Dementia type
Sleep disturbances
Causes of sleep disturbances
AD
Insomnia
Sundowning
Excessive daytime sleepiness
Decreased REM sleep time
Increased REM latency
Increased nocturnal waking
Increased daytime napping
Medication side effects
Altered sleep/wake cycle
SCN degeneration
Decreased melatonin production
Cholinergic denervation
Progression of dementia
DLB
RBD
RLS
OSA
Insomnia
Sleep fragmentation
Sleep-related leg cramps
Excessive daytime sleepiness
RBD
Medication side effects
Altered sleep/wake cycle
α-synuclein-associated degeneration
Cholinergic denervation
Depression
OSA
PD
RBD
Insomnia
Excessive daytime sleepiness
Sleep attacks
Sleep fragmentation
RLS
PLMS
OSA
Sleepwalking
Muscle rigidity and tremors
Dopamine deficiency
RBD
Medication side effects
Depression
α-synuclein-associated degeneration
Cholinergic denervation
Iron deficiency
Progression of PD
OSA
MCI
Increased WASO
Reduced REM sleep time
Fragmented SWS
RBD
OSA
Poor sleep quality
ApoE ɛ4 sleep
RBD
Altered sleep/wake cycle
Medication side effects
FTD
Decreased TST
Fragmented sleep/wake cycle
Phase-shifted sleep/wake cycle
Nocturnal confusion
Medication side effects
Dementia progression
Frontal variant FTD
HD
Insomnia
Decreased sleep efficiency
RBD (infrequent)
Decreased SWS
Decreased REM time
RLS
Excessive daytime somnolence
Altered sleep/wake cycle
RBD
Dopamine deficiency
Medication side effects
VaD
OSA
Vascular abnormalities
Sleep Disturbances in Dementia
Alzheimer’s Disease
AD is the most common form of dementia , characterized neuropathologically by neuritic plaques (comprised of beta-amyloid) and neurofibrillary tangles (consisting of abnormal tau protein), and clinically by major disturbances in memory followed by other language, behavioral, and sleep disturbances [1, 52]. Approximately 25–64 % of AD patients report sleep disturbances [9, 53]. Sundowning behaviors occur in 2.4–25 % of patients with AD, and altered circadian rhythm results from progressive degeneration of the suprachiasmatic nucleus (SCN) [8, 54]. Sleep disturbances may be present in the early stages of AD, but usually become worse with progression of dementia [52, 55]. Nocturnal wandering and nighttime confusion are common manifestations of an altered sleep/wake cycle, and lead to insomnia, increased nighttime wakefulness, excessive daytime sleepiness, daytime napping, and altered behavior with worsened cognitive functioning, and increased caregiver burden [7, 56].
There appear to be some genetic underpinnings to sleep disorders in AD. A high-activity four-repeat allele of the monoamine oxidase A variable number of tandem repeats (MAO-A VNTR) promoter polymorphism of the MAO gene has been shown to confer increased susceptibility to sleep disturbance in AD [57]. MAO-A has been implicated in the control of circadian rhythm and sleep regulation [187]. The enzyme MAO-A degrades various neurotransmitters and assists with the conversion of serotonin to melatonin , which is partially responsible for synchronizing the circadian rhythm . In addition, AD patients negative for the ε4 allele of the apolipoprotein E gene also experience greater sleep disruption than those positive for the ε4 allele [57].
Recognition and treatment of common comorbid sleep disorders in patients with dementia may lead to improvements in cognitive and daytime functioning. OSA is particularly common in patients with dementia , with 70–80 % having an apnea–hypopnea index (AHI) of > 5, 48 % with an AHI of > 20, and 24 % having an AHI > 40 [59, 60, 61] (Table 33.2). More severely demented patients appear to have more severe OSA [59]. Whether worsening dementia causes OSA or if severe OSA results in more impaired cognition and dementia remains unclear. Nasal continuous positive airway pressure (CPAP) therapy may benefit cognitive functioning in those with mild/moderate AD, with improvements in attention, vigilance , episodic memory, and executive functioning, as well as daytime sleepiness and mood [60, 61, 62, 64]. In addition, caregiver sleep also reportedly improved when dementia patients with OSA successfully maintained CPAP therapy [61]. Patients with mild dementia who are treated with CPAP may show a slower cognitive decline or even improved cognition [64], although CPAP therapy does not reverse many cognitive deficits [63, 64]. Restless legs syndrome (RLS) may be associated with nocturnal agitation and difficulties initiating or maintaining sleep [65]. Checking ferritin and offering iron replacement therapy for patients having low normal (< 50 µg/L) levels is reasonable, and dopaminergic therapies may be offered for patients with disturbing RLS symptoms or frequent movement-related arousals related to periodic limb movement disorder [66].
