22 Simulation and Training—Preparing for Vascular Injury
Rowan Valentine and Peter-John Wormald
Summary
Vascular injury is perhaps the most challenging complication of skull base surgery to manage. Given that most skull base pathologies are centered around major vascular structures, surgical training on their management is critical. Surgical simulators create a vehicle for the development and teaching of surgical skill acquisition and excellence. Recently there has been a range of vascular injury simulators developed, predominantly synthetic, cadaveric, or live animal simulators. Each model has its own advantages such as portability, anatomical accuracy, or hemostatic physiology. Most importantly, simulated training of endoscopic skull base surgeons has allowed the development of the surgical techniques required to control the surgical field and identify methods and technologies that reliably achieve rapid hemostasis in this setting. Simulated training improves surgeon’s confidence and skill during vascular events, resulting in superior outcomes for patients.
Keywords: Vascular injury, carotid artery, endoscopic, skull base surgery, simulation, surgical training
22.1 Key Learning Points
●Preparing and training for a major vascular event through simulation is imperative.
●Controlling the surgical field is the first vital step; “if you can’t see then you can’t do.”
●Animal simulation models provide surgical confidence in hemostatic techniques with physiological accuracy.
●Simulation and training for vascular injury improves patient outcomes.
22.2 Introduction
Over the last few decades there has been a universally accepted paradigm shift from traditional external approaches to endoscopic endonasal approaches (EEAs) for many areas of the skull base. The benefits of the endonasal corridor over traditional approaches include reduced hospital admission times, improved visualization, minimized surgical morbidity by avoiding sacrifice of intervening structures, and avoidance of external skin incisions and brain retraction.1 This change in direction has been driven by technological innovations and the development of new and improved surgical instrumentation. Vitally important (as with all new surgical corridors) is improved understanding of endoscopic endonasal anatomy, especially the course of the internal carotid artery (ICA). However, surgical technique advances rely not only on improved surgical instrumentation and anatomic understanding but also on surgeon’s training and experience.
Endonasal transphenoidal pituitary surgery has become universally accepted and is commonplace, with a low estimated ICA injury rate of 0.3%.2 Ciric et al performed an interesting postal questionnaire survey involving over 900 microscopic transsphenoidal neurosurgeons, enquiring about their complication profile. Surgeons with an experience of over 500 transsphenoidal pituitary approaches had a 50% chance of intraoperative ICA injury.3 This highlights that increasing subspecialization of endoscopic pituitary surgery will increase the chance that the team will need to know how to manage a carotid artery catastrophe.4 Additionally, endoscopic management of advanced skull base pathologies centering on the ICA come with a greater risk of ICA rupture. Endoscopic resections of craniopharyngiomas, clival chordomas, and chondrosarcomas have a vascular injury rate of up to 2 to 9%.5 , 6 , 7
Vascular injury, during either endoscopic endonasal or open surgery, is perhaps the most challenging complication of skull base surgery to manage. It creates immediate confusion and panic among the skull base team and is incredibly anxiety provoking. Ordered systematic thought during the chaos of a vascular injury is virtually impossible, leading to a need for prospective training of skull base teams in its management. Training of specialist skull base surgical teams on how to manage this challenging event will help to improve patient outcomes.
It is not surprising to see that there is a lack of high-quality research in the field of appropriate techniques and protocols for the management of major vascular injuries. Surgeons are reluctant to publish their experience with vascular injuries and articles that are published are typically case reports only without scientific prospective research into the best surgical techniques required.
22.3 Simulation Training
Simulation of a real-world scenario allows the users to gain experience and to observe and interact with the simulation via realistic visual, auditory, or tactile cues.8 This is usually done in the form of a device or model. Experience with simulators began with flight simulation, used for improving aviation safety and pilot training. Both aviation disasters and major vascular injuries during surgery are unpredictable and uncommon. This makes traditional skill acquisition via the apprentice model of teaching not possible. Surgical simulation creates a vehicle for research and development, to develop and teach surgical skill acquisition and excellence and to transfer these learned techniques to the operating room.9
Surgical simulators each have their own advantages and disadvantages, with a range of different types existing. Bench top-based models are easily portable and cheap, and require no specific supervision but are the least realistic and inanimate. Cadavers have the advantages of anatomical accuracy and high fidelity; however, they can be expensive with limited availability of material. Cadaver models are excellent for surgical field training but are limited for hemostatic evaluation, requiring pump perfusion for a realistic simulation.10 Virtual reality provides the opportunity for reusable simulation with anatomical accuracy, with immediate objective feedback but sense of realism varies greatly and it may also appear inanimate.
