A 45-year-old man is admitted to the intensive care unit (ICU) for head trauma related to a fall on the stairs after acute alcohol intoxication. He is found by neighbors lying on the ground and unconscious, with no idea on the delay since the fall. When the ambulance arrives on scene, the Glasgow Coma Scale (GCS) is 8/15, and the man is intubated. On admission to the emergency department (ED) his blood pressure (BP) is 150/90 mm Hg, heart rate 86 bpm, and temperature 35.9°C. His pupils are symmetric (2.5 mm) and reflective, and there is no obvious sign of lateralization. The patient localizes to pain, does not obey orders, and does not open his eyes. A CT scan performed at ED admission discloses several petechial lesions and moderate edema. The day after admission, the patient becomes febrile (temperature 38.6°C) with no obvious sign of infection. Neurologic examination is deteriorating, with the patient being unable to localizing pain (E1M4V1). A repeated CT scan shows an increase in the cerebral contusions but no increase in edema and no mass effect. Transcranial Doppler ultrasound does not show signs of intracranial hypertension. The neurosurgeon does not consider implantation of intracranial pressure (ICP) monitoring at this stage. During the night the patient develops progressive hypoxemia with a Pao2/Fio2, ratio of 180, hypotension (BP 85/40 mm Hg), tachycardia, fever (temperature 39.5°C), and oliguria (urine output 0.3 mL/kg/h at time of assessment). Clinical examination reveals mottled skin mostly around the knees, no heat murmur, and a few crackles predominant at the right lower lobe. The abdominal examination is without specificities. An arterial blood gas reveals pH at 7.33, Paco2 42, Pao2 72 mm Hg, Sao2 95%, hemoglobin concentration 8.6 g/dL, and lactate 2.9 mmol/L.
Sepsis is a major health problem, representing close to 10% of admissions in the ICU. Mortality is still quite high, depending on the criteria used to define it, but remains around 30% for sepsis without shock and 40% to 50% for septic shock. The definition of sepsis has been revised,1 but it can be defined as a dysregulated host response associated with organ dysfunction that is frequently observed in critically ill patients. Of note, the presence of systemic inflammation response (SIRS) criteria is not mandated, because up to 1 patient in every 12 patients presenting with sepsis can be missed when SIRS criteria are mandated.2
Shock is defined as a life-threatening, generalized form of acute circulatory failure, associated with inadequate oxygen utilization by the cells.3 Arterial hypotension (defined as systolic BP < 90 mm Hg, or mean arterial pressure [MAP] < 65 mm Hg, or a decrease by at least 40 mm Hg from baseline), although commonly present, should not be required to define shock.3
Hypotension was present in this patient, with a systolic arterial pressure < 90 mm Hg and a MAP at 55 mm Hg suggesting circulatory failure. However, hypotension without signs of tissue hypoperfusion is insufficient to qualify for septic shock. First, we should exclude some degree of hypovolemia and the impact of sedation. If hypotension persists after a fluid loading of 30 mL/kg of crystalloids, vasopressors should be used. A patient with sepsis treated with vasopressor agents and presenting signs of hypoperfusion qualifies for the definition of septic shock.
In addition to hypotension, we have three important clinical windows that can be used to evaluate the impact of tissue hypoperfusion on organ function: the brain, the skin, and the kidneys.3,4
Brain dysfunction is often characterized by confusion, agitation, or lethargy. Coma is uncommon in these patients. In neurologic patients, this window is particularly difficult to appreciate because several confounders, including the use of sedative agents or pre-existing neurologic abnormalities, may co-exist. In this patient, it is difficult to ascribe the alteration in mental state to septic shock, because the patient is febrile and fever can markedly alter mental state in the neurologic patient. In addition, the CT scan showed some worsening, which can also contribute to the alteration in mental state.
Skin mottling was effectively present in this patient and was highly suggestive of circulatory failure. Skin mottling can mostly be detected at the level of elbows and knees, but can be more diffuse in the most severe cases. The extension of mottling is associated with a poor outcome.5 Even though a quantitative assessment can be performed (mottling score5), it is seldom performed at bedside because it does not provide major information compared with subjective assessment (only differences between the extremes were associated with difference in outcome and of course, these can easily be assessed without scoring). Capillary refill time can also be informative. After pressure on a digital extremity, recoloration usually occurs within < 2 seconds. A capillary refill time about 4 seconds is considered as prolonged and is a sign of tissue hypoperfusion. In opposition to skin mottling which also occurs during high-output states, a decrease in capillary refill time occurs only in low-output states.
A decrease in urine output is an excellent marker of renal hypoperfusion. A low urine output is defined as < 0.5 mL/kg/h. If this sign takes some time to be detected in the ED (at least 1 hour is needed after insertion of bladder catheter), it is very easily detected in hospitalized patients equipped with a bladder catheter.
