3 Setting Up a Movement Disorder Surgery Practice Brian Harris Kopell, Kenneth Baker, and Nicholas M. Boulis This chapter addresses practical considerations in starting and building a neurosurgical movement disorder practice. This effort may entail merely adding deep brain stimulation (DBS) to an existing general neurosurgical practice; however, it has traditionally implied the creation of a practice focused on functional neurosurgery, usually in the setting of an academic or large specialty clinic. Because of this association, this chapter addresses both scenarios but focuses on the broader functional neurosurgery practice. Functional neurosurgery can consist of an intervention to correct aberrant information processing or altered synaptic communication in the nervous system through surgical ablation (anatomical) or electrical stimulation (physiological) to treat neurophysiological abnormalities. The discipline itself remains loosely delineated with no standard syllabus of skills and disease targets. This confusion is reflected in the significant variance in training offered by different fellowships. The core of functional neurosurgery includes the treatment of epilepsy, spasticity, pain, and psychiatric and movement disorders. However, there is a consensus that psychosurgery should be practiced sparingly and with great circumspection, and some contend that epilepsy and pain surgery are entirely independent disciplines. Finally, the emergence of restorative techniques for neuroprotection, axonal regeneration, gene therapy, and cellular grafting promises to further obscure the already imprecise boundaries of this discipline. It is clear, however, that movement disorders alone will not provide a sufficient caseload to occupy most full-time neurosurgeons. The mix of services offered by functional neurosurgeons must balance the surgeons’ interests with the demand in their individual environment. Thus the demand for DBS may motivate generalists in regions lacking the service to add these techniques, whereas young functional neurosurgeons with a specific interest in movement disorders may be forced to practice general neurosurgery as the focused functional practice evolves. Even more than spine or vascular neurosurgery, functional neurosurgery requires an intricate and diverse relationship with a network of referring physicians as well as a variety of integrated teams. The development of the functional practice usually parallels the evolution of these teams. As the application of DBS is expanded to other fields additional teams will be needed. Thus, when DBS is used as an alternative for cingulotomy, subcaudate tractotomy, and capsulotomy in the field of psychosurgery a very different team will be assembled. Setting up a functional neurosurgical practice poses particular organizational and financial hurdles. This chapter broadly outlines and organizes these challenges. As the technology of functional neurosurgery continues to evolve, some of the specifics discussed in this chapter will cease to be relevant. We anticipate that the technology will become more user friendly, making both acquisition and application easier. However, the hope is that the general principles described will continue to be of use in setting up a practice focusing on movement disorders and functional neurosurgery in general. At present, starting a movement disorder practice can require a considerable capital investment. These expenses will include a stereotactic frame, a stereotactic planning software package or planning station, and neurophysiological equipment. Individual surgeons will vary in their utilization of these three core products. Frameless stereotaxis and the integration of these products are trends that may significantly change the nature of the capital expense required to initiate a DBS program. That is, it may become possible to include the cost of such an integrated system into the implanted devices or for hospitals and practices to lease the systems. Because presently frame-based stereotaxis remains the gold standard, it is assumed that these devices are essential. Three of the most popular frames will be considered here. Most commercially available frames have similar accuracies and idiosyncrasies.1 As such, it is important to choose a frame that will have the most robust company support, ease of use, and compatibility with computer planning systems. Globally, the Leksell Stereotactic System (Fig. 3.1) is the most widely used frame. The frame, designed by Lars Leksell in 19492 after his period of study with Spiegel and Wycis, is the first stereotactic apparatus based on the arc-centered principle. Leksell’s original description of the principle reads as follows: Fig. 3.1 Leksell Stereotactic System (Elekta AB, Stockholm, Sweden, www.electa.com). (See Color Plate 3.1.) “Essentially, it consists of a semicircular arc with a movable electrode carrier. The arc is fixed to the patient’s head in such a manner that its center corresponds with a selected cerebral target. The electrodes are always directed towards the center and hence the target. Rotation of the arc around the axis rods in association with lateral adjustment of the electrode carrier enables any convenient point of entrance of the electrodes to be chosen independent of the site of the target.”3 The current model of the Leksell stereotactic headframe (Elekta Instruments, Inc. Norcross, GA, www.eleckta.com), the Model G frame, is nonferromagnetic and compatible with all available