Table 33.2
Treatment options for sleep disorders
Sleep disorder | Treatment options |
---|---|
Insomnia | Discontinue medications known to cause insomnia Treat pain-causing medical comorbidities Emphasize sleep hygiene and physical activity Melatonin 0.5–10 mg Rozerem 8–16 mg Nonbenzodiazepines (e.g., eszopiclone 2–3 mg, zaleplon 5–10 mg, zolpidem 5–10 mg) Bright light therapy |
Sundowning | Discontinue medications known to cause insomnia Adhere to a strict sleep schedule to help maintain constant sleep/wake cycle Emphasize physical and psychosocial activity Melatonin Bright light therapy |
Excessive daytime sleepiness | Treatment of RBD, OSA, or insomnia Discontinuation of dopaminergic agonists Stimulants (e.g., Modafinil) Discouragement of daytime napping Bright light therapy |
RBD | Clonazepam 0.25–2 mg Melatonin 3–12 mg |
RLS | Dopaminergic agonists: Pramipexole, ropinirole, caribidopa–levodopa Treatment of iron deficiency |
OSA | CPAP |
Sleepwalking | Discontinue zolpidem Clonazepam 0.25–2 mg |
Dementia with Lewy Bodies
Dementia with Lewy bodies (DLB) accounts for approximately 15–25 % of dementia cases, and is characterized by diffuse α-synuclein deposits in the neuronal cell bodies (as Lewy bodies) and axons/dendrites (as Lewy neurites) and associated neurodegeneration. The clinical manifestations of DLB include recurrent fully formed visual hallucinations, spontaneous parkinsonism (i.e., unrelated to medications), fluctuations in cognition and/or arousal, and visuospatial and attention deficits, with more variable memory deficits [67, 68, 69, 70]. Sleep disturbances are very common in DLB, impacting up to 88 % of patients [9]. Patients with DLB are twice as likely to report a sleep disorder than those with AD [9, 71].
At least 70 % of neuropathologically confirmed cases of DLB patients have RBD [9, 52, 68, 72]. RBD is so common in patients with DLB that including RBD as diagnostic criteria for DLB increased the likelihood of autopsy-proven DLB diagnosis sixfold [68]. However, RBD is uncommon in tauopathy neurodegenerative diseases such as AD and frontotemporal dementia , suggesting that key neuronal networks involved in REM sleep control are often affected in the synucleinopathies but infrequently affected in the nonsynucleinopathy disorders [67, 73, 74, 75].
In a recent analysis of PSG findings in DLB patients with sleep-related complaints [76], RBD was common (83 % of 78 patients had RBD confirmed), yet other findings were observed: reduced sleep efficiency (< 80 % in 72 % of the sample), increased respiratory disturbance index (RDI) despite the relative absence of classic OSA features on history and examination alone (mean RDI = 11.9), and frequent arousals unrelated to disordered breathing or periodic limb movements (> 30 %). The authors interpreted their findings as indicating that multiple sleep disturbances may be present in DLB patients with sleep-related complaints [76].
Parkinson Disease
Parkinson disease (PD) is another α-synucleinopathy neurodegenerative disorder in which α-synuclein deposits primarily affect the substantia nigra, resulting in a loss of dopaminergic neurons and frequent cognitive and sleep disturbances [52, 54, 75]. RBD is also strongly associated with PD, with about 46 % of patients reporting RBD symptoms that often precede the development of clinically overt motor symptoms [74, 79, 80, 81]. Insomnia, characterized by difficulties initiating and maintaining sleep, affects approximately 60 % of patients with PD, is more severe in patients with longer duration of PD symptoms, and is often comorbid with depression. [56, 82, 83] . Parkinsonian motor features such as muscle rigidity, tremor, and stiffness make sleep initiation and maintenance more difficult and result in more frequent early-morning wakening . RLS affects approximately 20 % of patients with PD and also contributes to an inability to initiate sleep [84, 85]. Sleep apnea symptoms are frequent in PD patients, seen in up to 49.3 % of patients [86]. OSA occurs in 31 % of PD patients, but polysomnographic studies have shown that OSA is no more frequent in PD than in other patients matched for age and gender [87, 88, 89]. Patients with PD may also experience excessive daytime sleepiness and sleep attacks, the sudden onset of sleep in the setting of normal alertness [52, 90]. Dopamine agonists appear to exacerbate excessive daytime sleepiness and may cause sleep attacks, with larger doses causing decreasing daytime alertness, regardless of disease severity or duration [91, 92, 93]. On the other hand, higher doses of levodopa appear to correlate with improved daytime wakefulness [91] .
