Fig. 2.1
A patient with late poststroke seizures; EEG (a) shows seizure pattern in left fronto-central region, onset at C3–F3 > f7–Fp1. Onset consisted of rhythmic alpha activity at F3–C3, then involved Fp1–F7, to evolve into rhythmic theta, then sharply contoured delta; seizure lasted 30–60 s. This patient suffered 18 seizures over 9 h. MRI (b) shows a focal encephalomalacia in the left posterior temporal and parietal lobes compatible with remote ischemic infarction
Imaging
Five to thirty percent of cases identified as brain attacks may be due to stroke-mimicking conditions [23]. Winkler et al. found that of 250 consecutive patients treated with intravenous thrombolysis, 2.8 % were stroke mimics; none of whom were harmed by thrombolysis. Seizure was the most frequent diagnosis in these patients (85.7 %), with global aphasia without hemiparesis presenting ten times more frequently in the mimic than in the stroke group [33]. The clinical significance of advanced neuroimaging studies and EEG to distinguish stroke mimics from strokes before intravenous thrombolysis is unclear [33]. Furthermore, seizures may produce radiological changes that may make it difficult for a clinician to distinguish a seizure at the onset of an ischemic stroke from a seizure with postictal paresis, known as Todd’s paresis. Seizure activity may lead to metabolic exhaustion of neurons within the epileptogenic focus, producing clinical paralysis. Increased regional permeability in the blood–brain barrier and edema at this site may subsequently be visualized by neuroimaging. An MRI of the brain may demonstrate focal cortical swelling, signal change in fluid-attenuated inversion recovery sequences, and cortical blush when contrast is administered [34]. Seizure duration may correlate with the intensity and size of these changes. Angiography after seizure may demonstrate a focal area of enhanced blood flow secondary to disruption of the blood–brain barrier [35]. Cerebral perfusion imaging may also demonstrate large areas of ictal hyperperfusion or postictal hypoperfusion. When this is observed without corresponding arterial pathology, it may be more indicative of a seizure than acute stroke (Fig. 2.2) [36, 37]. Computed tomography (CT) perfusion brain imaging has recently gained popularity as a method for evaluating penumbral tissue. Masterson et al. reported the CT perfusion changes in four patients presenting with stroke symptoms who were subsequently diagnosed with status epilepticus. Focal cortical hyperperfusion was demonstrated in all patients, with approximately 50 % increase in cerebral blood flow, 30–40 % increase in cerebral blood volume, and 40 % decrease in mean transit time. These findings were attributed to the peri-ictal phases of status epilepticus and are the opposite of perfusion changes found in acute stroke. The authors cautioned that migraines or strokes with reperfusion can produce similar results [32]. Table 2.1 demonstrates the common CT perfusion changes in ischemic infarction, penumbral tissue, and seizures .


Fig. 2.2
a–b Periodic lateralizing epileptiform discharges (PLEDs) in a patient with acute ischemic stroke. EEG (a) shows intermittent PLEDs in the left parietal-central region, maximum P3 > C3, approximately 20 % of the record, lasting 3–10 s in a patient with acute ischemic stroke of the left hemisphere. MRI (b) shows a diffusion restriction in the left hemisphere in the distribution of the middle cerebral artery with mass effect and midline shift in an 83-year-old man who suffered acute-onset aphasia and right hemiplegia
Table 2.1
Cerebral CT perfusion changes in acute ischemic stroke and seizure
Perfusion changes | Penumbral tissue | Core infarct | Seizure |
---|---|---|---|
Cerebral blood flow (CBF) | ↓ | ↓ | ↑ |
Cerebral blood volume (CBV) | Normal or ↑a | ↓ | ↑ |
Mean transit time (MTT) | ↑ | ↑ | ↓ |
Time to peak (TTP) | ↑ | ↑ | ↓ |
Electroclinical Manifestations of Acute Stroke
Acute ischemic stroke may have a number of EEG patterns, of which periodic lateralized epileptiform discharges (PLEDs) are of particular significance. PLEDs are electrographic phenomena characterized by widely distributed, polymorphic, and repetitive complexes of approximately 0.5–3 Hz, having one or more sharp components, present over one or more hemispheres (Fig. 2.3a) [38]. PLEDs may be an expression of dynamic brain damage in the very acute stage. While PLEDs are commonly associated with large cortical destructive processes, they may be seen in patients with subcortical, chronic lesions, or metabolic disturbances [32, 39]. Mecarelli et al. analyzed the EEGs performed within 24 h of a cohort of 232 patients admitted with acute ischemic or intraparenchymal hemorrhagic stroke. Focal or diffuse slowing of background was found in 84 % of