imaging techniques, including computed tomography (CT), magnetic resonance imaging and angiography (MRI and MRA), and positron emission tomography (PET). The base ring is rectangular, measuring 190 mm by 210 mm.4 The x coordinate is set on the arc, whereas y and z coordinates are set on sidepieces attached to the base ring. At the center of the frame x = 100 and y = 100 and 0,0 in the posterior right corner. The arc attaches to the sidepieces. The x, y, and z coordinates are initially set before sterile draping, and the apparatus needed to adjust these coordinates during the procedure is draped out of the sterile field. Thus, to avoid compromising the sterile field, target adjustments should be done by an individual who is not scrubbed. Frame placement can be easily done on a conscious patient with local anesthesia. Generally, two people are required for satisfactory placement of the frame. The CT or MRI results can be sent via a network connection to a computerized surgical planning station (SurgiPlan, Elekta). All major planning software packages include settings to register the Leksell frame. A major advantage of the Leksell frame is its long history of proven use in functional neurosurgery as well as stereotactically guided endoscopy, brachytherapy, radiosurgery, and the like. A typical assembly includes the base ring, two front pieces, a collection of MRI-compatible skull fixation pins, and a set of wrenches for pin placement and removal. With some minor variation, the basic set costs $86,000 to $100,000 and includes the Model G headframe, a set of four posts for pin fixation, a titanium pin set, and the arc system. The second most common headframe used in the United States, the CRW Stereotactic Apparatus is another arccentered system. The CRW frame (Fig. 3.2) is the second generation5 of a stereotactic apparatus first developed by Theodore Roberts, Russell Brown, and Trent Wells in the late 1970s. Most contemporary stereotactic frames use some variation of Brown’s vertical triangulation fiducials to convert images to stereotactic space.6 Like the Leksell frame, it is also a highly versatile, arc-centered system and is compatible with MRI and CT. Fig. 3.2 The Cosman-Roberts-Wells (CRW) Stereotactic Apparatus (Integra Radionics, Burlington, MA, www.radionics.com). (See Color Plate 3.2.) A typical assembly includes a head ring, the arc system, a universal CT/MRI localizer, a phantom base assembly, and trunnion reticles. Its estimated cost is $85,000. The functional CRW package includes sidebars for the ears similar to those of the Leskell frame to more easily apply the frame in a rectilinear alignment relative to the skull. Developed by Fritz Mundinger and Traugott Riechert in 1951.7 this device is a popular stereotactic system in Europe. The RM frame (Fig. 3.3) is a polar coordinate system with the target determined by multiple arcs in lieu of being at the center of an arc. The electrode carrier is offset, and it is desirable to use a phantom (or a computer) to adjust the apparatus. The phantom mechanically simulates the placement of the arc system on the head and the coordinates of the target. Multiple targets require multiple independent calculations and therefore are slower and more complex than on the arc-centered systems discussed earlier. Using this device, Riechert and Mundinger were the first to execute “open stereotaxy,” in which open craniotomy is combined with stereotactic localization. They also used the device in pioneering the use of computers and CT imaging in the targeting of stereotactic procedures. This device can be utilized in a variety of transcranial and transfacial procedures and has also been adapted for radiosurgery. More than 450 of these headframes in over 80 countries are currently in use.8 However, most planning software packages do not include settings for registration of an RM frame. This system is no longer commercially available. Two alternatives to frame-based stereotaxis are currently available. One is the Nexframe system (Fig. 3.4). The other is the StarFix system (Fig. 3.5). Both allow a frameless solution to DBS and stereotactic lesioning procedures. Typically, individual CT/MRI compatible fiducials are affixed to the skull prior to surgery. Currently, the only commercially available bone-fixed fiducials for frameless stereotaxis is the Acustar system (z-kat Company, www.z-kat.com). Bone-implanted rather than adhesive fiducials are used to eliminate targeting error due to scalp mobility. Each fiducial is a self-drilling screw assembly, which allows for MRI- and CT-compatible scanning. This process generally involves small stab incisions at each fixation point, done under local anesthesia. This is usually easier for the patient than stereotactic headframe placement. CT and MRI, as well as target planning, can then be done the day before surgery. The fiducials must remain affixed and immobile for the period prior to surgery. Although a study comparing the accuracy of DBS electrode placement utilizing frame and frameless solutions is still ongoing, preliminary data suggest they may be equivalent.9 Fig. 3.3 The Riechert-Mundinger Stereotactic frame. Fig. 3.4 Nexframe Frameless Microelectrode Recording Guidance Assembly (Medtronic, Minneapolis, MN). (See Color Plate 3.4.) Developed by Image Guided Neurologics (IGN Melbourne, FL, now owned by Medtronic, Neuromodulation [Minneapolis, MN, www.medtronic.com]) this was the first commercially available frameless DBS solution. The Nexframe