l-Asparaginase
Chlorambucil
Interferon-a
Bevacuzimab
Cyclosporin A
Lomustine
Carmustine
Etoposide
Methotrexate
Cisplatin
5-Fluorouracil
Procarbazine
Busulphan
Ifosfamide
Vincristine
Table 9.2
Semiology and aetiology of new-onset seizures in cancer
Semiology | Aetiological condition |
---|---|
Isolated generalized tonic–clonic seizures/seizure clusters | Most drug-induced seizures Metabolic encephalopathy Endocrine tumors Intracranial metastasis CNS infections Viral encephalitis Parenchymal space-occupying infections Cerebral venous sinus thrombosis |
Myoclonic seizures | Busulfan |
Photosensitive seizures | Interferon-alpha |
Multiple focal seizures/varying semiology | Intracranial metastasis Multifocal parenchymal infections Paraneoplastic encephalomyelitis |
Status epilepticus | Cyclosporine Toxic drug overdose Drug-induced seizures in the setting of impaired clearance |
Non-convulsive status epilepticus (a) Generalized (b) Complex partial | Ifosfamide Paraneoplastic limbic encephalitis Limbic encephalitis due to herpes virus Cyclosporine |
Epilepsia partialis continua | Paraneoplastic encephalomyelitis Venous sinus thrombosis with venous infarct |
Unprovoked recurrent seizures, easily controlled with epilepsy drugs Unprovoked seizures | Methotrexate-radiation Leukoencephalopathy Following cerebral ischemia or hemorrhage CNS infections |
Intractable complex partial seizures/epilepsy | Paraneoplastic limbic encephalitis Delayed radiation necrosis Herpes virus-induced encephalitis |
Brain Tumors
Epilepsy occurs in 13% of all cancer patients and accounts for 5% of neurological manifestations. Half of all seizures in systemic cancer are associated with brain or leptomeningeal metastasis [1, 2]. Epilepsy may be the first sign of cancer either as manifestation of a primary brain tumor or from systemic cancer that presents with brain metastasis. Seizures are also an early sign of paraneoplastic encephalomyelitis, an intriguing albeit rare manifestation of cancer.
Brain Metastasis
Seizures occur as the presenting symptom in 18% of patients with brain metastases; another 15% develop seizures later in the course of the disease [1, 4, 5]. The incidence varies from 16% with breast cancer, 21% with gastrointestinal metastases, 29% in lung cancer patients and up to 70% with melanoma, possibly due to its predisposition for intratumoral hemorrhage. In a retrospective study of 470 patients with brain metastases of any type, 24% of patients had one or more seizures at any point in their disease course [6]. The incidence can be higher for patients with metastases involving or adjacent to brain regions of high epileptogenicity, such as motor cortex, and in patients with multiple brain metastases.
Meningiomas
About one-third of patients with meningioma present with epilepsy, of whom 70% have secondary generalized and 30% partial seizures. Seizures are more frequently seen with convexity-based lesions than with tumors in other regions, and are more common in the presence of manifest peritumoral edema [7–9]. Epileptic seizures occur in 30% of patients before surgery and in 26.6% patients after surgery. Of patients with preoperative epilepsy, 59.0% become seizure-free after surgery, and 20% of those without preoperative seizures will develop epilepsy after surgery. Risk factors for postoperative epilepsy are preoperative epilepsy, surgical complications including CNS infections, repeat craniotomy, intracranial hemorrhage, and tumor progression. Postoperative improvement or recovery from preoperative neurologic deficits is associated with improved seizure control [8, 9].
Neuroepithelial Tumors
DNETs
In dysembryoblastic neuroepithelial tumors (DNETs) , seizures affect essentially 100% of patients with an average age of 15 years at presentation, and 50% of patients have complex partial seizures with or without secondary generalization. On MRI, 80% of the tumors are located in the temporal lobe, 16% in the frontal lobe, and the remainder in other cortical regions [10, 11].
Gangliogliomas
Among patients with gangliogliomas, 80–90% have seizures, usually as the first and only clinical symptom, at an average age of onset of 17–21 years. Of these, 74% are complex partial, 43% generalized tonic-clonic/mixed, 12% simple partial, and 6% absence seizures. The majority of gangliogliomas are located in the temporal lobe and display staining for the CD34 glycoprotein, in contrast to gangliogliomas located elsewhere [11–13].
