Fig. 4.1
Schematic illustration of skin sympathetic nerve activity. Skin sympathetic nerve activity mainly includes sudomotor nerve activity governing the sweat glands and vasoconstrictive nerve activity innervating the skin arteriolar presphincters of skin vessels. Sympathetic skin response is produced by the movement of sweat in the subcutaneous tissue, and output at the sweat gland orifice provides the sweat expulsion. In contrast, vasoconstriction by vasoconstrictor SSNA causes the reduction in skin blood flow measured by laser Doppler flowmetry
The following describes the basic properties of SSNA.
4.4.1 The Relationship Between SSNA Bursts and Sweating Rate and Skin Blood Flow (Fig. 4.2)

Fig. 4.2
Sudomotor and vasoconstrictor components in skin sympathetic nerve activity. Traces are full-wave rectified and integrated skin sympathetic nerve activity (SSNA), skin blood flow measured by laser Doppler flowmetry, photoelectric plethysmogram, and sweat rate measured by the ventilated capsule method. The latency from the SSNA burst to the inflection of sweat rate identifies the property of the SSNA burst as sudomotor, and that to the reduction point of laser Doppler skin blood flow identifies as vasoconstrictor. The burst indicators are SM sudomotor, VC vasoconstrictor, and SM+VC mixed. The numbers after the burst indicators correspond to the sweat rate inflections and the skin blood flow changes [17]
The report by Bini et al. [1, 2] showed that laser Doppler flowmetry could enable the accurate measurement of skin blood flow, and sweat rate could be measured quantitatively by development of the ventilated capsule method. The full-wave rectified and integrated SSNA sudomotor and vasoconstrictor bursts were proved to be correlated with the slope of the sweat rate and the rate of reduction of skin blood flow by laser Doppler flowmetry, respectively [17].
4.4.2 Effect of Thermal Change in the Environment (Fig. 4.3)

Fig. 4.3
Changes in sudomotor and vasoconstrictor components in skin sympathetic nerve activity depending on the ambient temperature from the tibial nerve and the peroneal nerve
The tibial SSNA innervating to the glabrous skin (sole) and the peroneal SSNA innervating to the hairy skin (dorsum pedis) change their components depending on the ambient temperature [10]
SSNA contributes to thermoregulation by enhancing or reducing its activity, which is lowest under thermoneutral conditions. This thermoneutral temperature depends on ethnic factors and is estimated to be 27 °C in the case of the Japanese [10].
SSNA changes depending on the recorded nerve when the environmental temperature changes. In the peroneal nerve innervating hairy skin, the lowest level was designated at the thermoneutral temperature, and vasoconstrictor SSNAs were predominant at 15 °C, while sudomotor SSNA is predominant at 35 °C. Therefore, the shape of the SSNA showed a hairpin curve, reaching its nadir at 27 °C. In contrast, changes in the tibial nerve SSNA showed the lowest value at 35 °C, with gradual enhancement as environmental temperature decreased. Since the tibial SSNA reflects the stress received from the environment, environmental cold stress enhances the vasoconstrictor SSNA [9, 10].
In this way, sudomotor and vasoconstrictor SSNAs contribute to human thermoregulatory function by altering its components depending on the environmental temperature.
4.4.3 Conduction Velocity of Skin Sympathetic Nerve Activity (Fig. 4.4)