FTD, Vascular Dementia, and Huntington Disease
FTD, characterized by executive and language difficulties as well as personality changes, often involves fragmented sleep/wake rhythms with poorer sleep efficiency and decreased TST, and more pronounced phase advances or delays in the sleep/wake cycle that are accompanied by more prominent nocturnal behaviors when compared to AD [90]. Patients with FTD often have an insidious onset with earlier expression of behavioral changes than other dementias. The temporal variant of FTD has a greater propensity for sleep disorders than the frontal variant of FTD and AD [94]. OSA is a common feature of vascular dementia, and leads to fragmented sleep , increased nocturnal confusion, and excessive daytime sleepiness [90]. Sleep in patients with Huntington disease (HD) is highly variable. Choreiform movements are primarily present in waking and light NREM sleep, and patients often have difficulty falling asleep, resulting in excessive daytime somnolence [95]. In addition, sleep spindle frequency and sleep fragmentation is increased, with decreased sleep efficiency, N3 and REM sleep [52, 90, 96, 97, 98, 99]. RBD has also been described in some HD patients [96]. Case studies have reported a possible relationship between RLS and HD, citing RLS as a possible predictor of HD; however, this connection requires further study [100,101].
Mild Cognitive Impairment
Mild cognitive impairment (MCI) refers to a clinical syndrome with impairment in one or more cognitive domains, with essentially normal performance of activities of daily living. There are two subtypes of MCI: amnestic and nonamnestic MCI [102, 103, 104]. Patients with either subtype of MCI experience sleep disturbances, including reduced REM time, more fragmented SWS, poor sleep quality, and OSA [105]. Patients with amnestic MCI, in which memory is primarily impaired, often develop the neuroimaging and neuropathologic characteristics of AD in terms of hippocampal atrophy on MRI, normal striatonigral uptake on dopamine transmitter scanning (DATscan), and positive uptake on amyloid PET scans [102]. Nonamnestic MCI is more often associated with Lewy body pathology. In a study of the Mayo Clinic aging and dementia databases, all patients with RBD and MCI were shown to have autopsy-proven Lewy body disease [106]. Functional imaging shows normal hippocampal structure, absence of amyloid uptake on PET scan, and reduced striatonigral uptake on DATscan, in addition to more visuospatial disturbances [102]. RBD, increased WASO, and reduced executive functioning are also common in nonamnestic MCI, and can be used to help distinguish between MCI subtypes [102, 107]. In addition, patients with amnestic MCI have a higher 5-year rate of conversion to dementia than nonamnestic MCI patients [108]. MCI patients of the ApoE ɛ4 genotype, a main risk factor for developing AD, have decreased REM time and are at increased risk for developing OSA, indicating there may be some link between OSA and AD, although this relationship is not well understood [82, 105, 109, 110].
Sundowning and Complex Nocturnal Visual Hallucinations in Dementia Patients
Circadian rhythm disturbances are common in patients with dementia and affect more than 80 % of those over age 65, resulting in insomnia, excessive daytime sleepiness, and day/night reversal [111, 112, 113]. Sundowning, which can be described as the evening or nocturnal exacerbation of behavioral disturbance characterized by agitation, delirium, anxiety, aggression, and other disruptive behaviors, is often cited as the main reason for the institutionalization of patients with dementia. While the exact prevalence of sundowning is difficult to determine, between 2.4 and 25 % of patients with AD, and 13–66 % of elderly individuals living at home exhibit some degree of sundowning behaviors, with higher percentages being reported in patients with more severe dementia [1, 114, 115].
Sundowning may be viewed as a nocturnal expression of delirium, and has been known since the time of Hippocrates [116]. In the modern era, the first experiment to examine sundowning behaviors was performed in 1941, when demented patients were brought into a dark room for an hour during the day, and subsequently developed delirium [117]. The spectrum of sundowning behaviors in dementia patients may also include mood swings, demanding attitude, resistance to caregiver instruction, and visual and auditory hallucinations [114, 115, 118, 119, 120, 121].