Gliomas
Astrocytomas are frequently located in temporal or insular locations, while oligodendrogliomas have a predilection for the frontal lobe [14, 15]. Oligodendroglial tumors more often involve the cortex and result in a higher risk of seizures as compared to astrocytomas, which tend to occur in the white matter. Frontal, temporal, insular, and parietal lobe tumors are more commonly associated with seizures as opposed to occipital and midline tumors. Localization to functional cortex renders tumors particularly epileptogenic.
Anaplastic astrocytomas and high-grade gliomas with temporal lobe or cortical involvement can be associated with preoperative seizures. In contrast, older age and larger tumor size are less commonly associated with preoperative seizures. Seizure-free outcome at 12 months following surgery is 77% [16]. A lower incidence of seizures is seen in de novo GBMs compared to secondary GBMs (e.g., those that developed from prior low-grade gliomas) [17, 18].
Tumor Location
In addition to tumor type and grade, tumor location influences the incidence of epilepsy. Tumors located in superficial cortical areas are more likely to be associated with seizures as are as lesions centered in the temporal lobe, frontal lobe, or insula [5, 19–21]. Inherent epileptogenicity of structures in the mesial temporal lobe contributes to seizure generation in this region [22, 23]. Additionally, localization of tumor to eloquent or near-eloquent brain increases risk for seizures [24–26].
Molecular Biological Factors of Seizure Development
Low-grade brain tumors have a stronger predilection for epileptogenesis than more malignant brain cancers. In comparison to higher grade tumors such as glioblastoma, low-grade brain malignancies including gliomas more frequently present with seizures despite being larger in size at the time of discovery [21, 27]. This may be explained by the slow growth rate associated with low-grade lesions which favors development of seizure-prone changes like de-afferentation and disconnection of cortical areas leading to denervation hypersensitivity [28]. This slower rate of growth may also permit adaptive changes of the surrounding brain tissue to occur, thereby diminishing the development of focal neurological deficits.
Brain tumors can also affect the brain network distant to the original site, leading to disruptions in functional connectivity in remote areas [29]. Alterations in microenvironment including hypoxia and acidosis induce swelling and cell damage together with deregulation of sodium and calcium influx with generation of electrical impulses. Overexpression of voltage-gated sodium channels as well as changes in the SV2A synaptic vesicle protein associated with calcium accumulation may facilitate recurring generation of action potentials around tumor cells [30].
Molecular genotypes also contribute to seizures. Solitary 19q loss associated with anaplastic tumors and temporal lobe location [14] has been associated with secondary generalized seizures [31]. The presence of mutations in the mTOR pathway and BRAF V600E mutations as seen in 50% of gangliogliomas increases the risk for seizures [32]. Likewise mutations of codon 132 isocitrate-dehydrogenase 1 (IDH1), which are present in 71–88% of grade II astrocytomas and oligodendrogliomas are associated with seizures [33–36]. The IDH1 enzyme belongs to the Krebs citric acid cycle, catalyzing the conversion of isocitrate into alpha-ketoglutarate. Instead, the enzymatic product of the mutated gene produces 2-hydroxyglutarate. The latter structurally resembles glutamate, which may activate NMDA receptors with ensuing seizure activity. Altered expression of glutamate transporters, including the cysteine-glutamate transporter (xCT) system , increases the concentration of extracellular glutamate, which contributes to epileptic discharge, tumor proliferation, and peripheral excitotoxicity [37, 38]. Furthermore, dysregulation of intracellular chloride promotes glioma cell mitosis and migration, and γ-aminobutyric acid (GABA) signaling suppresses proliferation. In neurons, however, chloride accumulation leads to aberrant depolarization on GABA receptor activation, thereby promoting epileptic activity [26, 38]. Disturbances of chloride balance in gliomas are secondary to changes in chloride cotransporters by reduced KCC2 and increased NKCC1 expression with accompanying changes in GABA metabolism [39]. Glutamergic stimulation of NMDA- and AMPA-receptors activates intracellular mTOR, AKT, and MAPK signaling pathways leading both to cell growth and to epileptogenesis [40, 41].