Fig. 4.4
Conduction velocity measurement in skin sympathetic nerve activity. Traces are integrated SSNA recorded at the medial malleolus, integrated SSNA recorded at the popliteal fossa, electrocardiogram, skin potential change, and skin blood flow change measured by laser Doppler flowmetry. The distance between the recording points at the medial malleolus and the popliteal fossa divided by the burst rise time differences provides the sudomotor and vasoconstrictor conduction velocities [18]
A double recording technique [4] enabled us to measure the conduction velocity of SSNA. Two microelectrodes were inserted into the proximal and distal sites of one nerve, the time differences between burst rises or burst peaks were measured, and the velocity was calculated by dividing the distance between the electrodes by this time difference. The conduction velocities of MSNA thus measured were reported to be 0.72 m/s in the median nerve and 1.09 m/s in the peroneal nerve, while those of SSNA were 0.93 m/s in sudomotor, 0.76 m/s in vasoconstrictor at rest, 1.12 m/s in sudomotor, and 0.81 m/s in vasoconstrictor in elicited bursts by electrical stimulation [18].
4.4.4 Change in Skin Sympathetic Nerve Activity During Sleep
In contrast to the MSNA change during sleep, which reduces its activity as sleep stage proceeds, SSNA changes were reported to be the component changes of sudomotor and vasoconstrictor. Generally, it is said that sympathetic nerve activity functions to cool the body during non-REM sleep, but to warm the body during REM sleep.
Takeuchi et al. [19] reported that SSNA reduces its activity until sleep stage 2 as the sleep stage proceeds, while in turn it increases during sleep stages 3 + 4. Kobayashi et al. [20] observed SSNA change during sleep by its effector responses and described that the sudomotor activity during NREM sleep is enhanced compared with that in REM sleep, while vasoconstrictor activity is enhanced during REM sleep compared with that in NREM sleep, the same tendency as reported by Noll et al. [21].
Thus, vasoconstrictor SSNA reduces its activity as the sleep stage proceeds, to cool the human body, while sudomotor SSNA is slightly enhanced to cool the human body during slow-wave NREM sleep (stages 3+4). Contrarily, vasoconstrictor is enhanced and sudomotor is reduced to warm the body during REM sleep.
4.4.5 Effect of Skin Sympathetic Nerve Activity on the Core Temperature Change
Upon exposure to a cold environment, vasoconstrictor SSNA is enhanced to reduce the heat dissipation from the skin surface, and thus, skin blood flow decreases. By this vasoconstriction, it has been observed that the core temperature, measured as the tympanic temperature (Tty), gradually increases with a certain latency, showing the usefulness of recording SSNA in studies of human thermoregulation [22] (Fig. 4.5).


Fig. 4.5
Changes in body temperature and SSNA following acute local cooling. Traces are skin temperature of cooling site, skin temperature of non-cooling site, tympanic temperature, and SSNA burst rate with the sudomotor and vasoconstrictor components. Acute local cooling was provided by inserting both hands into a dry icebox [22]
When we analyzed the magnitude of increase in SSNA upon cold exposure and the increase in the core temperature, a significant correlation between increase in vasoconstrictor burst rate (%) and the rate of change of tympanic temperature was found (Fig. 4.6). This means that the greater the capability to change the SSNA upon cold exposure, the more excellent thermoregulatory function the individual possesses. The cross-correlation between the SSNA and the core temperature showed that the peak latency is approximately 10 min, meaning that it takes approximately 10 min to raise the core temperature upon cold exposure (Fig. 4.7) [22–25].



Fig. 4.6
The relationship between the increase in vasoconstrictor component of SSNA taking the baseline reading as 100 % and the change rate of tympanic temperature per min
A significant correlation is observed between the two, indicating that the larger the individual who is capable of SSNA, the higher the tympanic temperature, i.e., the core temperature [22]

Fig. 4.7
Cross-correlation between SSNA and tympanic temperature during ambient temperature drop. The cross-correlogram exhibited at its maximum at 10 min indicating that the SSNA discharge provides the maximum tympanic temperature rise at 10 min after the discharge [22]
In contrast, we also reported that rewarming from a cold environment of ~15 °C suppresses the vasoconstrictor SSNA (Fig. 4.8), and the greater suppression, rate of vasoconstrictive SSNA is associated with the larger suppression of the core temperature (Fig. 4.9) [25]. Cross-correlogram between SSNA discharge and the core temperature (tympanic temperature) change designated that the time delay exhibited ~7 min (Fig. 4.10), indicating that not only the cooling but also warming takes approximately 7–10 min to raise the core temperature through the convectional pathway.




Fig. 4.8
SSNA suppression and tympanic temperature drop with ambient temperature decrease
The traces are room, skin, tympanic temperatures, SSNA, and skin blood flow. The room temperature was decreased to 10 °C and rewarmed to 28 °C. With the room temperature rise, SSNA was suppressed, and skin blood flow was increased, which enhanced the heat dissipation from the skin surface and resulted in a tympanic temperature drop

Fig. 4.9
The relationships between the change rate of SSNA and the tympanic temperature change rate (a) and maximal change in tympanic temperature (b) The established significant correlation indicates the larger the capacity to enhance SSNA, the larger the tympanic temperature to change [23]