Sundowning is reported more often during the fall and winter months, presumably due to decreasing amounts of sunlight available to help synchronize the circadian rhythm [122]. While the function of the SCN decreases through the process of normal aging, the SCN appears to be more damaged in patients with dementia , resulting in a more drastically altered circadian rhythm. Neuropathological studies of the SCN in patients with AD have shown a greater than normal degree of vasopressin and neurotensin loss, resulting in a decreased ability to set the circadian rhythm [123]. Degeneration of the NBM in AD causes the downregulation of choline acetyltransferase activity and deregulated control of acetylcholinesterase, resulting in a significant decrease in ACh, and causing a further altered sleep/wake cycle [119]. Since the NBM is also markedly affected in DLB and PD, sleep/wake cycle disruption in these disorders may have a similar underlying basis. In addition, a rat model of AD has shown increased locomotor activity just prior to the dark phase, with a corresponding increase in acetylcholinesterase expression in the basal forebrain [119]. Melatonin receptors are also substantially reduced in the SCN of patients with AD, resulting in further circadian rhythm dysregulation. Patients with preclinical AD prior to the onset of cognitive symptoms show decreased CSF levels of melatonin, possibly indicating another biomarker for future neurodegeneration, and providing further evidence for potential sources of disturbed circadian regulation in AD [124].
Management of Sundowning
Many predisposing neurobiological alterations underlie circadian rhythm abnormalities in patients with dementia, so it is important to eliminate or control comorbid medical disorders or medication uses that may further disturb the sleep/wake cycle. Beta-blockers, corticosteroids, bronchodilators, and anti-cholinesterase medications may contribute to insomnia in elderly individuals and should be avoided prior to bedtime if possible [52]. Daily physical activity, exposure to light, social interaction, and adherence to a strict sleep schedule may help balance circadian rhythm disturbances and improve nighttime sleep quality. In fact, the best predictor for institutionalized patients with dementia maintaining a normal circadian rhythm is physical and psychosocial activity [113].
The most effective treatment for sundowning behaviors remains controversial. A systematic review showed that melatonin has efficacy in treating sundowning behaviors and agitation, in addition to increasing sleep quality and improving cognition in patients with dementia , although the optimal dose is unclear [114, 124]. Light therapy has also been effective in stabilizing circadian rhythm in institutionalized patients with dementia. Bright light therapy results in decreased nocturnal awakenings, daytime napping, aggression, and sundowning behaviors. In some cases, bright light therapy has resulted in improved cognition, less reported depressive symptoms, and less functional decline [126]. Again, the best “dose” and time frame for administration of bright light therapy is unclear, since studies have used different light sources and intensities for varying durations administered at variable time frames. Bright light therapy of 2500 lx or greater for at least 1 h during the morning or continuous bright light exposure throughout the day (≥ 8 h) appears to be beneficial in regulating the circadian rhythm , improving nighttime sleep, and decreasing sundowning behaviors [126, 127, 128, 129, 130, 131, 132, 133, 134, 135]. Individuals with a phase-advanced circadian rhythm often benefit from bright light therapy in the evening while those who are phase-delayed should have exposure in the morning [136]. The combination of light therapy and melatonin may also be useful for consolidating sleep and improving mood. However, the severity of dementia, as well as the most prominent type of circadian disturbance, most likely determines the optimal timing, dose, and impact of therapy [90, 126].
Complex Nocturnal Visual Hallucinosis
Patients with dementia often experience complex hallucinations, especially in low light or occurring with confusional arousals from sleep. Hallucinations are common throughout the disease course of DLB, with 80 % experiencing visual hallucinations. The hallucinations may contribute to altered thought content, such as beliefs that others are stealing, persecutory delusions, delusions of reference, infidelity, grandiosity, and, occasionally, somatic delusions. Conversely, hallucinations are reported less frequently and later in the course of AD [71, 137, 138]. Approximately 12 % of patients with PD experience hallucinations, and are even more common in DLB and PD dementia, possibly due to defective control of REM sleep causing REM intrusion into wakefulness [139]. Hypnopompic hallucinations often appear as silent, vivid, and brightly colored distortions of people or animals that occur just after sudden arousal from sleep typically lasting a few seconds and disappearing with brighter ambient light. Hypnagogic hallucinations have similar clinical characteristics and instead occur in the state of drowsiness just prior to the onset of sleep [139, 140]. Hypnagogic and hypnopompic hallucinations may represent intrusions of REM sleep into wakefulness and are often difficult to distinguish from dreams. However, as compared with dreams of REM sleep, rarely do sleep–wake transitional hallucinations evolve or contain a plot. Sleepwalking and night terrors can also present with complex nocturnal hallucinations, or as parasomnia behaviors without accompanying hallucinations [140]. Polysomnography (PSG) may be helpful in distinguishing confusional arousals with hallucinations from other parasomnias such as RBD [141].