Seizure Semiology
In about half of cases, the first epileptic event presents as a generalized seizure, while partial seizures predominate thereafter [42]. In low-grade gliomas, seizures most frequently present as secondarily generalized seizures (70%), followed by simple partial seizures (24%), and complex partial (7%) [24]. In glioblastoma, the most frequent presenting seizure semiology is that of secondary generalization in 40% of cases, followed by partial seizures in 37% and both partial and generalized seizures in 14.4% [16, 43]. Status epilepticus is observed in 12% of cases [43].
Factors in Seizure Control
In patients presenting with epilepsy, the number of seizures and their duration prior to surgery are predictive of postoperative seizure behavior [24, 44]. Furthermore, the underlying tumor histology can influence seizure control. DNETs show a postoperative seizure control of 83% compared to 78% in patients with gangliogliomas (Table 9.3) [13, 45].
Table 9.3
Seizure characteristics in patients with neuroepithelial tumors
Glioneuronal | LGGc | GBMd | ||
---|---|---|---|---|
DNETa | Gangliogliomab | |||
Age at presentation (median) | 15 years | 17–21 years | 38 years | 60 years |
Seizures | ||||
At presentation (%) | 100 | 60–95 | 65–85 | 42.1 |
Incidence (%) | 80–90 | 70–90 | 30–60 | |
Type of epilepsy | ||||
Partial (%) | 50.7 | 86 | ||
Simple partial | na | 12% | 23.7% | 20.3–27% |
Complex partial (%) | 52 | 74 | 6.6 | 3.1–10 |
Generalized or mixed (%) | 49.3 | 43 | 69.7 | 35 |
Location of tumor | ||||
Temporal (%) | 79.4 | 35–76 | 37 | 24–27 |
Extra-temporal (%) | 20.6 | 24 | 63 | 73–76 |
Frontal (%) | 13.8 | 7–13 | 71 | 28–43 |
Parietal (%) | 3.4 | 6–10 | 9 | 19–27 |
Insular (%) | na | na | 21 | na |
Occipital (%) | 3.4 | 2.0–3.2 | 0 | 5–9 |
Surgery plays an important role in seizure control . Rates of complete seizure freedom postoperatively range from 45 to 100%. In a systematic review on DNETs and gangliogliomas, 80% of patients with tumor-related epilepsy preoperatively became seizure free after surgery [13, 45–47]. The presence of preoperative partial seizures was associated with 87% seizure freedom after resection, as compared to 73% for generalized seizures. Duration of seizures shorter than one year and extensive tumor resection are both favorable prognostic factors for postoperative seizure control. This suggests that earlier intervention may lead to better control and that residual tumor increases the risk of postoperative seizures [45].
In low-grade gliomas , prognostic factors for good postoperative seizure control include generalized seizures, preoperative seizure control, extra-temporal location, and a gross tumor resection within <1 year after presentation [24, 48, 49]. Overall, seizure freedom is achieved in 74% of patients with generalized or mixed type epilepsy, in 63% with complex partial, and 53% with simple partial epilepsy (Table 9.3) [24]. In oligodendrogliomas, seizure control is linked to tumor control, independent of the presence of seizures as the first clinical sign [50]. AED-resistant seizures are not rare, occurring in 40% of patients.
In GBM, preoperative uncontrolled seizures and parietal lobe involvement are negative prognostic factors. Prolonged seizure control is associated with a better Karnofsky performance score [16].
Seizures in Relation to Survival
Seizure as the presenting symptom of brain tumors is a favorable prognostic factor for survival, as are the number of seizures and duration of preoperative seizures [24, 44].
In low-grade gliomas, seizures without other neurological symptoms at presentation are associated with longer overall survival time (HR: 0.27) [50, 51]. Conversely, neurological deficits or a poor performance status at presentation are associated with worse longevity [49]. Overall, the presence of seizures, smaller tumor volume and extent of surgical resection are all associated with longer survival in low-grade gliomas [11].
In oligodendroglial tumors , uni- and multivariate analysis reveal that the presence of IDH1 mutation, co-deletion of 1p and 19q and frontal tumor location are positive prognostic factors regardless of presence of seizures [14, 52, 53]. Nonetheless, the relation between seizures and survival is remarkable. Patients with a single seizure prior to surgery (14% of all patients) do extraordinarily well, showing a 90% 10 year survival. In contrast, one-third of the small subgroup presenting with other neurological deficits (10% of all patients) die within 1 or 2 years. The remaining patients have either occasional or clearly relapsing seizures, and both groups show a steadily decreasing survival slope with a median survival of 10 years. However, patients with refractory seizures tend to do worse than those with controlled seizures [50].