system includes two burr-hole locking systems for DBS electrode fixation and a tower assembly that allows for targeting in conjunction with a variety of stereotactic planning software packages. A microelectrode recording (MER) guidance assembly that locks in the tower assembly is also available (MicroTargeting from FHC, Inc., Bowdoin, ME, www.fh-co.com). In this system, only target planning is performed prior to surgery. On the day of surgery, once the tower assembly is fixed to the skull over the burr hole, target trajectory is then established by a swivel/pivot mechanism on the tower itself by active/passive laser guidance. If there is any need to change trajectory, this can be done intraoperatively. The per-use cost of this system is $1800 for two base rings and one tower. It is currently compatible with several stereotactic planning software packages. Fig. 3.5 STarFix tripod (FHC, Inc., Bowdoin, ME, www.fh-co.com) coupled to the skull via the Acustar bone markers (z-kat Company, www.z-kat.com). (See Color Plate 3.5.) This system offers an approach that is slightly different from that of the Nexframe. Utilizing the STarFix (FHC, Inc., Bowdoin, ME, www.fh-co.com) requires fiducial placement at least 3 days prior to surgery. Target and trajectory definition are done using a proprietary software package known as VoXim (IVS Solutions AG, Chemnitz, Germany), although efforts are being made to enable the use of other software packages. This information is sent to FHC, where a STarFix system is custom designed for each patient. During surgery, the STarFix tripod is coupled to the skull via the Acustar bone markers. Note that this system requires the intraoperative application VoXim software as well as a microelectrode recording system also proprietary to FHC. Finally, the trajectory is fixed for the course of the procedure. Any change in trajectory will necessitate the use of a new STarFix system. The advantage of this rigidly constrained system is that it eliminates the potential errors in a “moving-parts” system. There are some initial upfront costs to this system as opposed to the Nexframe system. To utilize this system, the VoXim software, Acustar adapters, and microdrive adapters must be purchased for approximately $90,000. In addition, the FHC microdrive must be used with this system; the motorized version costs $24,000. Each STarFix platform costs $1200. There are several avenues to offset these expenses. The platforms are patient chargeable. Also, placement of fiducials can be billed as placement of cranial tongs. The added cost of a frameless solution is partially offset by the safety/comfort advantages and time saved in the operating room (because imaging and case planning can be done prior to surgery). Also, although the total cost may be more, the upfront costs for such systems as the Nexframe are essentially nil because each device can be obtained on a per-use basis. Although the importance of intraoperative physiological mapping with respect to optimizing patient outcome is still debated in some circles,10–13 it is commonly considered to represent the gold standard approach to DBS surgery. This debate and the utility of MER are discussed at length elsewhere in the book (chapter 8). Although no prospective clinical trials are available to support MER, our group utilizes it for all procedures. Apart from our belief that MER optimizes accurate electrode positioning, it may prove to be important in building a referral base. Patient support groups and the Internet have served to enhance the dissemination of information about DBS. Thus both patients and referring neurologists have often been alerted to the variance in application of these techniques. We make a point to explain our view of the importance of MER to both patients and referring physicians as part of the process of building confidence and credibility. Electrical noise in the operating room is one of the greatest inconveniences encountered in initiating a movement disorder practice. In general, minimizing the number of electrical devices functioning in the operating suite can eliminate noise. Nonetheless, early in the process of developing a practice an MER system should be tested in the operating room that is to be used for DBS. Some MER systems are more susceptible to noise. Importantly, critical operating room equipment that generates significant noise may need to be replaced. In some cases, architectural issues may require relocation of the planned operating room (personal experience). The MER equipment constitutes an additional capital investment, currently ranging from $50,000 to $100,000. Although such systems actually consist of numerous components, a typical system can be separated into the microdrive and its accessories, the collection of electronics used to amplify and filter the signal, and an oscilloscope and/or high-quality audio monitor. Table 3.1 gives a list of some of the more popular commercially available MER systems. Such systems offer an “all-in-one” package of the various components discussed in this section. Self-compiled systems, using hardware acquired from various vendors of neurophysiology equipment, can be substantially cheaper than integrated systems. However, this do-it-yourself method requires a high level of expertise for both construction and maintenance. Fortunately, prepackaged MER equipment includes both the recording equipment as well as microdrives with adapters for the Leksell and CRW frames. Slavin recently published a