Fig. 4.10
Cross-correlation between SSNA and tympanic temperature during ambient temperature rewarming The cross-correlogram exhibited at its maximum at 7 min indicating that the SSNA discharge suppression provides the maximum tympanic temperature drop at 7 min after the discharge suppression [25]
The height of integrated sudomotor SSNA is found to be proportional to the number of sweat glands observed with a video microscope [26]. This means that the height of integrated SSNA represents the driving force to expel sweating droplets, and it well correlates to the slope of the sweat rate.
4.4.6 Effect of Aging on Skin Sympathetic Nerve Activity in Humans
Although it is known that MSNA increases with advancing age, age-related changes in resting SSNA have not been clarified. Attenuation of peripheral effectors has been reported by Watanabe et al. [27]; however, another report described that SSNA in the elderly is decreased compared with that in the young and middle aged, and responsiveness to the thermal environment is also reduced [28].
In a recent study by Greaney et al. [29], it was observed that SSNA response to whole-body cooling is blunted in the elderly, which is related to a marked impairment in reflex cutaneous vasoconstriction, although cutaneous vascular responsiveness to adrenergic stimuli is not reduced and that to a non-thermoregulatory stimulus is preserved. Nevertheless, interindividual differences of resting SSNA are very large, which makes this issue difficult to investigate.
4.5 Clinical Application of Skin Sympathetic Nerve Activity
4.5.1 Investigation of Sweating Physiology
Thermoregulatory sweating is only observed in some primates, including humans. The phenomenon of “sweat expulsion,” which involves the emission of sweat from the sweat gland orifices, has been clarified to correspond to SSNA burst discharges [30]. The more impulses are included in sudomotor bursts, the more sweat glands are driven by SSNA bursts, and the more sweating is facilitated, which contributes to the thermoregulation of the human body.
4.5.2 Investigation of Anhidrosis/Hypohidrosis
Anhidrosis/hypohidrosis is defined as an absence of sweating. It may have multiple etiologies, but the recording of SSNA can identify the site of the lesion (Fig. 4.11) [31–36]. Attenuated SSNA represents neurogenic anhidrosis/hypohidrosis, and no change or activation, especially activation under heat exposure, indicates that a lesion is at a sweat gland. In neurogenic anhidrosis/hypohidrosis, a peripheral origin increases the burst rate, while a central origin does not exhibit an SSNA change (Fig. 4.12).



Fig. 4.11
Secretion of individual sweat glands and various effector responses to spontaneous sudomotor bursts during mild sweating. Skin blood flow, sweat rate, skin potential activity, and skin sympathetic nerve activity recorded from the tibial nerve are indicated. The lower panel indicates the timing of sweat secretion from individual sweat glands observed on a small area of the sole by a videomicroscope. The numerals 1–3 along the vertical bar represent individual glands. Two sudomotor bursts (S with no asterisk) elicited sweat secretion from three or five glands (Nos. 6, 12, 16, 18, 20). Another sudomotor burst (S with asterisk) did not elicit any sweat secretion, although the burst caused small responses in skin potential and local sweat rate. Skin vasoconstrictor bursts (shown as V) induced a transient reduction in skin blood flow. One of the sudomotor bursts contains the vasoconstrictor component. Three vertical lines show the onsets of sudomotor burst, skin potential response, and sweat response

Fig. 4.12
SSNA from acquired idiopathic generalized anhidrosis (AIGA). Traces are ① raw trace of SSNA, ② integrated SSNA, ③ laser Doppler skin blood flow, ④ photoelectric plethysmogram, and ⑤ sweat rate (hidrogram) in the control subject, and in AIGA patient in baseline recording (a), and after electric stimulation (b). No sweat rate increase was observed after electric stimulation at the wrist joint [37]
Idiopathic and secondary anhidrosis and hypohidrosis exist, among which acquired idiopathic generalized anhidrosis (AIGA, 36, 32, 33, 34, 35) is characterized by a generalized absence of sweating without other autonomic and neurologic dysfunctions. AIGA is classified into three subgroups: (1) sudomotor neuropathy, (2) idiopathic pure sudomotor failure (IPSF), and (3) sweat gland failure (SGF), with each subgroup presenting a different pathogenesis. Sudomotor neuropathy is neurogenic and may sometimes be accompanied by vasoconstrictor neuropathy (Fig. 4.13). IPSF is the most common presentation and is characterized by loss of sweating in the absence of any neurological features or destruction of sweat glands by skin biopsy. IPSF is associated with cholinergic urticaria and estimated to be of autoimmune origin. In SGF, skin biopsy demonstrates the destruction and devastation of the sweat glands, which suggests the possibility of the resultant devastation of IPSF. SSNA is normal or even enhanced in IPSF and SGF without neurological deficits but with a generalized absence of sweating, sometimes associated with heat intolerance, especially upon heat exposure [37–39].