patients, epileptiform focal abnormalities in 10 %, and PLEDs in 6 %. Three out of the 23 patients with epileptiform abnormalities developed isolated partial motor seizures without secondary generalization, while none of the patients with slowing had seizures. Of the 14 patients with PLEDs, 10 had focal status epilepticus (9 convulsive, 1 nonconvulsive), and 2 had focal motor seizures. Multivariate analysis demonstrated that only early epileptic manifestations were independently associated with PLEDs [39].Various theories regarding the neurophysiology of PLEDs have been suggested. Some authors have proposed that cortical PLEDs are produced from a large external zone of hyperexcitability, which generates synchronous discharges that communicate with subcortical structures, where they are modulated and returned via corticopetal connections. In addition, subcortical lesions disrupt the basal ganglia–thalamus–cortex network, causing oscillations to be propagated through the thalamocortical projections and eventually widespread areas of cortex [38]. An EEG maybe normal in 5 % of cases, and therefore, a normal EEG does not exclude epileptogenic lesions [40]. Acute ischemia may produce EEG changes within minutes of onset, providing real-time and dynamic information of the neurophysiologic state. Progressive reductions in cerebral blood flow and degree of neuronal injury lead to changes in EEG characteristics, from loss of fast beta frequencies in reversible mild ischemia, to suppression of all frequencies, or isoelectric EEG activity in neuronal death [41].


Fig. 2.3
CT perfusion analysis showing the cerebral blood volume (CBV), cerebral blood flow (CBF), and mean transit time (MTT) of a patient with ischemic penumbra in the right hemisphere due to a hyperacute occlusion of the right middle cerebral artery (a CBV, b CBF, and c MTT), and a second patient with a seizure emanating from the left hemisphere (d CBV, e CBF, and f MTT) followed by a complete resolution of the hyperperfusion (g CBV, h CBF, and i MTT)
Treatment
Treatment and recurrence of seizures of varying etiology other than stroke have been studied in depth. Berg and Shinnar used a meta-analysis to study the issue of recurrence after a first unprovoked seizure. Among 16 reports, the average risk of a first unprovoked seizure was 51 %. At 2 years following the first seizure, the risk of recurrence was 36 % in prospective studies and 47 % in retrospective studies. Recurrence risk varied depending on a number of factors, such as seizure etiology, seizure type, and electrographic findings. The recurrence risk was as low as 24 % and as high as 65 % [42].
The development of poststroke epilepsy occurs in 54–66 % of those who experience late seizures, and less than 43 % in early-seizure patients. The risk of developing a second seizure is similar to the nonstroke patient who experiences a first unprovoked seizure. In these non-stroke patients, initiating AED treatment only after a recurrent seizure does not appear to be harmful [43]. Furthermore, indiscriminate treatment of a first unprovoked seizure without regard to patient’s EEG characteristics, the presence of structural lesion, or risk–benefit profile is not recommended [27, 44]. Similarly, the use of prophylactic AEDs in acute traumatic brain injury without an initial seizure is not recommended because it has been found to be ineffective in reducing mortality, functional outcome, and eventual development of epilepsy, even if it potentially reduces the likelihood of early seizures [3].
However, strokes produce a structural lesion and some authors have favored initiating AEDs after an early poststroke seizure, while others have recommended treatment only after a late seizure [1]. Labovitz and Hauser et al. suggested that the risk of epilepsy in some patients with a single-poststroke seizure is high enough to justify the initiation of AEDs before the second seizure [40, 45]. Others have suggested treating an early seizure for 1 month, then stopping the AED if seizures have not recurred during this period [40]. One approach is to initiate one AED at standard dosage if a patient has developed immediate poststroke seizure. Therapy would be continued for 1–3 months during the time of highest risk for recurrence and discontinued if no further seizures had occurred. A more conservative approach is to attempt discontinuation of AEDs only after 1 year of seizure freedom; an approach facilitated in part by the emergence of newer-generation AEDs that have more tolerable adverse effect profiles and little to no drug–drug interactions. Recurrent seizures while on AED therapy may be milder in severity and type (focal instead of secondarily generalized). Because late seizures carry higher risk of recurrence, AED therapy is recommended.
