supratentorially, this lesion can occur in the foramen of Monro as a small, lobulated, well-defined lesion that can invade both the lateral and third ventricles. 14 , 15 , 16
Middle Segment
Extrinsic
Masses arising from the floor of the third ventricle are uncommon. Hypothalamic hamartomas are nonneoplastic processes that typically manifest in childhood and can upwardly distort the floor of the third ventricle. Dermoid, epidermoid, or arachnoid cysts that arise from the prepontine cistern can also cause compression of the floor. In addition, basilar artery aneurysms and basilar artery ectasias can project directly to the prepontine cistern, causing third ventricular compression and often even mimicking colloid cysts. 17 , 18 , 19 , 20
Intrinsic
Intrinsically derived intraventricular lesions of the middle segment of the third ventricle are rare. Most of these lesions involve the choroid plexus. 21 They include primary choroid plexus papilloma, choroid plexus carcinoma, metastatic processes, vascular malformation, and infectious processes (e.g., neurocysticercosis) infiltrating the plexus. Choroid plexus cysts are neuroepithelial in origin and are often associated with chromosomal abnormalities. In addition, seeding of both primary and secondary lymphomas may occur within the choroid plexus. These lesions, however, very rarely occur within the third ventricular space and instead are more common in the lateral and fourth ventricles.
Choroid plexus papillomas, which more commonly occur in the fourth ventricle in adults, can grow in the third ventricle in 5% of the population. These lesions are derived from choroid plexus epithelium and often are associated with nonobstructive hydrocephalus due to CSF overproduction or impaired resorption. Chordoid glioma is also another rare, slow-growing, benign neoplasm that is derived from both chordoid and glial cellular progenitors. Of note, these can also involve the anterior segment. 6 , 11 , 12 , 13 , 22 , 23 , 24
Posterior Segment
Extrinsic
While there is some controversy about whether lesions of the pineal regions should be considered intrinsic or extrinsic, we prefer to consider them extrinsic because of the location of the pineal gland within the quadrigeminal cistern. Most tumors of the posterior segment of the third ventricle that originate in the pineal gland are extrinsic to the ventricle but then grow toward and distort the suprapineal and pineal recesses along the posterior C-shaped terminus of the ventricle. These pathologic conditions represent the most relevant considerations with respect to lesions of the posterior third ventricle.
A wide range of pathologic processes can occur—from asymptomatic cysts to pineocytomas, pineoblastomas, other germ cell tumors, and teratomas. 25 , 26 Tumors in this area in the adult population rarely cause obstruction and hydrocephalus. Pediatric tumors in the posterior pineal region tend to cause hydrocephalus even with small-sized lesions. Other tumors mentioned previously, such as pineocytomas or pineoblastomas (WHO grade IV), more often occur in teenagers and young adults and tend to be more aggressive. These tumors are considered to be neuroectodermal in origin, and they closely resemble medulloblastomas, ependymomas, retinoblastomas, and supratentorial primitive neuroectodermal tumors on imaging and histologically.
Intrinsic
In the adult population, the most common intrinsic tumors in this region are germinomas and lymphomas. 27
Clinical Presentation
The clinical manifestations of third ventricular lesions can be categorized by those that result in CSF obstruction and hydrocephalus and those that impact the function of the respective segments of the third ventricle via intrinsic invasion or extrinsic compression.
Cerebrospinal Fluid Pathways
Most lesions within or surrounding the third ventricle result in hydrocephalus, which is caused by obstruction of either the foramen of Monro superiorly or the cerebral aqueduct inferiorly. Disruption of the contour, either by intrinsically or, more commonly, by extrinsically applied forces can result in dilation of the third ventricle. Symptoms related to increased intracranial pressure, including headache, nausea, vomiting, papilledema, and cognitive impairment, can occur in such patients.