Fig. 4.13
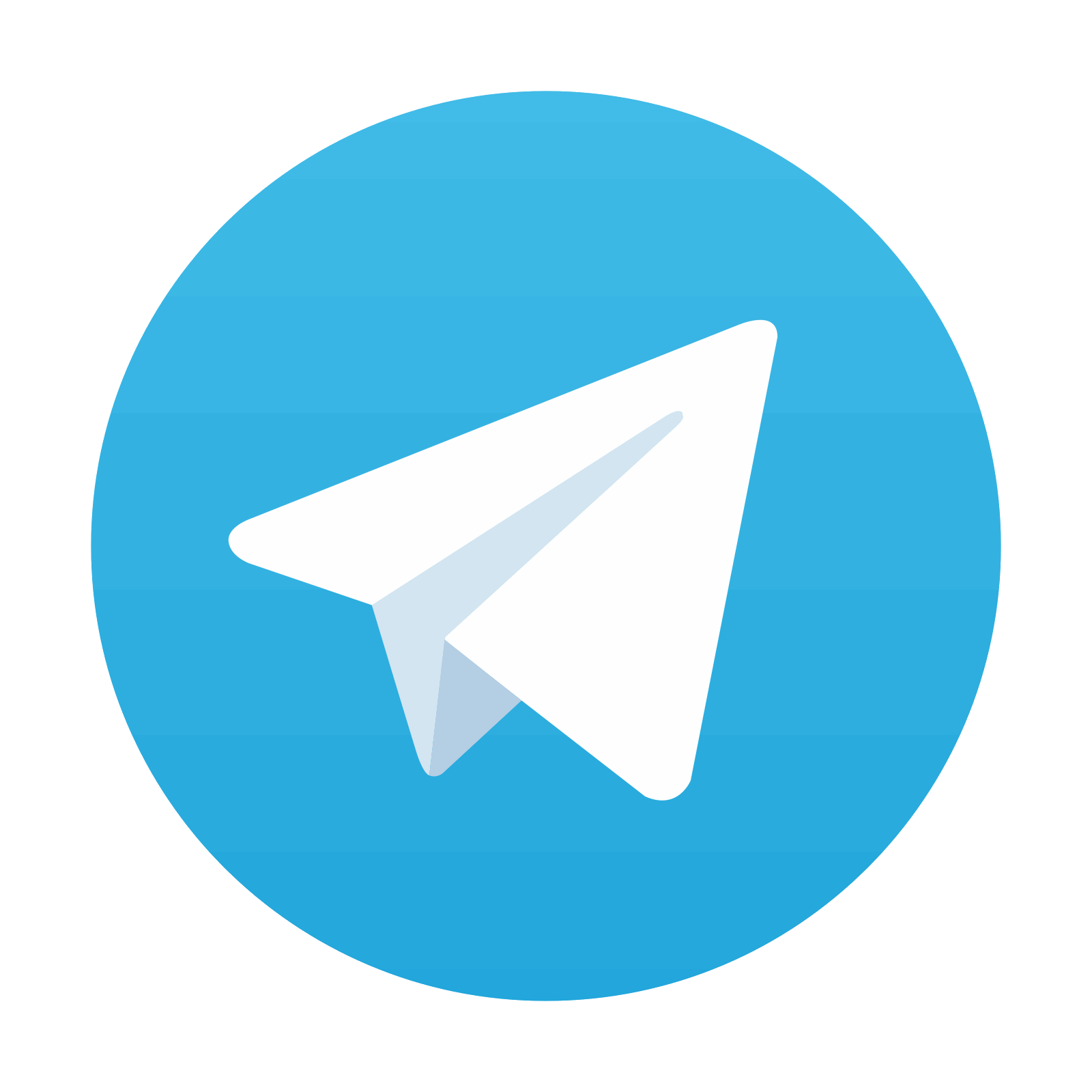
Changes in skin blood flow, sweat rate, skin potential change, and integrated skin sympathetic nerve activity followed by sound/electric stimulations in acquired idiopathic generalized anhidrosis (a) and autonomic neuropathy (b) (a) In acquired idiopathic generalized anhidrosis, resting SSNA is enhanced, reflex response to sudden sound is favorable, and skin potential change exhibits fair response, although no sweating response is