No single AED has been shown in studies to be clearly and consistently superior to another in the treatment of early seizures or epilepsy after stroke [36]. Therefore, when choosing among the different AEDs, the clinician must take into account the potential side effects of the drug, its pharmacokinetic profile, interaction with other medications, and the potential influence on the neurologic recovery process. Phenytoin, phenobarbital, and carbamazepine are hepatic enzyme inducers, while valproic acid is an enzyme inhibitor. Phenytoin and valproic acid are highly protein bound, and the former also interferes with vitamin K metabolism. These properties of older-generation AEDs may lead to difficulties in maintaining a therapeutic drug range when a patient is concurrently on warfarin. Salicylates may displace valproate from its plasma protein binding sites, leading to reduction in total plasma level. Newer-generation AEDs do not demonstrate significant interactions with warfarin or antiplatelet agents [3]. For example, levetiracetam and lamotrigine lack hepatic metabolism and have a favorable pharmacokinetic profile in terms of drug interaction , compared to older AED [35, 46]. Animal models and clinical studies suggested that older AEDs may impair the recovery after stroke. Phenytoin, phenobarbital, and benzodiazepines have been shown to impair the motor and behavioral recovery process in brain-injured rats and functional recovery in patients [15]. In contrast, vigabatrin and carbamazepine have not demonstrated negative effects on poststroke outcome [47].
Tolerability of the drug remains an important issue to consider when initiating AEDs. Studies comparing various AEDs have suggested better tolerability and retention rates for lamotrigine, gabapentin, or levetiracetam when compared with carbamazepine, a medication known to be effective in poststroke seizure [3, 46]. The long-term tolerability and efficacy of gabapentin was studied in patients who had their first seizure at least 2 weeks after stroke: 81.7 % of the cohort remained seizure-free at a mean follow-up of 30 months. Side effects were reported in 38 %, but were mild to moderate with only 2.8 % withdrawing from the study as a result. The authors concluded that gabapentin was safe and useful for poststroke seizures [48]. Other authors randomized 106 patients with late poststroke seizures to levetiracetam or sustained-release carbamazepine [35]. No statistically significant difference in the number of seizure-free patients was found between the two groups. At the 52-week follow-up, 94 % of levetiracetam and 85 % of carbamazepine were seizure free. Attention deficit, frontal executive function, and functional scales were significantly worse in the carbamazepine group. Premature discontinuation due to serious adverse effects was not statistically significant between the groups [35]. In a smaller study, 64 patients presenting with the first (early or late) poststroke seizure received lamotrigine or carbamazepine in a randomized 1:1 ratio. After 12-month follow-up, significant differences between the two groups were found in terms of efficacy, side effects, and tolerability in favor of the lamotrigine. The number of patients remaining seizure free was found to be 72 % in the lamotrigine group and 44 % in the carbamazepine group. Three percent of patients in the lamotrigine group dropped out because of adverse events, compared to 31 % in the carbamazepine group [46].
Prognosis
Animal stroke models suggest that early seizures may be associated with increased infarct volume; however, it is unclear if early seizures have deleterious effects on the eventual functional outcome after stroke. A significant number of studies found worse outcomes in patients with late or recurrent seizures after stroke, but others failed to demonstrate this effect. The differences in these outcomes may be due to the lack of accounting for confounding factors such as stroke severity, stroke location, or AED treatment at the time of assessment, which has been shown to independently contribute to worse functional outcome with certain drugs [3, 15]. Previous studies have demonstrated increased hospital mortality rates among patients with early seizures after stroke. These studies did not account for stroke severity or rule out seizures as an independent predictor of poor outcome. It has been proposed that seizures maybe a sign of severe brain injury rather than a predictor of poor recovery [11, 49]. It is unclear if seizures independently alter functional outcome after stroke. SASS demonstrated a worse neurological score during initial hospitalization and worse Rankin scale at 9 months of follow-up in patient who had seizures after stroke; however, SASS failed to correct for stroke severity and did not analyze the role of early versus late seizures [3, 50]. Seizures do not influence the rehabilitation outcome in poststroke patients [10, 50]. Prospective studies which did account for stroke severity found no association between early seizures and outcome or mortality [7].