As dementia progresses, distinguishing between dreams, visual hallucinations, and reality may become more difficult for the patient. Treatment should be reserved for patients who are frightened or bothered by their hallucinations, and other conditions known to precipitate hallucinations such as urinary tract infections or medication side effects must be excluded. Cholinesterase inhibitors and/or atypical neuroleptics may be effective in treating hallucinations in some patients, however, the atypical neuroleptics should be used carefully in patients with dementia [137].
Evidence for Sleep Disturbances that Could Predict Development of Cognitive Impairment ± Dementia
Sleep Deprivation
The first study of sleep deprivation and cognition was performed in 1896 [142]. Numerous studies since have elucidated the deleterious effects of sleep deprivation on cognition, especially demonstrating prominent deficits in alertness, executive function, memory, and learning of novel tasks that usually return to baseline after sufficient recovery sleep [18, 21, 38, 143, 144, 145, 146, 147, 148]. However, more recent evidence indicates that sleep deprivation and disturbance may be associated with permanent neurobiological changes that may accelerate future cognitive decline. Epidemiological studies show that chronic insomnia is an independent predictor for poor cognition in elderly men, but not women [149]. In addition, nondepressed men with chronic insomnia are more likely to develop cognitive impairment than patients reporting normal sleep. Sleep duration less than 6 h or naps greater than 1 h have also been associated with cognitive impairment [150].
One recent study found an increased and earlier expression of amyloid-beta protein deposits in the brains of sleep-restricted mice, indicating that the sleep/wake cycle may be important in regulating amyloid-beta, and a disruption of this cycle may accelerate development of AD [151]. Rats undergoing REM sleep deprivation also have impaired spatial learning and decreased hippocampal excitability and LTP, the major cellular mechanism underlying learning and memory formation, which may be partially responsible for decreased learning and memory abilities resulting from sleep deprivation [143, 145, 152].
Studies using functional imaging have shown patients undergoing sleep deprivation have decreases in alertness and cognitive function, which correspond with a decreased global metabolism of glucose, especially in the thalamus and prefrontal and parietal cortices [148]. In addition, there is a decrease in the activity level of the medial temporal lobe during a verbal learning task with an increase in prefrontal and parietal activation in sleep-restricted individuals compared to controls, indicating a potential compensatory attempt by other brain regions for the decrease in medial temporal function [18, 153]). Neuroimaging has also revealed that frequent and progressively longer cognitive lapses, which are a hallmark of sleep deprivation, involve widely distributed changes in brain regions including frontal and parietal control areas, secondary sensory processing areas, and thalamic areas [21]. Human subjects and rats who have been subjected to sleep fragmentation or deprived of both REM and NREM sleep also show a reduced neuron proliferation and a reduced number of developing neurons, especially in the dentate gyrus of the hippocampus [154, 155, 156]. Growth hormone appears to facilitate neurogenesis in the dentate gyrus, and has even been shown to protect against neuronal loss caused by prolonged sleep deprivation in rats [157]. However, preserved circadian rhythm or melatonin secretion has little effect on neuronal proliferation, indicating the importance of sleep itself in neurogenesis [158]. Longitudinal sleep loss may cause overall decrease in neurogenesis, primarily in the hippocampus, leading to cognitive dysfunction, especially memory loss, and mood disorders, potentially exacerbating underlying cognitive deficits seen in dementia [155].
Altered Circadian-Activity-Rhythm Architecture
A recent analysis on circadian-activity-rhythm architecture provided insights on whether such rhythms might predict future cognitive decline [159]. The study investigators sought to determine whether circadian activity rhythms as measured by wrist actigraphy in community-dwelling older women are associated with incident dementia or MCI. The subjects were elderly female participants in a longitudinal aging study, in which subjects underwent wrist actigraphy and many other measures during one wave of study (2002–2004), and underwent a comprehensive cognitive assessment in a subsequent wave of the study (2006–2008). This permitted characterization of the subjects ( n = 1282) as cognitively normal, or meeting criteria for MCI or dementia . Their data showed that older women with decreased activity rhythms had a higher likelihood of developing dementia or MCI when comparing those in the lowest quartiles of amplitude or rhythm robustness to women in the highest quartiles. Furthermore, an increased risk of dementia or MCI was also found for women whose timing of peak activity occurred later in the day (after 3:51 p.m.) when compared to those with average timing (1:34 p.m. to 3:51 p.m.). The authors concluded that women with decreased circadian activity rhythm amplitude and robustness, and delayed rhythms, have increased odds of developing dementia and MCI [159].
