Sleep and Vascular Diseases
James D. Geyer
Camilo R. Gomez
Paul R. Carney
INTRODUCTION
Vascular disorders, including stroke, myocardial infarction, heart failure, and arrhythmias, are the most common causes of morbidity and mortality in the industrialized world (1,2). Since sleep disorders are also very common (3), vascular disorders and sleep disorders frequently coexist. In fact, aggressive management of sleep disorders may improve the quality of life and prognosis of patients with vascular disease.
NORMAL AUTONOMIC PHYSIOLOGY OF SLEEP
The autonomic nervous system regulates the involuntary, automatic functions of the visceral organs. It is composed of the parasympathetic and sympathetic divisions, two opposing systems, the balance of which determines autonomic function and therefore the status of the vascular system. The sympathetic system is responsible for the “fight or flight” response. Its primary neurochemical mediator is noradrenaline, which produces increased heart rate, increased respiratory rate, vasoconstriction in visceral organs with concomitant vasodilatation in skeletal muscles, increased blood pressure, pupillary dilation, and inhibition of digestion, urination, and defecation. In contrast, the parasympathetic system counterbalances these effects. Its primary neurotransmitter is acetylcholine, which produces slowing of the heart rate and respirations, vasodilatation in visceral organs with decreased blood pressure, pupillary constriction, increased peristalsis, and emptying of the bladder and the rectum.
In general, at sleep onset there is a decrease in sympathetic tone and an increase in parasympathetic tone, which causes a lowering of the heart rate, blood pressure, respiratory rate, and tidal volume. These changes continue during nonrapid eye movement (NREM) sleep (4). With the transition to tonic rapid eye movement (REM) sleep, the portion of REM sleep that occurs without REMs, the parasympathetic activity continues to increase and the sympathetic activity is further suppressed. During phasic REM sleep, the portion of REM sleep that has accompanying REMs, there are bursts of sympathetic activity (4).
During normal inspiration, the heart rate has a brief acceleration in order to accommodate venous return and increased cardiac output (4). During expiration, there is a progressive decrease in heart rate. This normal variability in the cardiac rhythm is a marker for cardiac health and its absence is associated with increasing age and/or cardiac disease. During REM sleep, it is normal for the heart rate to become increasingly variable with episodes of moderate tachycardia and bradycardia. Respiratory patterns are also irregular and may result in mild oxygen desaturations even in healthy subjects. The neurons controlling the principal diaphragmatic respiratory muscles typically are not significantly inhibited during REM sleep, but accessory airway muscles in the rib cage and neck may have partial muscle atonia, causing the diaphragm to bear most of the load of respiration. This may lead to partial diaphragmatic fatigue, hypoventilation, and decreased oxygenation, which can last for several minutes. These episodes are referred to as REM sleep hypoventilation episodes and are not necessarily pathologic (4).
Bursts of sympathetic activity may produce a transient increase of up to 35% in the baseline heart rate, which occurs during phasic REM sleep. Beta-blockers such as atenolol tend to reduce this phenomenon. Increased parasympathetic activity during NREM sleep contributes to cardiac electrical stability and helps decrease cardiac metabolic activity, decreasing the risk of cardiac arrhythmia;
however, the decreased blood pressure produced by the parasympathetic system can contribute to decreased blood flow into the coronary arteries and result in myocardial hypoperfusion, increasing the risk of infarction in patients with significant coronary atherosclerosis (4). The surges in autonomic activity and increased heart rate during REM sleep increase the risk for ventricular arrhythmias. Sympathetic activity results in increased oxygen consumption by the cardiac muscle and also produces coronary vasoconstriction, decreasing the blood flow to the heart, and increasing the risk of cardiac ischemia.
however, the decreased blood pressure produced by the parasympathetic system can contribute to decreased blood flow into the coronary arteries and result in myocardial hypoperfusion, increasing the risk of infarction in patients with significant coronary atherosclerosis (4). The surges in autonomic activity and increased heart rate during REM sleep increase the risk for ventricular arrhythmias. Sympathetic activity results in increased oxygen consumption by the cardiac muscle and also produces coronary vasoconstriction, decreasing the blood flow to the heart, and increasing the risk of cardiac ischemia.
Cerebral blood flow is, of course, closely linked to cardiac output, and the factors described above also impact blood flow to the brain. In addition, during REM sleep, there is an increase in blood flow to the limbic system and the brain stem, with circulation to these structures decreasing during NREM sleep. As brain activity increases during REM sleep, the cerebral requirements for glucose and oxygen both increase, and there is a compensatory increase in oxygenated hemoglobin delivery, which accompanies the transition from NREM sleep to REM sleep. Mild hypercapnia develops during NREM sleep and appears to counteract the circulatory effect of the decreased cerebral metabolic rate during NREM sleep. Partial pressure of arterial carbon dioxide (PaCO2) is an important determinant of respiration and cerebral blood flow during sleep in patients with obstructive sleep apnea (OSA) and other related disorders (4).