In addition to these clinical signs, biologic signs can be used. In particular, blood lactate levels are important indices of tissue hypoperfusion. In normal conditions, glucose is transformed into pyruvate, generating 2 molecules of ATP. Pyruvate thereafter enters the mitochondria and then the Krebs cycle, forming H2O and CO2 and generating 36 molecules of adenosine triphosphate (ATP). Some moderate amount of pyruvate is transformed into lactate so that a normal lactate level is 1.0 mmol/L. In anaerobic conditions, pyruvate cannot enter the mitochondria and is transformed into lactate. Therefore, lactate levels increase, usually above 2 mmol/L. The production of ATP is of course minimal in anaerobiosis, but this helps the cells to survive for a couple of hours. Admittedly, this mechanism alone is insufficient to ensure cell survival. Another important adaptive mechanism is O2 conformance, by which cells shut down all nonessential metabolic reactions (eg, reproduction but also most reactions characterizing the function of the organs, such as contraction for muscle cells and solute transport for epithelial cells) and just keeping active the reactions mandatory for cell survival (mostly membrane integrity). Even though severe metabolic acidosis is often the hallmark of tissue dysoxia, lactate and proton generation are dissociated. Metabolic acidosis is produced by generations of H+ during hydrolysis of ATP and the absence of use of these protons at the level of the cytochrome in the absence of oxygen. Of note, lactate can also be produced in aerobic conditions, mostly by acceleration of aerobic glycolysis in response to an activation of Na+/K+-ATPase under the influence of inflammatory molecules and catecholamines. The interpretation of lactate levels is not always easy, but at early stages hyperlactatemia is mostly of hypoxic origin.6 Hyperlactatemia is associated with a poor outcome, even when relatively mild.7,8 Hence, lactate can be used as a marker of the severity of shock.
Other biologic signs of tissue hypoperfusion (such as an increased liver enzyme concentration and an increased creatinine level) may take some time to develop and thus cannot be used for screening. These variables can later be of interest when assessing the severity of organ dysfunction.
This aspect is too broad to be covered in this chapter. Briefly, sepsis is the consequence of an initially appropriate response to an infection that becomes out of control by amplification by damage-associated molecular patterns (termed, DAMPS). This results in a dysregulated inflammatory but also an anti-inflammatory response to an infection that is associated with activation of the coagulation and diffuse endothelial damage. The balance between the different components (pro- and anti-inflammatory molecules) is not easily determined by clinical phenotype. In addition, multiple sometimes redundant pathways are implicated. Altogether, these factors may explain why multiple sepsis-targeted therapies (eg, steroids, anti-endotoxin antibodies, anti–tumor necrosis factor, or anti- IL-6 antibodies) have failed to improve outcome. Whether this pathway is completely blocked or whether these agents may benefit from individualized therapies remains to be determined.
What is the pathophysiology of septic shock, and why did this patient develop organ dysfunction?
The pathophysiology of sepsis and septic shock is quite complex. Septic shock associates a combination of hypovolemia, decreased vascular tone, myocardial depression, regional blood flow alterations, and microcirculatory alterations. These result in an impaired tissue perfusion, which can be disclosed by increased lactate levels and increased veno-arterial Pco2 (partial pressure of oxygen) gradient.
Hypovolemia is a common finding in sepsis and results from fluid shifts into the intracellular space due to increased microvascular permeability and from blood pooling in large-capacitance veins.
In septic shock vascular tone is reduced because of altered vascular reactivity to adrenergic and nonadrenergic vasopressor substances, and this factor results in hypotension but also in alterations in blood flow distribution.
Sepsis in patients without primary brain injury is often associated with mild cerebral dysfunction, but the neurologic deterioration that this patient presented is out of proportion.
Several mechanisms may have contributed to this worsening. In this patient, we should not neglect the potential impact of fever on intracranial pressure. Even though ICP was not directly measured, this mechanism seems unlikely as indirect measurements such as CT scan and transcranial Doppler ultrasound did not suggest increased ICP.
Sepsis can directly affect brain perfusion by two different mechanisms. First autoregulation may be altered. In normal conditions, brain circulation remains unchanged in the face of changes in BP and cardiac output. In septic conditions, autoregulation to BP can be lost in some patients, especially when hypercapnia is present.9 More exceptional, brain perfusion may also vary in proportion to cardiac output.10-12 Accordingly, the septic brain may be more sensitive to alterations in BP or in cardiac output. Second, microvascular perfusion is altered in sepsis. It is quite difficult to demonstrate in humans, but in relevant models of sepsis in large animals, brain microcirculation was shown to be altered.13,14 It remains to be determined to what extent these microcirculatory alterations remain accessible to therapeutic interventions or whether these make the brain more sensitive to altered systemic hemodynamics (Figures 53-1 and 53-2).
Figure 53-1.