Regional Effects
Endocrinologic deficits and visual disturbances usually manifest from extrinsic tumors that compress the third ventricle, especially along its anterior wall or floor. This can be the case especially with tumors originating from the sellar-suprasellar-chiasmatic axis. Involvement of, or mass effect from, the hypothalamus on the third ventricle can cause diencephalic syndrome, which can result in alterations in satiety (emaciation or obesity) or alertness (somnolence and hyperalertness) in children. Lesions affecting the middle segment and transitional zone will often result in forniceal and limbic system impairment, with consequent memory and processing dysfunction. Lesions located along the posterior wall of the third ventricle can create mass effect on the pretectal region. Parinaud’s syndrome (upward gaze palsy) can result, especially in infants, because this region is much more sensitive to compression at a young age. 28 , 29 , 30
Perioperative Evaluation and Surgical Management
As hydrocephalus is a common presentation, the first course of action is often to reduce the intracranial pressure. This can be accomplished either by CSF flow diversion (i.e., ventriculoperitoneal shunt) or by opening internal membranes to allow diversion into the basal cisterns (e.g., endoscopic third ventriculostomy, septum pellucidotomy). Cytoreduction or obtaining diagnostic tissue, as well as restoration of CSF flow, are the typical surgical goals. Adjuvant chemotherapy or radiotherapy can be an effective addition to surgical treatment and will be discussed later in this chapter.
Chemotherapeutic Considerations
The treatment of patients with high-grade and lower-grade gliomas affecting the third ventricle follows established treatment protocols. This section will focus on medical therapies for nonglioma lesions and rare third ventricle–specific glial or mixed neoplasms.
Pituitary Macroadenomas
For residual or recurrent hormonally active adenomas or multiple recurrent nonsecreting tumors not amenable to surgical intervention, radiotherapy and chemotherapy can be options. For refractory or aggressive corticotrophin pituitary tumors with rapid growth, local invasion, evolving cranial neuropathies, and the potential for CSF dissemination, CAPTEM, a chemotherapeutic regimen combining capecitabine and temozolomide has been reported, in a small cohort, to lead to radiographically confirmed high control rates (in all four patients treated) associated with dramatic improvements in both neurologic deficits and symptoms of Cushing’s disease. 31 As expected, the dramatic treatment response (complete radiographic regression) to temozolomide correlated with low MGMT (O6-methylguanine-DNA methyltransferase [a DNA damage repair gene]) expression levels and with an intact mismatch repair system (adequate expresion levels of MLH1, MSH2, MSH6, and PMS2). 31
Temozolomide is emerging as a viable treatment option for pituitary adenoma. A correlative study on 136 consecutive prolactinoma specimens showed low MGMT expression (< 25% immunopositivity) in more than 75% of the tumor specimens, correlating with a high rate of MGMT promoter methylation—a trend that was even more frequent in atypical prolactinomas. 32
Furthermore, disulfiram, an acetaldehyde inhibitor used in the treatment of alcoholism, has been reported to both directly inhibit MGMT expression in a variety of tumors 33 and decrease MGMT levels by enhancing MGMT protein proteasomal degradation in an in vitro pituitary adenoma model, 34 making DSF an attractive add-on to a temozolomide-containing regimen for treatment of refractory prolactinomas, particularly when MGMT is highly expressed.
Craniopharyngiomas
Residual or recurrent craniopharyngioma not amenable to further surgical intervention should be considered for fractionated radiotherapy. Additional medical therapies are also being developed. Genomic profiling suggests that the treatment landscape of recurrent craniopharyngiomas will move rapidly beyond the accepted but infrequently used techniques of intracavitary treatment with radioactive phosphorus, bleomycin, or interferon-α, all technically and logistically cumbersome and all associated with unacceptable toxicities. Given some of the anatomical limitations and the risk of radiation toxicity, including the overall increased lifetime risk for radiation-induced gliomas, it is likely that genomic profiling will completely shift the focus away from initial aggressive surgery and radiation in the treatment of craniopharyngiomas.