Animal models have been used for many years, with experience as far back as 600 bc.11 They play an important role in surgical education and training in the medical field. Live animals have a high level of fidelity, and offer the most realistic experience with regard to major vascular injuries and tissue hemostasis due to the similarities in tissue construct and blood constituents. Major disadvantages, however, are ethical issues, animal welfare laws, anatomical differences, high costs, and requirement of special facilities.
22.4 Vascular Injury Simulation
Surgical skill simulation improves operating room skill, proficiency, and surgical confidence. The development of the ideal intraoperative vascular injury simulator is challenging. It needs to be able to show attributes such as standardization, validation, and reproduction, allowing its use in research and training. Research and development rely on accurate reproducibility of the vascular injury simulator every time it is used.
Creating familiarity is perhaps the most important attribute of a surgical simulator if training in vascular injuries is to be effective. Surgeons need to be immediately familiar with the surgical environment and instrumentation that they will be using to manage the vascular event. This eliminates one major hurdle in skill acquisition. If special instrumentation or materials are required, simulation exercises provide the opportunity for the surgeon to develop familiarity for those rare events when they are employed.
Each scenario of a vascular injury has a unique set of challenging circumstances or constraints. The ideal surgical simulator should be able to recreate these challenges and circumstances. For example, surgical exposure at the site of injury may range from limited such as during a sphenoidotomy during endoscopic sinus surgery to wide surgical exposure during an expanded skull base resection. An endonasal vascular injury also occurs down a narrow nasal corridor where even a small amount of blood may immediately disorientate the surgeon and obscure the surgical field.
Not all vascular injury configurations are the same and will depend on the surgical instrument that caused the injury. Padhye et al12 investigated various hemostatic techniques in a range of injury configurations. Injury types investigated included a 3 mm punch injury, a 4 mm linear injury, and a 4-mm stellate injury. This study identified that the linear injury type was associated with the greatest volume of blood loss and the longest time to achieving hemostasis, hence the most challenging to manage. Clearly the size of the injury also has major impact on the ability to and options for control.
Challenging vascular injury scenarios may have different pressure and volume characteristics. High-flow/low-pressure scenarios such as cavernous sinus bleeding are considered easier to control during endoscopic approaches as the surgical field is less threatened. Visualization of the defect site is easier in low pressure scenarios and easier for the surgical team to act accordingly. In contrast, high-flow/high-pressure injuries such as the ICA create a pulsatile blood stream that rapidly fills the surgical field, making visualization of the injury site much more difficult. In an animal model, rapid exsanguination from an ICA rupture may occur, which will change the vascular event rapidly from a high-flow/high-pressure scenario to the high-flow/low-pressure scenario. Thus, rapid active resuscitation is important during ICA vascular injury simulation to maintain the more challenging high-flow/high-pressure vessel injury characteristics.
Vascular injuries can also have short-term and long-term vessel complications such as secondary bleed, pseudoaneurysm formation, and vascular occlusion. Minimizing morbidity following a carotid artery injury relies primarily on achieving hemostasis, but secondarily on maintaining vascular patency. The ongoing assessment of the injury site over time is important but often overlooked in simulating vascular injuries to allow analysis of the incidence of these complications and determine the best management algorithm.
The working environment should also closely simulate the clinical scenario as much as possible, which has been shown to improve skill acquisition.13 The use of anesthetic machines, monitoring equipment, pressure bag resuscitation, and the ability to monitor blood pressure and pulse parameters immediately recreates the familiarity of a working operating room. Perhaps most important is the recreation of the “life or death” pressure scenario, placing the trainee in immediate and obvious surgical stress. As such, large animals are ideal in vascular catastrophe simulation as they have a similar blood volume to humans, have similar blood pressure, and pulse characteristics and are robust.