The normal brain microcirculation. Image of the microcirculation obtained in a healthy anesthetized sheep. This image was obtained applying a Sidestream Dark Field imaging technique to the brain through a craniectomy. The white arrow shows a venule, and the black arrow a capillary. Note the rich network of capillaries.

Figure 53-2.
Image of the microcirculation obtained in an anesthetized septic sheep using the same technique as in Figure 53-1. The arrow shows an area almost deprived of capillaries. Several spots deprived of perfused capillaries are in close vicinity to well-perfused areas, indicating a huge heterogeneity of perfusion, a typical hallmark of distributive shock. Note that the density of perfused venules was not affected.

Because of the severity of the disease, we need to provide diagnosis procedures, source control, and organ support simultaneously rather than sequentially (Table 53-1). For the easiness of the comprehension, these are discussed below.
Management | Action | Comment |
---|---|---|
Recognize the presence of an infection | Fever or hypothermia Leukocytosis or leucopenia Tachycardia Biomarkers (eg, C-reactive protein and procalcitonin) | |
Recognize sepsis and its severity | Tachycardia Tachypnea Hypotension Altered mental state Mottled skin Oliguria (< 0.5 mL/kg/h) Blood lactate levels (≥ 2 mmol/L) | In neurologic patients, alteration in mental state is often difficult to ascribe specifically to sepsis. |
Identify the source of infection | Obtain blood cultures before antibiotics Sputum Urinary Cerebrospinal fluid Remove catheter (and culture their tips) Other specimens if available | Ideally, all samples should be obtained before antibiotic administration. However, these should not delay antibiotic administration so that antibiotics should be started if these cannot be obtained immediately. |
Infection control | Administer broad spectrum antibiotics within one hour of hypotension/recognition of sepsis Source control if possible | Antibiotics adapted to the potential organism (out of hospital vs nosocomial vs other facilities), location of infection (brain vs other sites). De-escalate as soon as possible when the responsible strain has been identified. Short durations of antibiotics are often equivalent to longer durations. |
Hemodynamic and organ support (see Tables 53-2,53-3,53-4) |
Neurologic patients often develop sepsis and septic shock during their hospital stay (see Chapter 56, Common Infections in the ICU). This aspect will be covered in more detail in another chapter. In general ICU patients, sepsis is mostly of respiratory origin (35%), followed by abdomen, urinary tract, and skin, as well as catheters and miscellaneous sources. The origin of sepsis cannot be determined in up to 10% of the cases. In neurosurgical patients, lungs, urinary tract and vascular (or ventricular) lines, and skin are the most common sources of infection, but other sources should never be neglected (ie, the abdomen, caused by perforated ulcus or acalculous cholecystitis), and a complete workup should always be performed. In our patient, the chest radiograph demonstrated bilateral lung infiltration with condensation of the right lower lobe associated with air bronchogram that is suggestive of a right lower lobe pneumonia.
This aspect will be detailed in more details in Chapter 54, Antimicrobial Therapies in the ICU. Nevertheless it is important to recognize that there is a tremendous difference between an infection without sepsis and an infection associated with sepsis. In patients with sepsis, and especially septic shock, the time between the onset of hypotension and adequate antibiotic administration should be minimized. Experimental studies have shown that a decrease by ≥ 6 hours is associated with a huge increase in mortality.15 Several trials including a total of 40,000 patients with septic shock have indicated that antibiotics should ideally be administered within hours of the onset of hypotension, each hour of delay being associated with a further increase in mortality.16-18 In addition, source control by removal of infected lines, or if necessary surgery or percutaneous drainage, should also be considered.
As adequate antibiotic therapy should be considered, it is of course important to consider the potential source of infection and the previous exposure to antibiotics. This patient was considered to have aspiration pneumonia. Because the delay since hospital admission was less than 3 days and he has not been exposed recently to antibiotics, amoxicillin-clavulanate was administered.
Of note, biologic blood samples should also be obtained to determine the strain responsible for the infection. Recovery of these samples should not delay antibiotic administration significantly. Blood cultures should be obtained immediately as well as sputum, if possible. Urine and CSF results may be delayed and are sometimes completed after antibiotic administration.
In this patient, sputum was positive for Streptococcus pneumonia and Haemophilus influenza; blood cultures were positive for S pneumonia. However, results for urine and catheter tests were negative. Antibiotics were administered for a total of 7 days, with good evolution of the chest radiograph and a marked decrease in C-reactive protein.
The hemodynamic management of the patient with septic shock aims at avoidance of tissue hypoperfusion. Four phases of hemodynamic management can be identified:4 salvage in which life-saving measures are performed; optimization in which the goal is to provide optimal oxygen delivery to the tissues by optimizing cardiac output, systemic venous oxygen content (Svo2), and lactate; stabilization in which we try to minimize complications but otherwise do not try to push the cardiovascular system; and de-escalation in which vasopressors are weaned and a negative fluid balance is achieved (Table 53-2).