Papillary craniopharyngiomas, which occur almost exclusively in adults, show a high rate (90%) of BRAF V600E mutation 35 constitutively activating the MAPK pathway, making BRAF and MEK inhibitor combinations potentially effective treatment alternatives. 36 It is unclear whether BRAF and MEK inhibitor combinations will lead to durable radiologic results, and the most reasonable way toward a cure will likely require— after size reduction with combination therapy—radical resection of residual craniopharyngioma followed by radiotherapy. 36 In a case report where there was significant reduction in size of a large craniopharyngioma with single-agent vemurafenib (a BRAF inhibitor), there was rapid regrowth within 6 weeks after discontinuation of therapy. 37
Adamantinomatous craniopharyngiomas occur in both children and adults. They have been reported to exhibit a high β-catenin (CTNNB1 [catenin β 1]) mutation rate (over 90%). β-catenin is an integral part of the canonical WNT signaling pathway that upregulates MGMT, 38 which suggests that an MGMT-targeted strategy may be a feasible treatment alternative in the treatment of adamantinomatous craniopharyngiomas.
Meningiomas
Meningiomas can involve any part of the third ventricle. They are more frequently extrinsic lesions of the anterior segment, less frequently involving the posterior segment, and they are rarely found to be entirely intrinsic lesions. The upfront standard treatment of meningiomas, following surgery with the intent of maximal safe resection, is based on their proliferation index, type, and grade, with radiotherapy frequently being used to prevent regrowth.
Some current guidelines recommend only three drugs as systemic therapy for refractory and high-grade meningioma— interferon α2b, hydroxyurea, and octreotide. However, none of these options is commonly used in daily clinical practice because of their modest clinical activity. 39
Therapies initially explored in the early 1990s that combined interferon α2b and hydroxyurea have proven to have limited activity in recurrent high-grade meningiomas. 40 They are also associated with moderate adverse effects and are not frequently used in the treatment of recurrent meningiomas in clinical practice.