Nevertheless, seizures as a good prognostic feature seem mainly a secondary phenomenon [38]. In low-grade gliomas, initial epilepsy as favorable prognostic factor could be explained by the association of the IDH1 mutation with formation of D-2HG, which may generate epileptic activity [36, 54]. In glioblastomas, new-onset epilepsy is associated with presentation at younger age, better overall health status and substantially smaller tumor size, which are all independent favorable factors for survival [55, 56].
Seizure Recurrence
Reappearance or worsening of seizures following a relatively long period of seizure control may signal progression or recurrence of glioma. In low-grade gliomas, relapse of seizures after a period of 6 months or more of seizure freedom is an indicator of tumor progression in about 50% of patients [24, 57]. Approximately 15% of glioblastoma patients have ongoing seizure activity despite multiple antitumor regimens [43]. Recurrence or worsening of seizures following a stable period in GBM heralds progression in approximately two-third of patients following first-line antitumor therapy [16, 24, 58]. This necessitates adjustment of seizure treatment and reevaluation of the tumor status of the patient. Thus, while early seizures in gliomas represent a favorable prognostic indicator with respect to survival, late recurring seizures are more likely to indicate progressive disease, often accompanied by adverse effects on cognition and quality of life.
Effects of Antitumor Therapy on Seizure Control
Surgery
Surgical resection is a crucial part of tumor therapy as well as being effective for seizure control. Large series of low-grade gliomas indicate an overall seizure-free rate of 67–87% following surgery [10, 20, 24, 49]. Refractory seizures benefit most from gross tumor surgery. In high-grade gliomas, tumor resection renders 77% of patients seizure-free [16]. For temporal lobe tumors, higher rates of seizure freedom (90–95%) are achieved by using electrocorticography (ECoG) for performing additional hippocampectomy, corticectomy or both as compared to the lower rates (79%) with gross total lesionectomy alone [59]. The most consistent finding in surgical studies of brain tumor-related epilepsy is that gross total resection is associated with greatly improved seizure outcome compared to subtotal resection.
Radiotherapy
Radiation therapy contributes to improved seizure control in low- and high-grade gliomas. A randomized EORTC trial of early versus delayed radiation in low-grade glioma found that 75% of patients became seizure-free following early radiation compared to 59% in the control arm [60]. Retrospective studies in low-grade glioma reveal that 56–77% of patients experience a 50% reduction in seizure frequency with 38–80% becoming seizure-free [61–64]. In a series of combined low- and high-grade gliomas, 77% of patients showed 50% seizure reduction and 38% seizure freedom at 12 months following radiation therapy, although patients were unable to discontinue AEDs [60].
Chemotherapy
The efficacy of chemotherapeutic drugs for treating low-grade gliomas, either as initial treatment or following surgery and radiotherapy, is well established. These agents also contribute to seizure control [44, 65, 66]. Upfront temozolomide chemotherapy in low-grade gliomas is associated with 50% seizure reduction in 50–60% of patients, with 13–55% becoming seizure-free [65, 67, 68]. In recurrent low-grade gliomas, 78% of patients achieve 50% seizure reduction with either temozolomide, PCV chemotherapy or bevacuzimab (in some cases in conjunction with preceding surgery) [65–70].
Symptomatic Treatment with Anticonvulsant Drugs
Symptomatic management of seizures in cancer patient is in principle not different from other causes for focal epilepsy. Antiepileptic drugs can be initiated after the occurrence of a single seizure attributable to a brain tumor [71, 72]. In general, the choice of a specific AED is primarily based on the type of the epilepsy. Epilepsy in patients with brain tumors belongs to the type of partial epilepsy in adults, either with or without secondary generalized seizures, and is essentially based on focal lesion or brain damage. For this type of seizures, the International League against Epilepsy (ILAE) has updated the most appropriate AEDs based on a meta-analysis of large number of randomized controlled trials [73]. As such, levetiracetam (LEV) , carbamazepine , phenytoin , and zonisamide are considered class A anticonvulsants, based on trial quality for efficacy. Valproic acid (VPA) represents the only class B anticonvulsant. Gabapentin, lamotrigine, oxcarbazepine, phenobarbital, topiramate, and vigabatrin are class C agents [73]. Subsequently, the choice of the most fitting AED among the approved agents depends on individual patient factors, most importantly age, sex, weight, comorbidities, and concomitant therapy including drug interactions [74]. Regarding concurrent treatment, in neuro-oncology a consensus exists to avoid enzyme-inducing anti-epileptic drugs (EIAEDs) —phenobarbital, carbamazepine and phenytoin—as these accelerate the metabolism of many chemotherapeutic agents [71, 75]. For zonisamide , there is still limited experience with brain tumors [76]. Table 9.4 provides pharmacological details on anticonvulsant drugs.