comparative review of the fundamental features of the systems currently available.14 However, the quality and nuances of any system should be evaluated carefully, and, where possible, it is best to consult others who have used or are using a given system and to observe each system in operation. Many commercially available MER systems are capable of moving up to five electrodes simultaneously. The movement of each electrode is not independent because these systems rely on a single motor or turn screw to advance and retract the array. If one is considering using more than one simultaneous electrode, we strongly recommend obtaining a commercially available system because custom design and assembly of such a system are complicated and potentially expensive. It is important to consider not only the durability of a microdrive in the face of repeated manipulation and sterilization, but also the ease with which its parts can be assembled under sterile conditions. This is particularly important for the guide tubes and cannulae. In addition, the drive and its cables must be as light as possible so as not to induce undesirable torque on the frame system without sacrificing quality. Current drives can be classified as manual, motorized, or hydraulic, with each group having distinct advantages and disadvantages. Motorized and hydraulic drive types enable the surgical team to control the action of the drive, and hence the microelectrode, from a remote location. In contrast, manual drives require the surgeon to advance or retract the microelectrode by manually rotating a turn screw system. This task can be ergonomically awkward and can slow down the process of mapping, which requires an integration of patient examination, evaluation of the recordings, and adjustments to the electrode position. In contrast, hydraulic systems do not generate electrical noise. These systems consist of a slave cylinder placed atop the drive unit. The cylinder is attached to a manual or motorized master cylinder unit by means of hydraulic tubing. This setup has long been preferred in laboratory neurophysiological applications, enabling remote control while keeping the motor, if present, sufficiently distant from the microelectrode so as not to induce artifact. Of greatest importance with respect to efficiency, this setup allows for almost continuous sampling of the trajectory with minimal or no movement-related artifact. The foremost disadvantage to hydraulic systems is the potential for the hydraulic tubing to break or leak, releasing fluid that is potentially nonsterile. Further, hydraulic systems tend to require more maintenance, including the constant need to replenish fluid that has evaporated. The components of the recording equipment are extremely sophisticated and encompass many individual features. The most important of these features is the quality of the signal output. Given their impedance, microelectrodes make superb antennae and quite readily pick up extraneous electrical noise. As such, a good MER system must incorporate both active and passive techniques to shield the signal from noise, as well as subsequent electronics to further filter any pervasive noise. The system should also be simple to operate. Although most systems now include more sophisticated techniques for analyzing the extracellular action potential data, in most cases little more is needed than the raw data signal. Because the human ear is often better at discerning a neuronal action potential and patterns of firing than the eye, a high-quality audio monitor is again warranted. The microelectrodes themselves are typically tungsten or platinum-iridium metal electrodes with tip exposures of 10 to 20 μm. Platinum-iridium metal electrodes are usually preferred because, in comparison with tungsten electrodes, there is less electrode erosion. Electrode erosion results in impedance changes during microstimulation, degrading recording quality. One final consideration to be made when exploring commercial MER systems is the potential application of the equipment, particularly the recording components, to non-DBS-related procedures. Many of the systems currently available are suited, though not ideal, for recording evoked potentials and thus may be used for other types of intraoperative and outpatient monitoring. Stereotactic software allows the movement disorder surgeon to plan the target and trajectory for a given procedure. A surgical planning software system is not an absolute necessity for a functional practice. Target coordinates for each of the available stereotactic frames can be manually calculated directly from the scanners themselves. However, commercial planning systems can make the process faster and more efficient. The most prevalent commercial systems are manufactured by Medtronic, Radionics, Elekta, and BrainLab (Table 3.2). Each has its own limitations and advantages.15,16 Some are designed specifically for functional neurosurgery, whereas others allow for tumor and spine stereotaxis. These systems provide features that may improve accuracy like image fusion or corrections for frame misalignments. Further, these systems have features that can save time, including image fusion (allowing MRIs to be done prior to day of surgery), preset indirect targets, and general software navigation protocols that speed the process. The most important considerations when choosing a software package are ease of use, compatibility with frame-based and frameless systems, and company support.