Various single-agent targeted therapies have been studied in clinical trials. These include somatostatin, temozolomide, irinotecan, interferon-α, mifepristone, megestrol acetate, PDGFR inhibition (imatinib), EGFR inhibition (erlotinib), and VEGFR inhibition (sunitinib and vatalanib), with modest results and often associated with significant toxicity. 41 , 42 , 43
Bevacizumab remains the only single-agent therapy reported in case reports and limited trials (phase 2) to potentially lead to a more durable clinical control at times associated with radiologic response in the treatment of recurrent meningioma. 44 , 45 , 46 However, some have questioned the radiologic response, suggesting that it may be due to effective treatment of the underlying radionecrosis, 47 which could be easily determined, given the sophisticated MRI techniques uniformly available in clinical practice today. Bevacizumab dose and treatment density remain significant contentious issues across the cancer literature and reflect the current clinical trial design conceived to evaluate cytotoxic drugs, not allowing for the important distinction between maximum tolerated dose and optimal biologic dose. 48 , 49
There are interesting results reported with bevacizumab-based combinations using add-on drugs such as everolimus 50 and paclitaxel. 51 There is a rapidly growing body of literature involving genomics and pathologic correlative studies describing a relatively small number of oncogenic driver mutations, which turn out to be type-, grade-, and intracranial location–specific. 52 , 53 The NF2 gene is altered across all meningiomas, while AKT1, SMO, TRAF7, and KLF4 are altered in WHO grade I meningiomas; CDKN2A/C, SMARCE1, and TERT are altered in WHO grade II meningiomas; and CDKN2A/C and TERT are altered in WHO grade III meningiomas, 52 with TERT promoter mutations predicting a shorter time to progression. 54 , 55
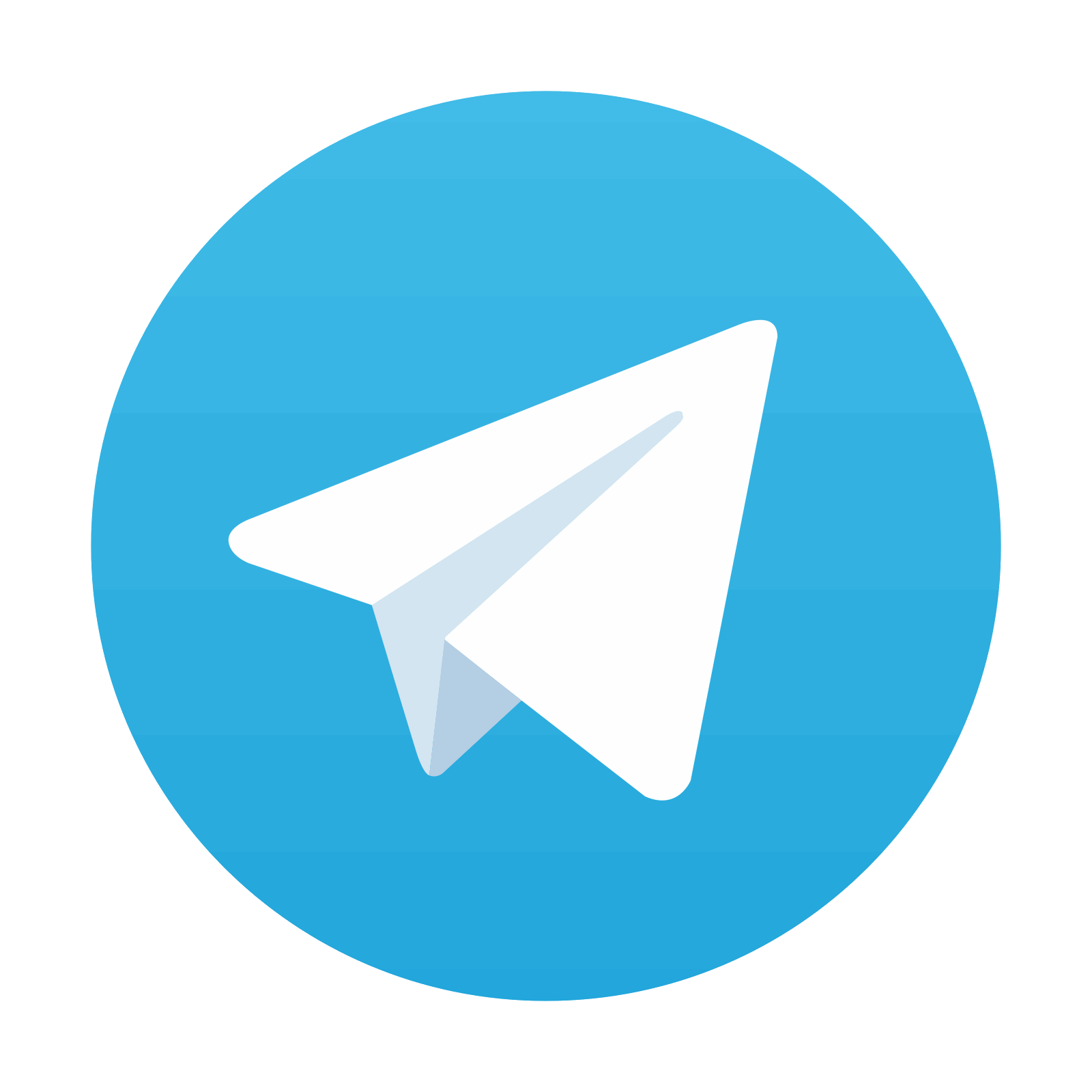
Stay updated, free articles. Join our Telegram channel

Full access? Get Clinical Tree
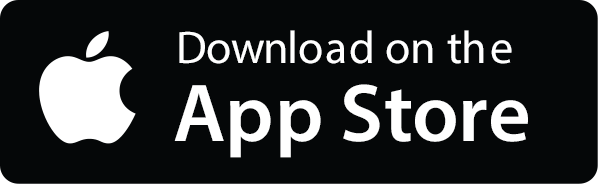
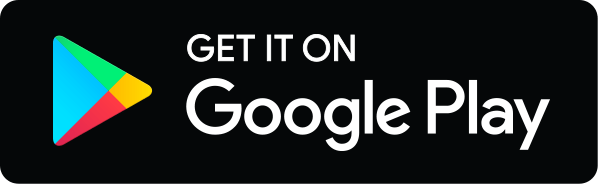