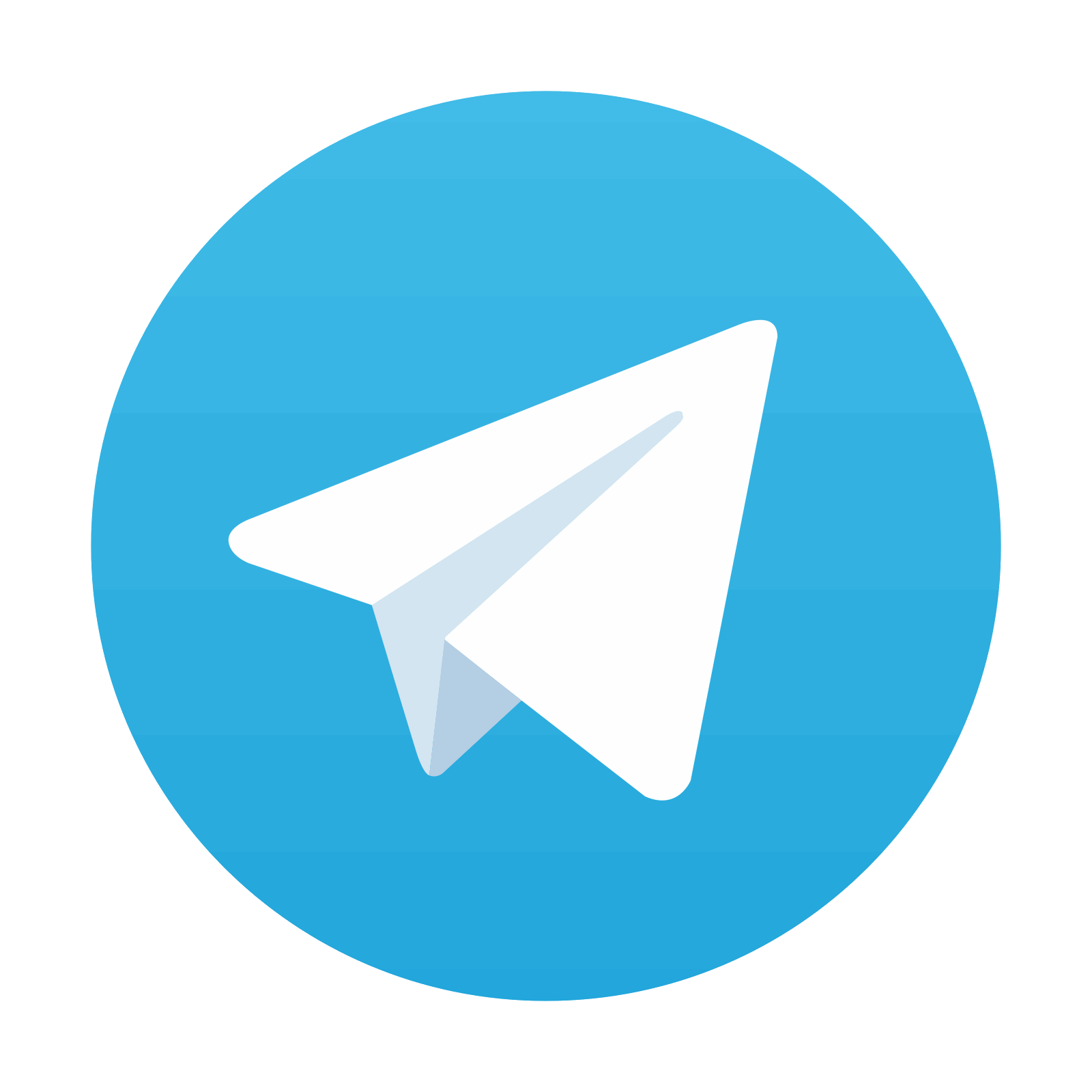
Stay updated, free articles. Join our Telegram channel

Full access? Get Clinical Tree
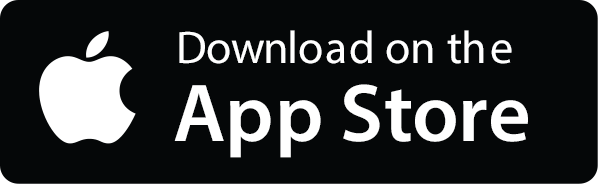
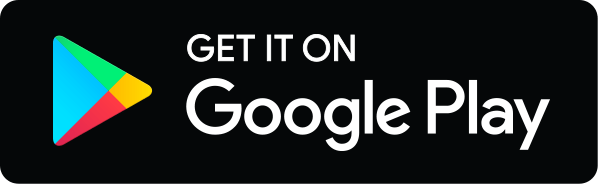