observed (b) In autonomic neuropathy, since both sudomotor and vasoconstrictor SSNA are disordered, reduced resting SSNA is observed, and electric stimulation elicits lowered reflex bursts, reduced skin potential change, as well as no sweat response, and no skin blood flow change
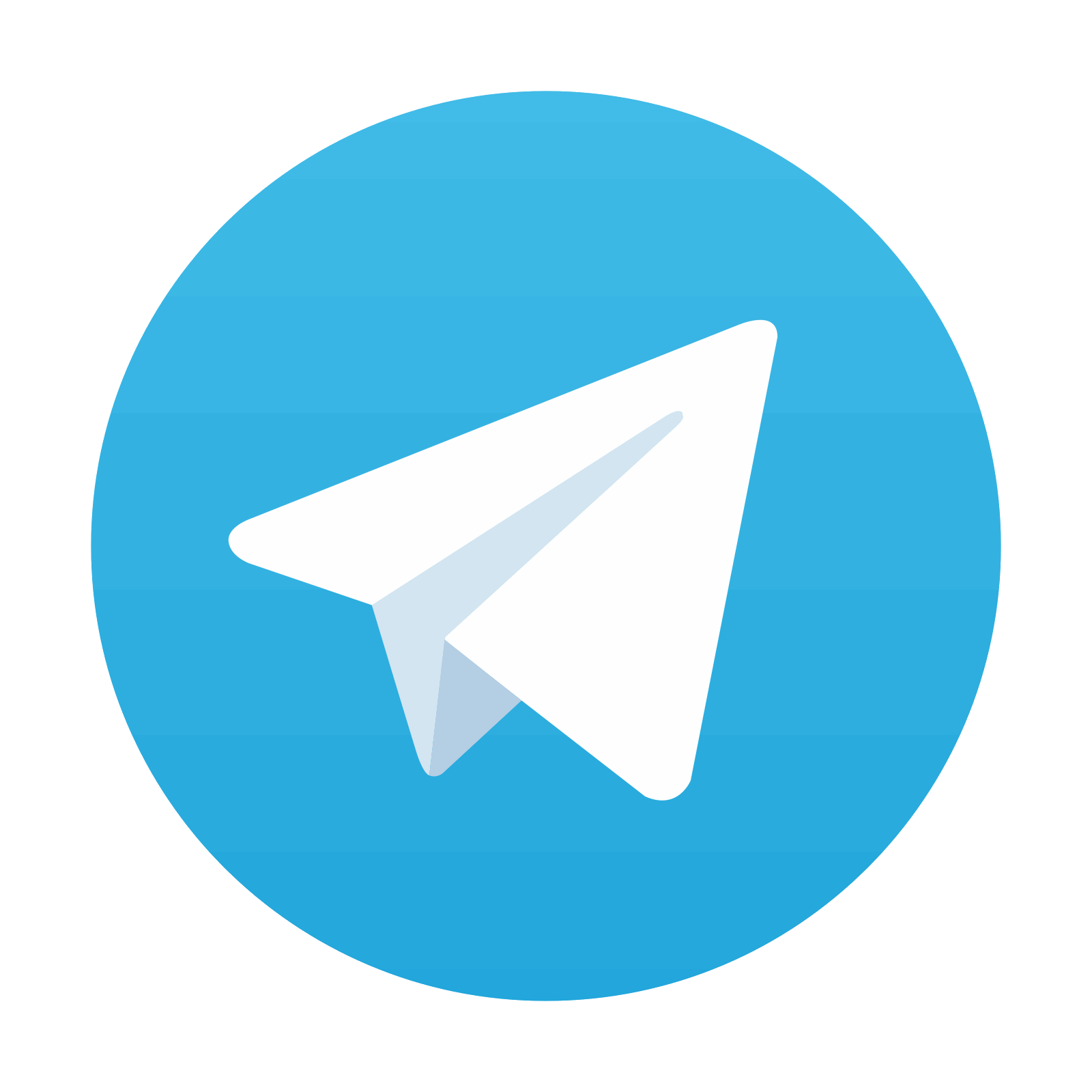
Stay updated, free articles. Join our Telegram channel

Full access? Get Clinical Tree
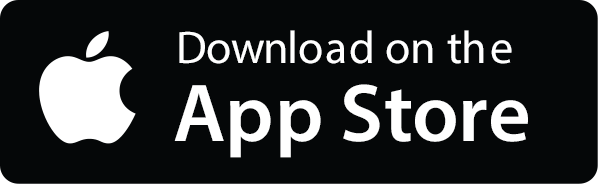
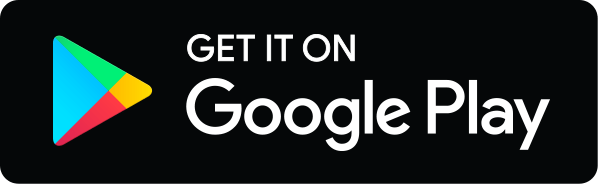