The direction of the association could not be surmised from these data. The authors suggested that interventions such as physical activity (which would be reflected on actigraphy as increased amplitude, mesor, and robustness) or bright light exposure in the early morning (which would be reflected on actigraphy as advancing the phase) might reduce the risk of cognitive deterioration in the elderly [159]. Hence, future intervention studies with physical activity and bright light therapy may indicate some readily available and inexpensive therapies that could modify the risk of future cognitive decline.
Sleep-Disordered Breathing
SDB primarily results in impairments in attention/vigilance , executive functioning, and memory in addition to construction and psychomotor skills, with more severe SDB correlating with worse cognition [34, 62, 105, 160, 161, 162, 163]. Whether intermittent hypoxemia, sleep fragmentation, or both cause cognitive dysfunction in SDB is unclear. One study inducing intermittent hypoxia at a simulated altitude of 13,000 ft. for 4 weeks resulted in no deficits in subjective or objective alertness, vigilance, or working memory [164]. However, other studies suggest that hypoxemia associated with sleep apnea may result in greater cognitive impairment. In addition, the degree of sleeping and wakeful hypoxemia appears to correlate with the degree of cognitive impairment [165]. A recent study of nondemented community dwelling women over age 80 found that SDB (measured by an AHI > 15 or greater than 7 % of total sleep time with hypoxemia below the oxyhemoglobin saturation margin of 90 %) were more likely to develop dementia or MCI, while sleep duration and sleep fragmentation did not appear to be risk factors for developing cognitive impairment [166]. However, sleep fragmentation and hypoxemia may result in cognitive impairments through different mechanisms. Sleepiness may result from sleep fragmentation, causing memory and attention deficits, whereas disturbances in executive functioning may be due to brain damage caused by hypoxemia [13] .
The prefrontal cortex is particularly susceptible to sleep deprivation and hypoxia. Sleep fragmentation and intermittent hypoxemia have both been shown to lead to neuronal loss in the hippocampus and prefrontal cortex, which are closely associated with memory processes and executive functions. In addition, the prefrontal cortex is believed to be involved in attention control, providing a basis for the attention deficits seen in patients with OSA [64, 167]. Some studies have shown a gray matter reduction in the right middle temporal gyrus, cerebellum, anterior cingulate, hippocampus, and parietal and frontal cortices in OSA patients with cognitive deficits [168, 169, 170, 171, 172]. After 3 months of treatment with CPAP therapy , improvements in cognition were seen with an increase in gray matter density [168]. While white matter changes in OSA have been less extensively studied, patients with OSA do exhibit changes in axonal connections between the limbic system, pons, cerebellum, and the frontal, parietal, and temporal cortices [173].However, there are currently little data correlating brain morphology changes and cognition in OSA [64] .
Recurrent episodes of hypoxia due to OSA may cause structural ischemic lesions in the prefrontal cortex, which could explain the greater cognitive impairment and lack of symptom reversal with therapy in more severe OSA cases [64]. Some individuals possess cognitive reserve marked by a lower resting metabolism and increased neural activation during activity on functional imaging that may allow them to be less susceptible to sleep deprivation caused by frequent arousals in OSA [64].
Recent research suggests that TNF-α may play a role in excessive daytime sleepiness and cognitive dysfunction in mice subjected to recurrent arousals from sleep, such as those seen in OSA. TNF-α induces sleepiness and enhances SWS [174]. However, excessive TNF-α can inhibit LTP and disrupt hippocampus-dependent memory consolidation [175, 176] . Increased TNF has been shown to be a risk factor for developing AD. Mice subjected to sleep fragmentation showed increased levels of TNF-α around cortical neurons and exhibited cognitive problems and sleepiness, while maintaining baseline amounts of sleep duration and similar sleep architecture. However, double knockout mice without TNF-α receptor, or those treated with a TNF-α antagonist, showed little cognitive dysfunction or daytime sleepiness, indicating that sleepiness and cognitive dysfunction in disorders of frequent arousal may be partially mediated by TNF-α [176].
Whether SDB contributes to the pathophysiologic processes involved in neurodegeneration inherent in the degenerative dementias remains unclear. One recent study suggested that daytime hypersomnia and other sleep disturbances increased the risk of developing vascular dementia [177]. Furthermore, OSA almost certainly exacerbates the cognitive deficits seen in dementia and normal aging, indicating that early treatment may be beneficial if OSA is detected and may prevent faster than normal cognitive decline [64].