PHYSIOLOGY OF OBSTRUCTIVE SLEEP APNEA
Acute Effects
The exaggerated and ineffective inspiratory effort against the occluded pharynx associated with OSA causes more negative intrathoracic pressure. This, in turn, increases left ventricular (LV) afterload and myocardial transmural pressure due to the exaggerated differences between extra- and intracardiac pressure. Venous return to the right ventricle is also increased with a shift of the ventricular septum, which limits LV filling. LV relaxation may also be decreased, which further impairs LV filling (5,6 and 7). The result of these effects is to decrease stroke volume.
Sympathetic tone is modulated by the effects of OSA and the associated altered lung mechanics and hypoxia. Neural feedback via vagal afferents inhibits sympathetic neural outflow during inhalation. This is disinhibited during apnea (8). Hypoxia and hypercapnia associated with apnea further increase sympathetic neural outflow (9). Recurrent apneas and hypopneas cause surges in blood pressure and heart rate (9). This effect appears more pronounced in patients with hypertension (10). Patients with OSA and normal LV function have been described to have elevated concentrations of plasma catecholamine (11), endothelin (12), and natriuretic peptides (13). Following treatment with continuous positive airway pressure (CPAP), these levels normalize.
When respiration resumes at the termination of apnea, stroke volume suddenly increases and, in the presence of the vasoconstricted peripheral circulation, is associated with dramatic surges in blood pressure.
Chronic Effects
Chronic alterations in autonomic, vascular, and endothelial function associated with OSA occur during sleep and wakefulness. Sympathetic neural activity is increased during wakefulness as well as sleep in patients with OSA compared with controls (14). CPAP reduces both nocturnal and daytime sympathetic neural activity. This effect is typically delayed and occurs after several months of therapy (14).
In most patients without hypertension or with essential hypertension, there is a nocturnal decrease in blood pressure (dippers). Patients with OSA often do not have a nocturnal decline in blood pressure (nondippers). This lack of nocturnal blood pressure dipping may be associated with increased cardiovascular risk (15).
The repetitive surges in blood pressure, sympathetic activity, and oxidative stress associated with OSA may promote vascular and endothelial injury. The inflammatory response with leukocyte accumulation and adhesion may then result in atherosclerosis. Elevated plasma levels of adhesion molecules have been reported in patients with OSA (16) and are reduced by CPAP therapy (17).
C-reactive protein (CRP) is an inflammatory marker associated with increased risk of cardiac ischemic events and its level is frequently elevated in patients with OSA. There is a dose—response relationship between the CRP level and the severity of the apnea—hypopnea index (AHI) (18). There are mechanisms linking OSA to increased CRP including repetitive hypoxemic episodes and sleep deprivation.
PHYSIOLOGY OF CENTRAL SLEEP APNEA
Central sleep apnea (CSA) is a type of periodic breathing in which apneas and hypopneas alternate with ventilatory periods, forming a crescendo-decrescendo pattern of respiratory effort and tidal volume. There is instability of ventilatory control associated with oscillation of the PaCO2, which fluctuates above and below the apnea threshold. The cardiovascular, autonomic, and neurohumoral consequences of CSA are less well characterized than that of OSA.
Patients with congestive heart failure (CHF) and CSA have lower PaCO2 during both sleep and wakefulness compared to subjects with CHF but no CSA (19,20 and 21). The mechanism for this chronic hypocapnia is incompletely understood. As systemic arterial oxygen saturation is not lower in affected patients compared with controls without CSA, the etiology cannot be ascribed to hypoxia (19,20 and 21). Patients with CHF are likely predisposed to CSA for
several reasons including increased central chemoreceptor sensitivity, hyperventilation, prolonged circulation time, and altered baroreceptor function (19,20,21 and 22).
several reasons including increased central chemoreceptor sensitivity, hyperventilation, prolonged circulation time, and altered baroreceptor function (19,20,21 and 22).
Increased central and peripheral chemoreceptor sensitivity occurs in patients with CHF and CSA (23). Hyperventilation may also arise from afferent neural input from vagal stretch receptors stimulated by pulmonary vascular congestion (24). Patients with CHF and CSA have higher LV filling pressures as well as lower Paco2 than do patients without CSA (24). The circulation time between the lungs and chemoreceptors is prolonged in patients with CHF and is associated with reduced cardiac output and instability of ventilation because of delayed feedback control (22). Furthermore, baroreflex control is abnormal in patients with ventricular dysfunction, possibly enhancing chemoreflex gain.
VASCULAR PATHOLOGY AND SLEEP
Many case-controlled and cross-sectional epidemiologic investigations have demonstrated an association between OSA and cardiovascular disease (25,26,27,28 and 29). However, confounding clinical factors have made it difficult to definitively establish a causal relationship, with the exception of systemic hypertension, in which the relationship appears more clear. Several large cross-sectional epidemiologic studies support the association between the presence of OSA and the risk for vascular disease. For hypertension, stroke, and ischemic heart disease, the risk appears modest, whereas the relative risk for CHF is highest (26). There also appears to be a relationship between OSA and the risk for atrial fibrillation (30,31).