Table 9.4
Main characteristics of AEDs (in alphabetical order) including dose range
AED | Usual dosage (mg/day) | Therapeutic range (mg/l) | Common/ important side effects | Main mechanism of action | Oral bioavailability (%) | T 1/2 (h) | Metabolism and excretion | Protein binding (%) |
---|---|---|---|---|---|---|---|---|
CBZ | 400–1600 | 4–12 | Leukopenia, aplastic anemia, hepatotoxicity, hyponatremia, SJS/TEN | Blocks voltage-dependent Na+-channels | 75–85 | 5–26 | Hepatic epoxidation, conjugation | 75 |
CLB | 5–40 | 0.1–0.3 | Sedation, cognitive effects, drowsiness | GABA receptor agonist | 80–90 | 12–60 | Hepatic N-demethylation | 85 |
CZP | 0.5–4 | 0.02–0.08 | Sedation, cognitive effects, drowsiness | GABA receptor agonist | 90 | 20–80 | Hepatic reduction and acetylation | 86 |
FBM | 1200–3600 | 30–100 | Hepatic disturbance, SJS, aplastic anemia, insomnia, weight loss | NMDA and Na+-channel conductance | >90 | 13–30 | Hepatic hydroxylation and conjugation (60%), renal excretion (40%) | 20–25 |
GBP | 900–3600 | 2–20 | Weight gain, worsening of seizures | Blocks Ca+ channels | <65 | 5–7 | Renal excretion without metabolism | None |
LCM | 200–400 | 10–20 | Dizziness, headache, nausea, diplopia, blurred vision | Slow inactivation of voltage-dependent Na+-channels | >95 | 13 | Hepatic demethylation, unchanged renal excretion (40%) | <15 |
LTG | 200–600 | 1–15 | Rash, SJS, TEN, DRESS, headache, blood dyscrasia, ataxia | Blocks voltage-dependent Na+-channels | >95 | 12–60 | Hepatic glucuronidation (without phase 1 reaction), renal excretion (10%) | 55 |
LEV | 1000–3000 | 3–30 | Somnolence, asthenia, irritability, psychosis | binding to synaptic vesicle protein 2 (SV2A) | >95 | 6–8 | Partially hydrolyzed in the blood, renal excretion (66%) | None |
OXC | 900–2400 | 10–35 | Somnolence, headache, diplopia, SJS, hyponatremia | Blocks voltage-dependent Na+-channels | >95 | 8–10 | Hydroxylation, conjugation | 38 |
PB | 30–180 | 15–40 | Rash, hepatotoxicity, impaired cognition, ataxia, mood change | GABA receptor agonist, glutamate antagonist, blocks voltage-dependent Na+-Ca+ channels | 80–100 | 46–136 | Hepatic oxidation, glucosidation, hydroxylation, conjugation | 45–60 |
PHT | 5 mg/kg | 10–20 | Blood dyscrasia, hepatitis, SJS, gum hyperplasia, lupuslike reactions, hirsutism | Blocks voltage-dependent Na+-channels | 95 | 7–42 | Hepatic oxidation, hydroxylation, conjugation | 85–95 |
PGB | 150–600 | 2–8 | Somnolence, dizziness, ataxia | Binds to Ca+-channels | 90 | 6 | No metabolism, renal excretion | None |
PMP | 4–12 | 0.2–1.0 | Dizziness, somnolence, fatigue, irritability | AMPA-receptor blocker | 100 | 24–500 | Hepatic oxidation, glucuronidation | 95 |
TPM | 100–500 | 2–20 | Impaired cognition, hepatotoxicity, weight loss, renal stones | Blocks Na+-channels, GABA receptor agonist, blocks NMDA receptors | 81–95 | 19–25 | Mainly renal excretion without metabolism | 13–17 |
VGB | 200–300 | 0.8–36 | Visual field defects (33%; often irreversible), fatigue, drowsiness | GABA-transaminase inhibitor | 80–90 | 6–8 | No metabolism, renal excretion (70%, unchanged) | None |
VPA | 500–2500 | 50–100 | Hepatotoxicity, thrombo- and neutropenia, aplastic anemia, tremor, weight gain, hair loss, ovarian cystic syndrome | GABA receptor agonist | >95 | 12–15 | Hepatic glucuronidation, oxidation, conjugation | 85–95 |
ZON | 200–600 | 20–30 | Somnolence, ataxia, dizziness, renal calculi | Blocks Na+– and Ca+-channels | >95 | 60–70 | Hepatic acetylation, glucuronidation (20%), renal excretion (30%) | 40–50 |
Monotherapy
Levetiracetam as monotherapy demonstrates a high seizure control rate of 70–100% in low- and high-grade gliomas, although preceding surgery and associated antitumor treatment contribute to these excellent results [43, 77]. Levetiracetam may also be effective with brain metastasis [78].