22.5 Cadaver Models of Endoscopic Vascular Injury
Recently there have been a number of endoscopic vascular injury cadaver models that offer a range of advantages and disadvantages. These models have the advantage of anatomical accuracy for endonasal surgery12 , 13 and Pacca et al even used an aortic balloon pump to recreate the pulsatile nature of blood flow.10 These models are very useful for training surgeons in surgical field control techniques and improving surgeon’s confidence. The less significant operational cost of these models improves accessibility. The main downsides to these models, however, are the lack of physiological hemostatic accuracy and absence of feedback to the surgeon regarding delayed outcomes of successful hemostatic technique.
22.6 The Sheep Model of Vascular Injury
Animal models of vascular injury provide accurate feedback and confidence to surgeons regarding the use of different hemostatic techniques. Sheep have the advantage of being a robust animal with a similar blood volume to humans and similar-sized carotid artery to the human ICA at the skull base. All sheep are weighed to ensure a weight greater than 20 kg. This minimum size ensures an accurate comparison to the caliber of the intracavernous ICA in humans. Coagulation profiling and full blood count is performed preoperatively. All sheep are fasted 12 to 18 hours before surgery. General anesthesia is induced and performed via injection with sodium thiopentone (19 mg/kg body weight) into the left jugular vein. Endotracheal intubation then follows with maintenance anesthesia maintained by inhalation of 1.5 to 2.0% isoflurane, to a depth that allows spontaneous ventilation and minimizes hypotension.
The Sinus Model Otorhino Neuro Trainer (SIMONT, Prodelphus, Brazil) is added onto the sheep model and simulates the endoscopic environment so that carotid injuries can be managed with the anatomical accuracy of the limitations and confines seen in the human nasal vestibule, nasal cavity, and sphenoid sinus. This model is an accurate reconstruction of the anatomical features of the human nasal cavity and paranasal sinuses. Surgical neoderma is a specifically patented material that recreates the colors, consistency, and elasticity of the nasal mucosa and paranasal sinus bony architecture. This model allows the use of routine sinus and skull base surgical instrumentation and provides the realistic tissue resistances encountered during endonasal surgery. Bilateral large sphenoidotomies and partial middle turbinectomies are performed. A detachable posterior sphenoid sinus wall has been designed to allow the placement of the freely dissected carotid artery into the sphenoid sinus. A plastic cover is placed over the vessel that mimics the thin bony covering over the carotid artery. A specific fastener system has been developed that allows this vessel to be held within the model and prevents blood leaking around the back of the model, allowing blood to run out through the nares of the model. Absence of carotid compression on entry and exit of the carotid artery into and out of the model is confirmed visually and by observing no change in the mean arterial pressure between the left and right carotid arteries. The model construction is then fixed to the operating table and onto the neck of the sheep to prevent displacement during surgical intervention (Fig. 22.1). Importantly, this model has also been modified to allow the use of the internal jugular vein of the sheep, creating a less challenging low-pressure/high-flow vascular injury scenario. This provides stepwise skill acquisition from a less challenging situation to the carotid blowout scenario.
Fig. 22.1 The model is fixed to the operating table with vessel sited within the model.