REM Sleep Behavior Disorder
RBD, characterized by the loss of skeletal muscle atonia and enactment of dreams, was first described in 1965 in cats with bilateral pontine lesions near the locus coeruleus, and formally identified in humans in 1986 [178, 179]. Traditionally affecting men older than 50, RBD is a hallmark of DLB and other α-synucleinopathy neurodegenerative disorders , including DLB, PD, and multiple system atrophy (MSA) [73, 75, 180]. PSG is required for diagnosis of RBD in order to identify the presence of REM sleep without atonia (RSWA) , the neurophysiologic substrate of RBD, in addition to ruling out nocturnal epilepsy or NREM parasomnias [141]. Approximately 80 % of patients with idiopathic RBD (iRBD) develop a synucleinopathy disease given longitudinal follow-up, and RBD symptoms can present up to half a century prior to the onset of neurodegenerative symptoms, providing a potential window of opportunity for delivery of future neuroprotective therapies for individuals who may be predisposed to impending neurodegeneration [73, 74, 75, 79, 181, 182]. The association between RBD and the synucleinopathies has been largely responsible for the growing interest of neurologists in the field of sleep medicine.
Patients with iRBD have shown decreased cerebral glucose metabolism on fluorodeoxyglucose (FDG)-PET scans in the occipital lobe, the area predominantly affected in DLB, while hypometabolism in the left anterior cingulate gyrus, right frontal lobe, and right anterior temporal lobe (areas primarily affected in PD) were the main findings in another subset of patients [69]. These findings support the contention that iRBD is often a very early clinical manifestation of a synucleinopathy neurodegenerative process. In addition, studies of α-synuclein immunohistochemistry in neurologically normal individuals have shown different regions of α-synuclein deposits in the brain, which may explain the variation in glucose metabolism [69, 183]. Occipital glucose hypometabolism may be related to visuospatial impairments seen in patients with DLB [69. Subjects with iRBD also show decreased striatal dopamine uptake in the substantia nigra on 23I-2β-carbomethoxy-3β-(4-iodophenyl)-N-(3-fluoropropyl)-nortropane (123I-FP-CIT) single-photon emission computed tomography (SPECT) and nigral hyperechogenicity on transcranial sonography (TCS), similar to that seen in PD and DLB [184]. In addition, patients with PD and RBD show neocortical, limbic, and thalamic cholinergic denervation not seen in PD patients without RBD, indicating that RBD may also be associated with cholinergic neuronal degradation [185].
Nondemented iRBD patients followed up for 2 years showed a decline in verbal memory and visuo-constructive functions when compared to nondemented age-matched controls [186]. While none of these patients developed dementia at time of follow-up, impaired visuospatial ability is a hallmark of DLB, indicating that these patients were likely undergoing an alpha-synucleinopathy neurodegenerative process. In a population based study, individuals with iRBD were 2.2 times more likely to develop MCI or PD than those without RBD [187]. RBD is also much more common in PD, with 69 % of those with PD also having probable RBD, while probable RBD was only seen in 20 % of those with RLS and 13 % having essential tremor [188] strengthening the belief that RBD is an early clinical expression of a synucleinopathy neurodegenerative process and suggesting that patients with RLS or essential tremor are not at increased risk for the development of PD [188]. RBD may also be a predictor of dementia in patients with PD. In one study, 48 % of PD patients with RBD developed dementia, while no PD patients without RBD developed dementia [189]. However, all 13 patients who developed dementia had MCI at baseline examination, indicating that they may have already been progressing towards dementia.
Loss of REM muscle atonia (RSWA) during PSG may also be a predictor for future development of dementia . In one recent study of RSWA, patients developing dementia had a 73 % muscle atonia loss, compared to only 40 % in those that did not develop dementia [74, 190]. Finally, RBD is also associated with the development of new hallucinations and cognitive fluctuations in patients with dementia. Patients with DLB or PDD were more likely to have RBD- or NREM-related arousals and hallucinations than nondemented PD patients, with more frequent arousals and dream enactment behaviors (DEB) in more severely cognitively impaired patients [80, 191].
The treatment of RBD focuses on decreasing potentially injurious DEB [72, 75, 192]. Clonazepam and melatonin have been shown to be equally effective in decreasing frequency and severity of DEB and injury occurrence in RBD [192]. However, melatonin was reported to be more effective in RBD patients with a neurodegenerative disorder compared with clonazepam [192]. This finding, coupled with reports of decreased sundowning behaviors in melatonin-treated dementia patients, provide evidence for use of melatonin as a potential first-line treatment for both RBD and sundowning in patients with dementia [192, 193]. Unfortunately, neither melatonin nor clonazepam has been found to slow progression of neurodegeneration in RBD and further research on potentially neuroprotective therapies is needed.