There are several advantages of levetiracetam over other AEDs, including good tolerability and lack of drug–drug interactions . A comparative trial in the early postoperative period showed a better efficacy and tolerability than phenytoin [79]. However, approximately 5% of patients develop irritability, aggression or psychosis, for which dose adjustment or withdrawal of levetiracetam is usually indicated [80]. Intriguingly, cognition improves in around 25% of patients taking levetiracetam in both general epilepsy and BTE [81–83].
Valproic acid monotherapy in BTE is extensively utilized for low- and high-grade gliomas and provides improved or complete seizure control in 55–78% [16, 43, 58]. Valproic acid is a broad-spectrum, well-tolerated AED although it may cause increased appetite, hand tremor, and thrombocytopenia as side effects. It is contraindicated in pregnant women.
With regard to class C agents, there is little information on gabapentin and lamotrigine in BTE, although both AEDs are generally well-tolerated. Topiramate is a broad-spectrum AED that often causes substantial cognitive side effects. Oxcarbazepine monotherapy in BTE produces complete seizure control in 40–63%, though with a relatively high rate of cognitive adverse effects [84, 85]. Lacosamide is a well-tolerated AED that has recently been approved as monotherapy in the US. With add-on lacosamide in BTE, 43% of patients became seizure-free, and 40–50% of patients showed a 50+% seizure reduction [86, 87]. Adjunctive perampanel in drug-resistant partial seizures produces about a 40% response rate and sustained seizure frequency improvement with a generally favorable safety profile [88, 89].
Polytherapy
When the initial anticonvulsant provides insufficient seizure control, one can switch to a second agent as monotherapy, though there is a trend to rather apply polytherapy as the next step by adding a second AED to the first one [90, 91]. Meta-analysis in drug-resistant epilepsy has indicated that add-on levetiracetam is remarkably effective both in general epilepsy as in BTE, producing 50+% seizure reduction in 65% of patients [43, 77]. This might suggest synergistic qualities of levetiracetam, particularly in combination with AEDs that have GABAergic or antiglutaminergic activity [92]. In neuro-oncology, a crucial advantage of synergistic therapy is that effective lower doses of AEDs carry smaller risks of toxicity, although this requires confirmation in prospective studies.
In BTE, we favor levetiracetam as the anticonvulsant of choice, if necessary to be combined with valproic acid , as both agents are well tolerated in the partial epilepsies [93, 94]. A good alternative anticonvulsant is lacosamide based on efficacy, good tolerability, and absence of drug interactions [87, 95, 96]. In glioblastoma, VPA may be given as first line AED in based on the evidence as class B agent for focal epilepsy together with emerging activity of antitumor efficacy if combined with temozolomide [43, 97–99]. If either levetiracetam or combinations with VPA or lacosamide are insufficiently effective, one can choose lamotrigine for its good tolerability and its potential of synergistic activity with VPA, perampanel for its efficacy and tolerability in refractory partial epilepsy, or zonisamide considering its recent designation as class A agent for the partial epilepsies [73, 88, 89, 100]. For the application of anticonvulsant therapy in daily clinical practice of BTE, see Table 9.4.