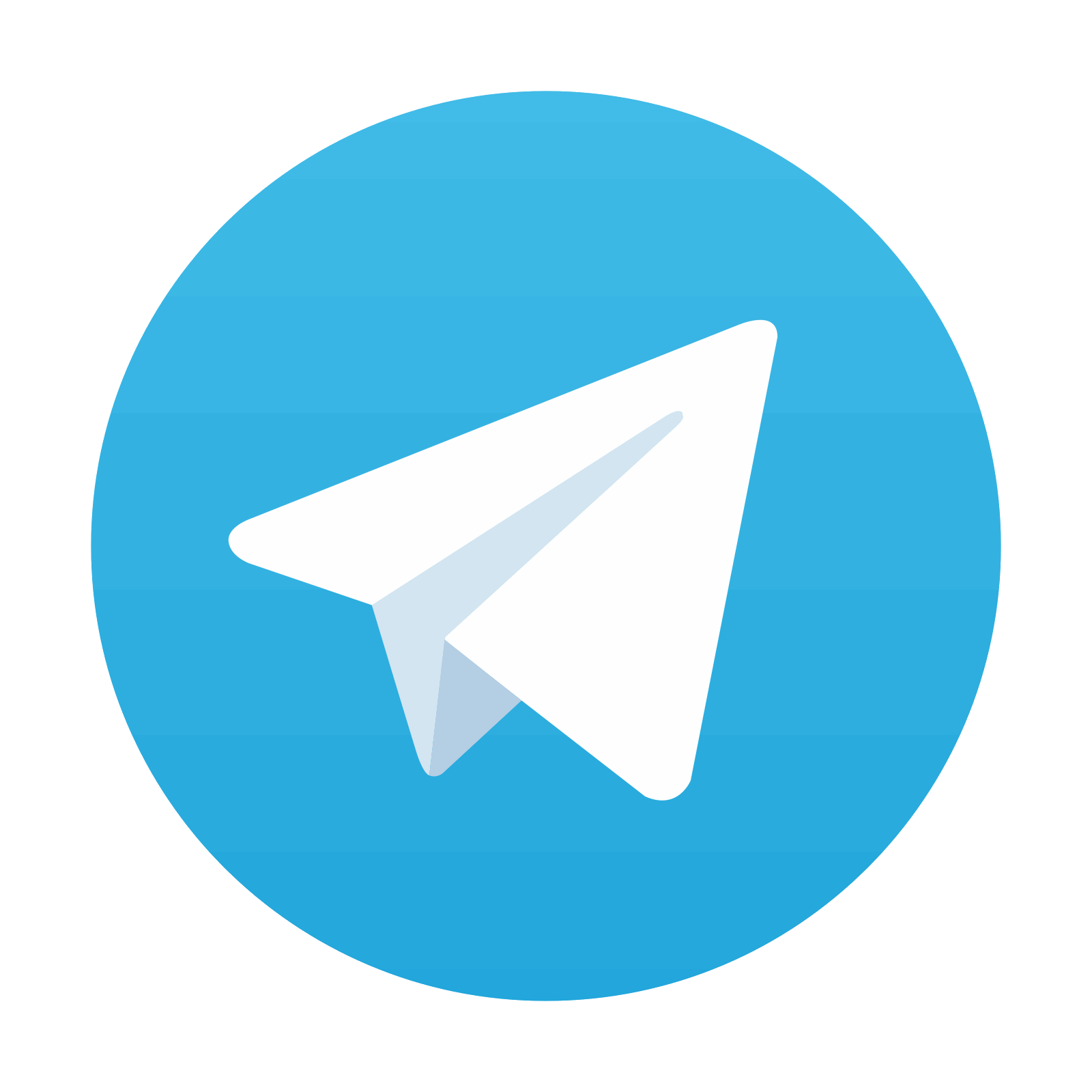
Stay updated, free articles. Join our Telegram channel

Full access? Get Clinical Tree
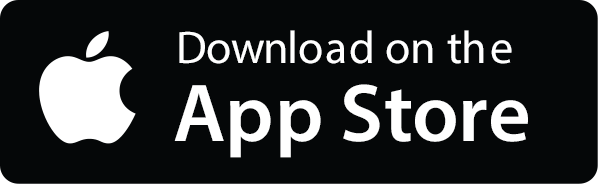
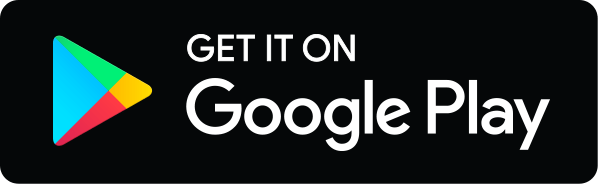