Capital Expenses
Stereotactic Frames
The Leksell Stereotactic System (Elekta AB, Stockholm, Sweden, www.electa.com)
The Cosman-Roberts-Wells (CRW) Stereotactic Apparatus (Integra Radionics, Burlington, MA, www.radionics.com)
The Riechert/Mundinger (RM) Apparatus
Frameless Deep Brain Stimulation
Nexframe Deep Brain Access System
The STarFix System
Neuronal Recording Systems
The Microdrive
Recording Equipment
Stereotactic Targeting Software
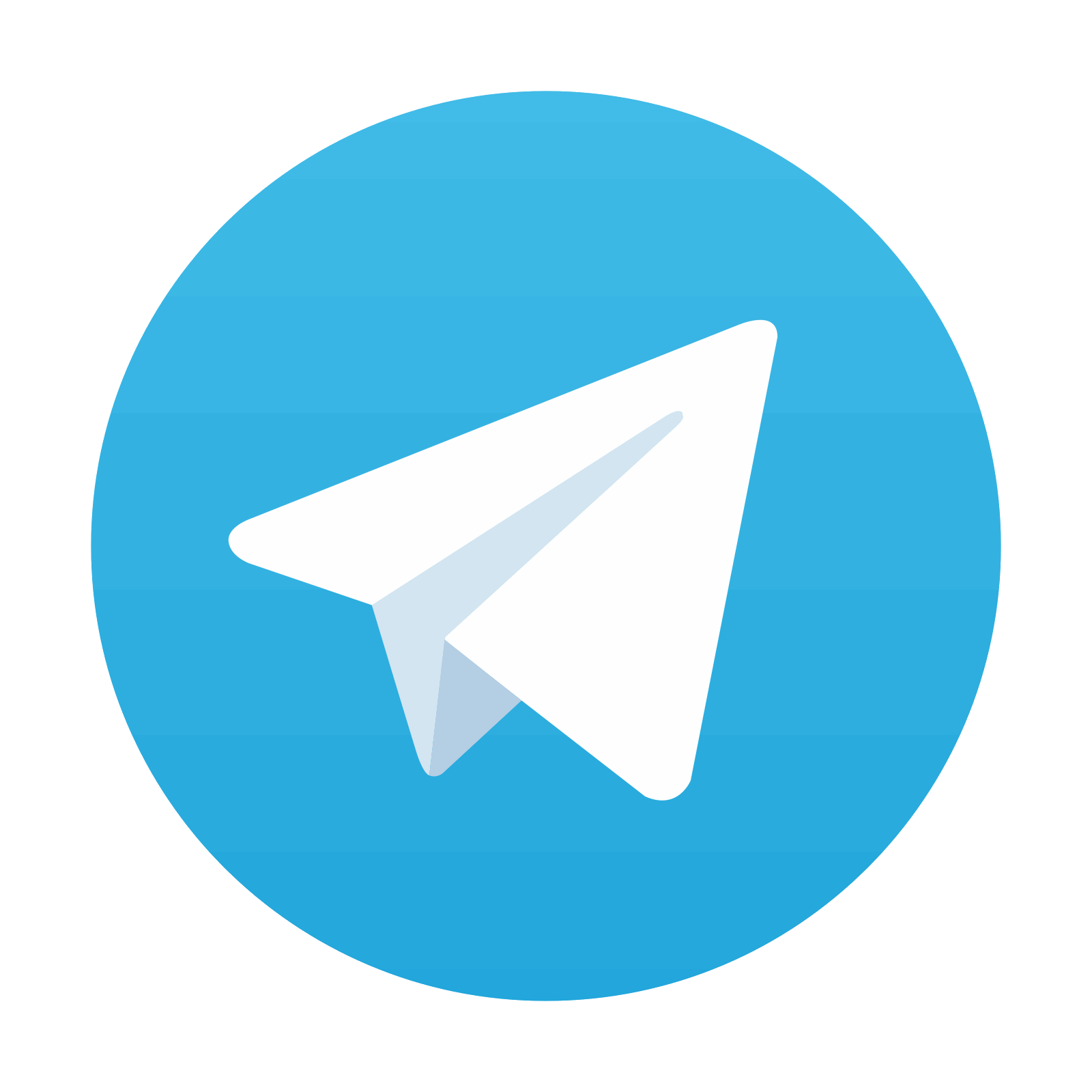
Stay updated, free articles. Join our Telegram channel

Full access? Get Clinical Tree
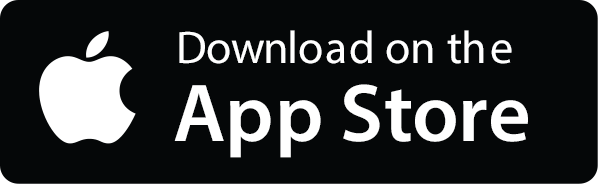
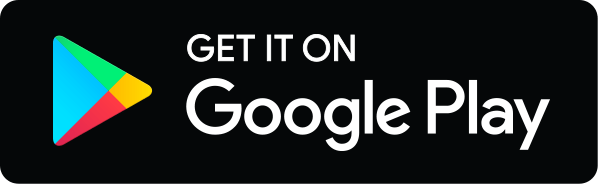