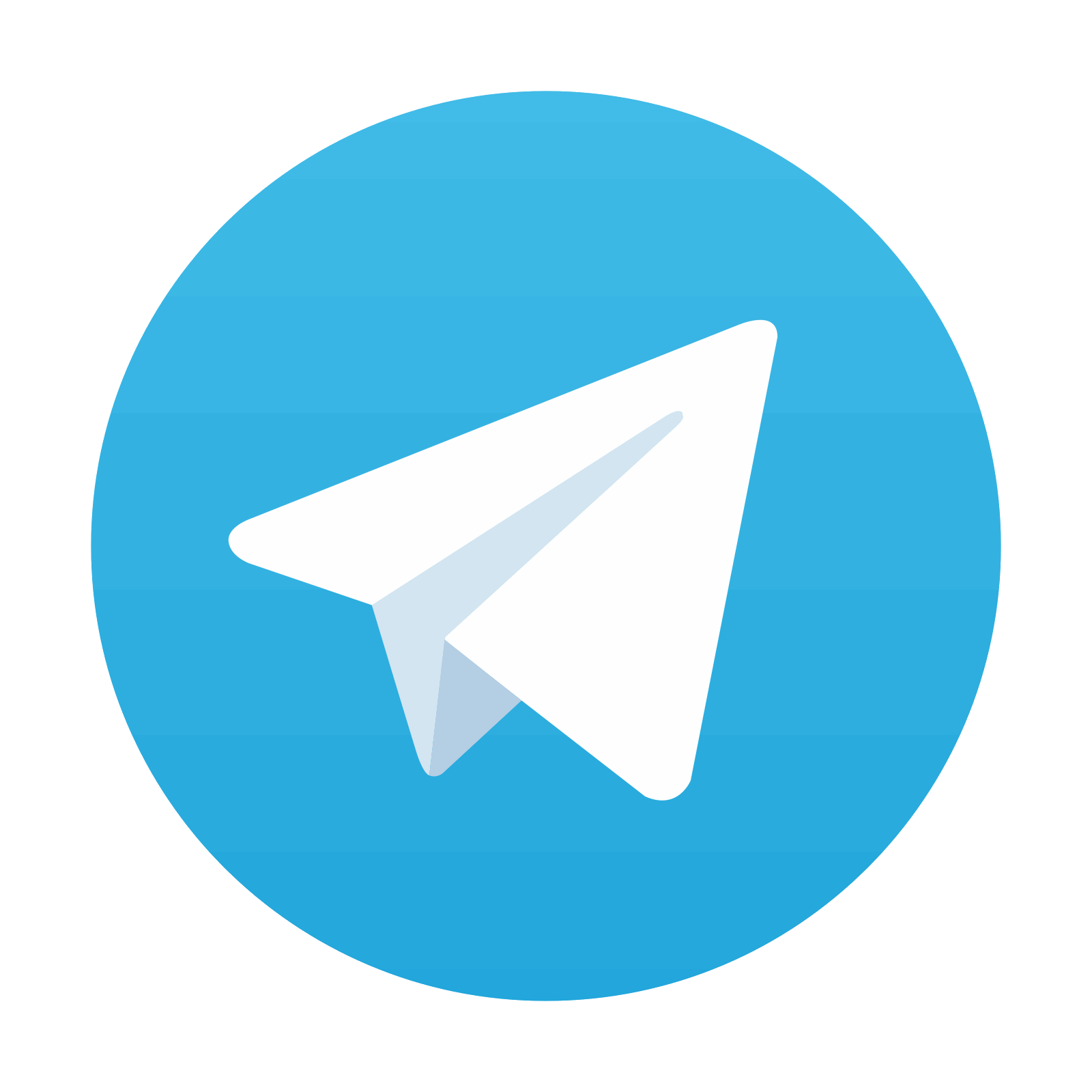
Stay updated, free articles. Join our Telegram channel

Full access? Get Clinical Tree
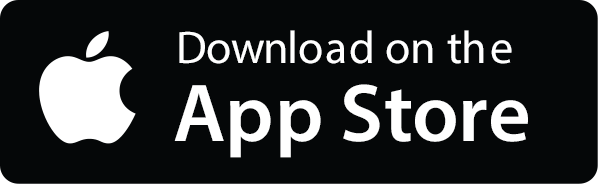
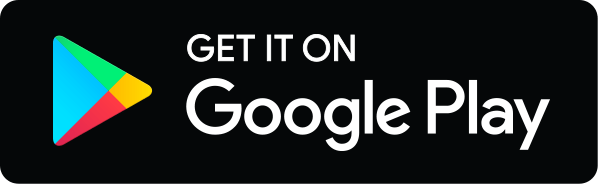