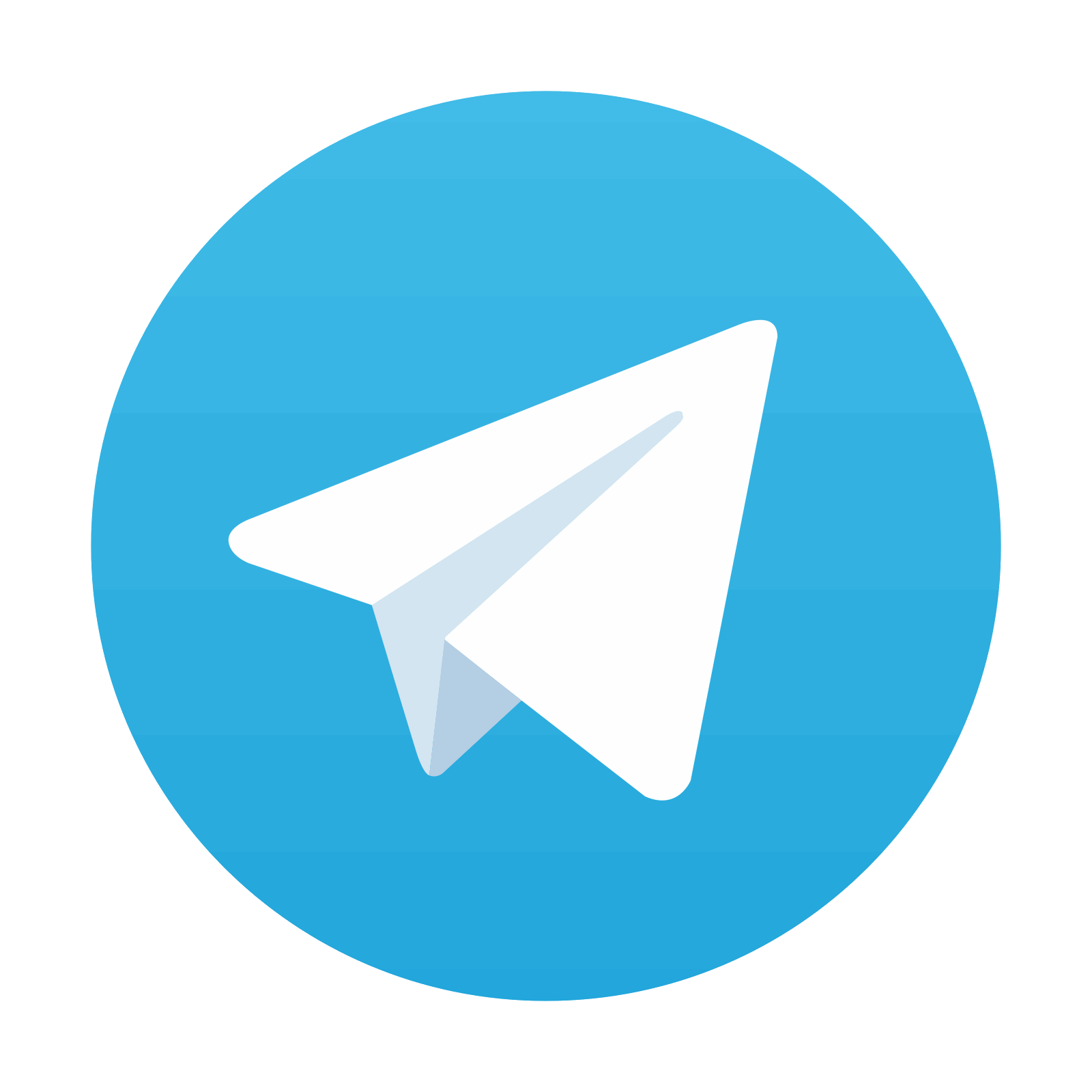
Stay updated, free articles. Join our Telegram channel

Full access? Get Clinical Tree
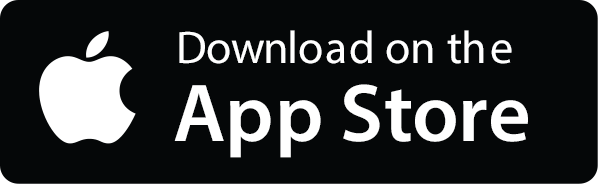
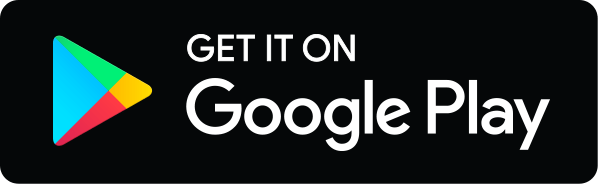