Duration of AED therapy depends on the underlying cause of seizures. For seizures associated with metabolic or toxic encephalopathies, we recommend continuing AEDs therapy as long as the underlying cause remains present or can easily recur. In the case of a structural lesion as cause of the epilepsy as with gliomas, we advocate continuing antiepileptic treatment for at least 2 years following seizure control [101, 102]. In patients with long-term good seizure control on polytherapy, it is reasonable to try to gradually taper the patient down to monotherapy.
For treatment recommendations in children, we refer to other reviews [103].
Prophylactic AED Use
It is uncertain whether there is any role for AED prophylaxis in brain tumor patients without a history of seizures. Consensus statements by the American Academy of Neurology and the European Association of Neuro-Oncology (EANO) have advised against long-term AED prophylaxis in newly diagnosed brain tumor patients [71, 75]. Meta-analysis of available studies has not provided evidence in support of AED prophylaxis [104]. A notable exception is that prophylactic AEDs may be considered for the first weeks after surgical resection, given the frequency of immediate postoperative seizures [105–107]. In a study on 121 patients undergoing glioma surgery, despite receiving prophylaxis, 9.1% of patients experienced a seizure within the first postoperative week, and problems with AED tolerability were not uncommon [108]. There is borderline evidence for AED prophylaxis in the peri-operative period [107].
Toxicity
Cognitive Function and AEDs
Overall, AED therapy—and particularly polytherapy—lead to a deterioration of cognitive functioning [109–111]. In a prospective cross-sectional survey in 147 patients with BTE, 54.4% showed cognitive impairment. On multivariate analysis, these impairments were associated with advancing age, lesion laterality, and chemotherapy use [112]. In a group of 156 long-time survivors of low-grade glioma, both the presence of seizures as well as therapy with conventional AEDs correlated with cognitive deterioration on several domains [113, 114]. In another prospective study, the presence of low-grade gliomas, prior radiation therapy (particularly >2 Gy per fraction), and anticonvulsant use were associated with slower psychomotor speed, memory deficits, and attention or executive deficits [115].
In high-grade glioma, data are less convincing that AED use leads to impaired memory [116, 117]. Chemoradiation leads to a diminished brain volume, and in that way patients are probably more prone to factors that lead to cognitive dysfunction [118].
AED polytherapy strongly contributes to cognitive dysfunction. Each added anticonvulsant leads to a further deterioration of cognitive functioning [111]. These effects are enhanced in patients who already have deficits from primary or metastatic brain tumors, brain surgery, and brain tumor therapy [119, 120].
These observations illustrate that in patients with brain tumors, neuropsychological deficits depend on the cumulative effects of previous neurosurgery, radiation therapy, chemotherapy, presence of epilepsy, and AED use [121]. Although the cognitive adverse effects of AEDs are usually mild or moderate, their impact in BTE can be substantial.
In the setting of AED side effects, options include lowering the AED dose or switching to another agent. Importantly, many patients with epilepsy, if forced to choose, would prefer to be free of AED side effects over having complete seizure control [122]. Consequently, a prudent dose reduction is often justified, particularly with partial seizures without loss of consciousness where complete seizure control is not mandatory [123].
Bone Marrow and AEDs
Bone marrow abnormalities may occur as a side effect of several AEDs, and are relatively more common in brain tumors than other epilepsy etiologies [75].
Although hematotoxicity can arise from phenobarbital, phenytoin, carbamazepine, or VPA, it is more common and severe with concurrent use of temozolomide or PCV [42, 124–126].
Aplastic anemia is a rare, though severe complication of AEDs. Its overall incidence is two to six cases in one million, of which about 20% drug-induced [127, 128]. Among AEDs, felbamate is the most frequently implicated with an incidence of 1 in 5000–10,000; for that reason it is rarely utilized. Rare cases have been associated with carbamazepine, lamotrigine, and phenytoin [129]. The odds ratio of inducing aplastic anemia compared to no AED at all is 10.3 for carbamazepine, 3.5 for phenytoin, and 18.2 for VPA [130].