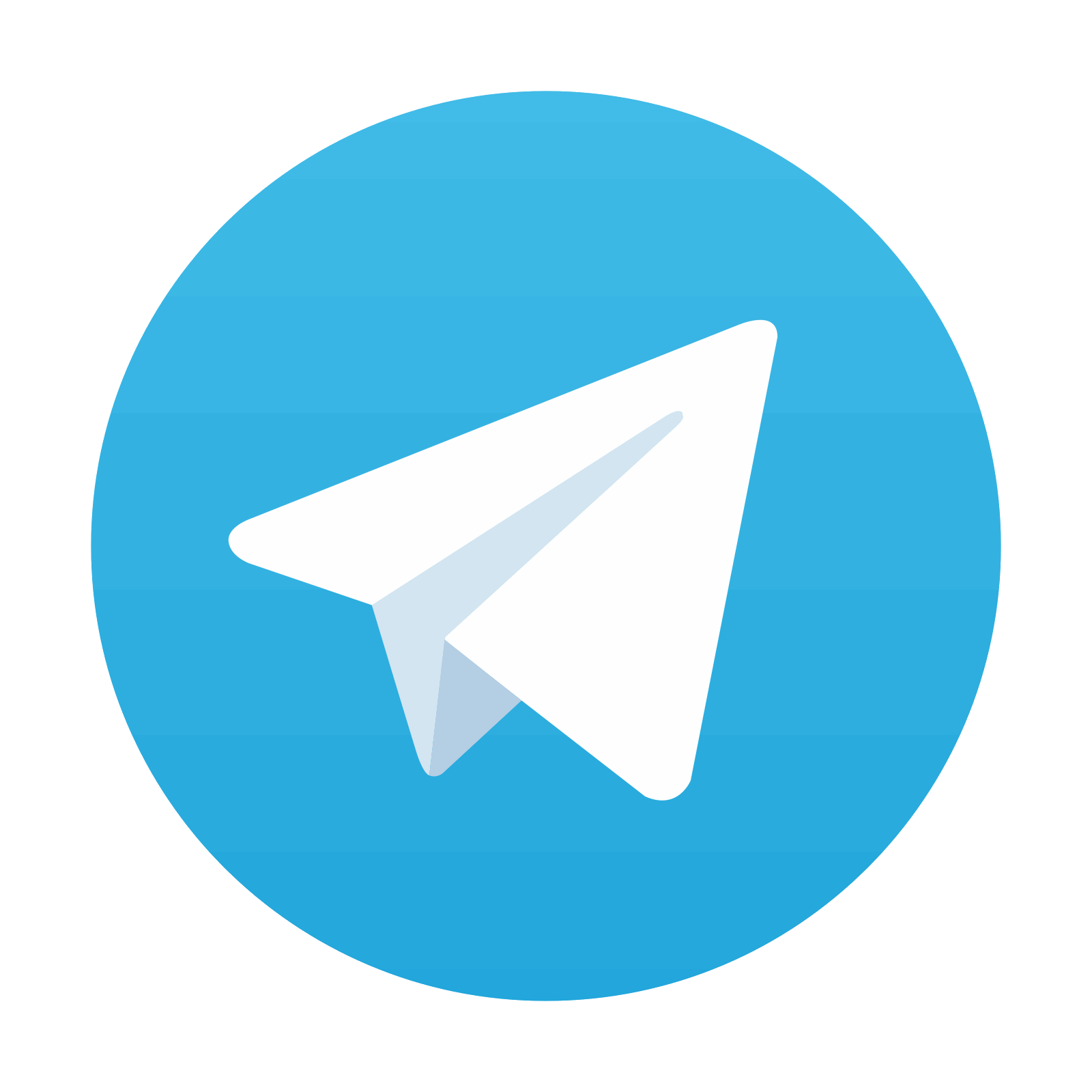
Stay updated, free articles. Join our Telegram channel

Full access? Get Clinical Tree
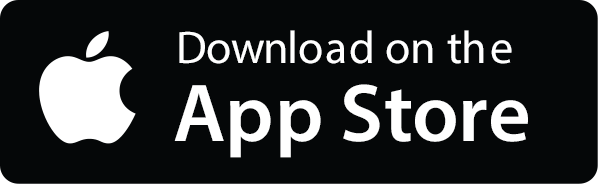
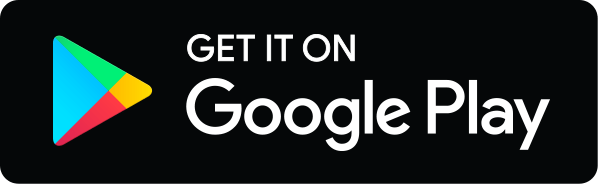