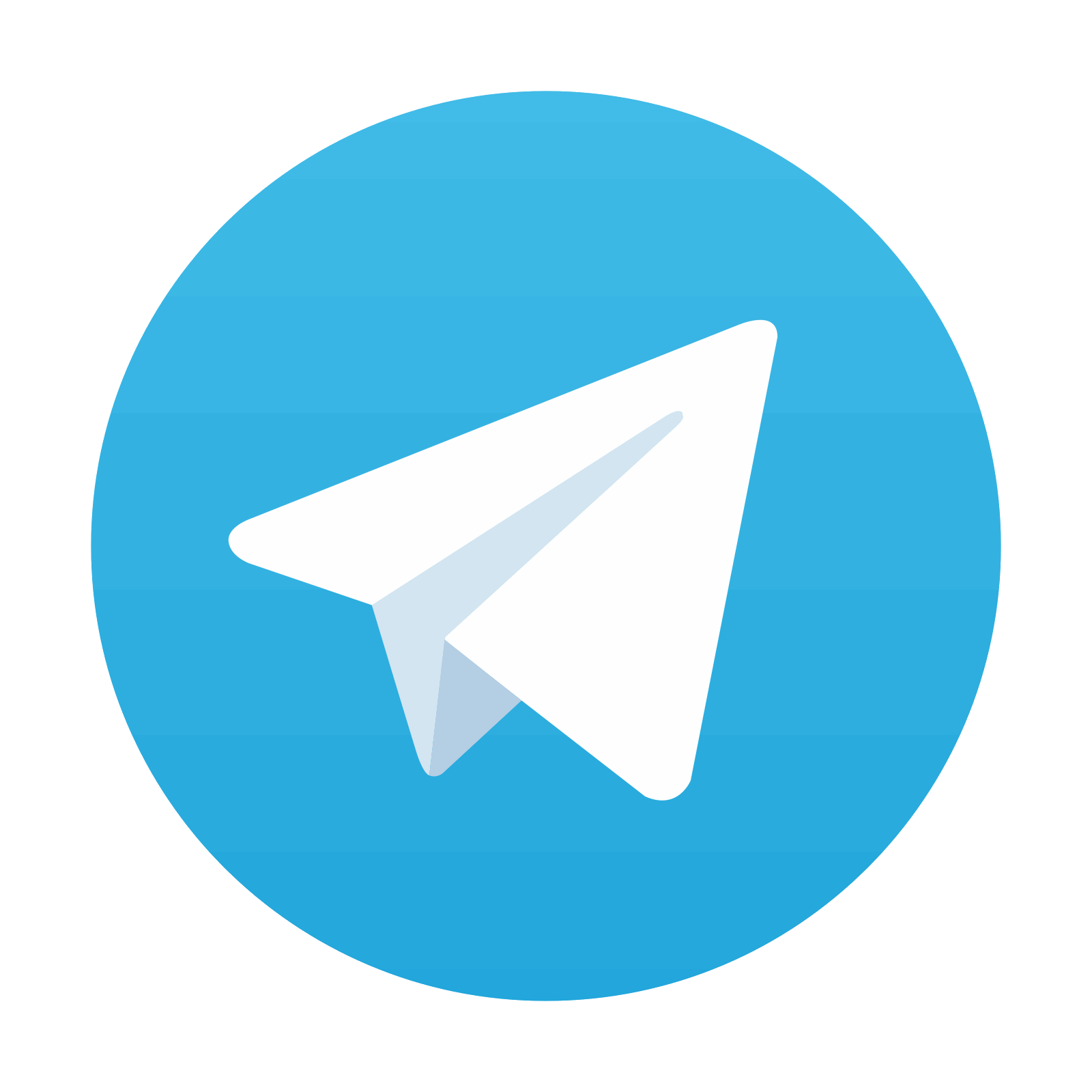
Stay updated, free articles. Join our Telegram channel

Full access? Get Clinical Tree
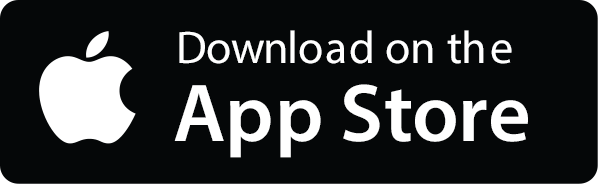
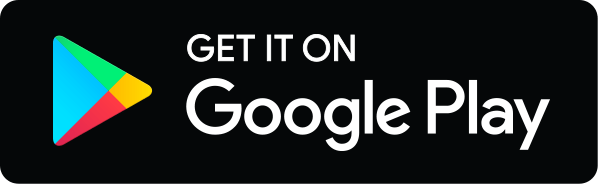