Fig. 43.1
The two main components of consciousness: wakefulness and awareness. In most pathological and physiological states, the two components are linearly correlated along the spectrum of consciousness. In some cases, however, they are dissociated. Vegetative state/unresponsive wakefulness syndrome, VS/UWS; minimally conscious state, MCS and emergence of MCS (EMCS). Adapted from [4]
Here, we review the clinical entities of disorders of consciousness (DOC) following brain injury (coma, VS/UWS and MCS) and the knowledge on (un)consciousness obtained by studying these patients, mainly focusing on functional neuroimaging and neurophysiological studies. We will then see how these neuroimaging techniques can detect covert awareness in this challenging patient population and, finally, we will further consider the converging points between loss of consciousness in pathological states such as DOC and in physiological states such as deep sleep.
Disorders of Consciousness
In the last decades, the development of emerging medicine and life-saving techniques has resulted in a progressively increased number of patients surviving after sustaining severe brain damage. Ever since, we witnessed a range of clinical entities of DOC never encountered before. DOC are among the most challenging and poorly understood conditions of modern medical care. The main difference between DOC and other states of unconsciousness, such as sleep, pharmacological anaesthesia and epileptic seizures, stems in the prolonged impaired awareness following severe brain damage. After a severe brain injury, a patient can spend some time in a coma, a condition characterized by the lack of both awareness and wakefulness, as evidenced by the closed eyes. Coma may arise after structural or metabolic lesions of the brainstem reticular system or after widespread bilateral cerebral damage [10]. The condition of coma usually does not last longer than 4 weeks, after which patients either evolve to brain death (i.e. permanent loss of brainstem functions), or completely recover consciousness or evolve to a VS/UWS [11]. VS/UWS is a condition of wakefulness, as evidenced by the opened eyes, without clinical evidence of awareness. This means that patients in a VS/UWS show only reflex behaviour, without any sign of voluntary conduct [4]. VS/UWS can be acute and reversible, as a transition before progressing to higher levels of awareness, or chronic and irreversible [12]. It has been proposed that the VS/UWS is permanent after 12 months following traumatic brain injury (TBI) and 3 months following non-traumatic insults, and therefore, chances for recovery are poor [13]. However, patients with late spontaneous recoveries have challenged these proposed time boundaries, and as such, the connotation of the VS/UWS has been revisited [14]. It has been recently recommended to substitute the term ‘permanent’ by the aetiology of the injury (traumatic or non-traumatic) and the length of time since onset, as these factors have been shown to influence outcome [15]. Patients from VS/UWS may evolve into a MCS, which may be the endpoint of their improvement or a temporary stage on the way to further recovery of consciousness [16]. MCS is a related diagnostic category recognized in 2002 [16]. It differs from the VS/UWS by the presence of discernible, yet inconsistent, non-reflex behaviours as a response to visual, auditory, tactile or noxious stimuli [16]. Although some patients in MCS may show intentional signs of communication [17], by definition they are not able to communicate accurately with their environment. The typical lesion profile consists of grade II or III diffuse axonal injury with multifocal cortical contusions, sometimes accompanied by thalamic involvement. In comparison with patients with VS/UWS, these patients show relatively preserved long-range thalamo-cortial pathways, which could explain why patients in a MCS retain some capacity for cognitive processing [15]. Diagnosis of MCS is clinically based on discernible and reproducible evidence of conscious awareness such as visual pursuit, simple command following, intelligible yes-no response and/or behaviours that are selected triggered by specific environmental stimuli. Example of the latter is the smiling of a patient only when looking at his/her mother and not other persons. The responses of these patients are typically inconsistent. That is, as the behaviour of interest may occur infrequently or may be ambiguous, serial assessment is often required to capture signs of awareness. The heterogeneity of MCS has been recently recognized, and it has been proposed to subcategorize this entity into MCS PLUS and MINUS [18]. The differentiation was based on the level of complexity of the observed behavioural responses, such as the ability to understand language and therefore to follow simple commands [19]. Patients may emerge from MCS (EMCS) once they regain the ability to reliably communicate and/or use objects in a functional manner [16]. The temporal limits of irreversibility have not been proposed yet for MCS. Although there is some evidence suggesting that patients in a MCS have better chances of recovery than patients in VS/UWS, at present we are not in a position to possibly refer to chronic MCS [20]. Some patients, mainly after a focal brainstem injury, may evolve from coma to a locked-in syndrome (LIS). This is not properly speaking a DOC; however, it is worth to be mentioned in this context, as it is easily and often misdiagnosed as a DOC. This clinical entity results from a lesion in the brainstem extensively impairing the cortico-spinal and cortico-bulbar tracts, which classically leads to complete paralysis of voluntary muscles except for oculomotor muscles in a fully regained consciousness. These patients, therefore, are able to communicate solely through their eyelids movements [21]. Rarely, all voluntary muscles are impaired, including extrinsic eye muscles (complete LIS) making even more challenging the diagnosis of this patient category [22].
Neural Correlates of Awareness
Studies using conventional brain structural imaging have shown highly variable and heterogeneous results in patients with DOC, suggesting that awareness cannot be unequi vocally related to a specific brain region [23]. Nonetheless, neuropathological findings seem to associate VS/UWS to profound damage to the subcortical white matter and the major relay nuclei of the thalamus [24, 25], supporting a role of the thalamus and cerebral cortex in the genesis of awareness. Using positron emission tomography (PET) during resting state, it has been shown that awareness is not related to a global brain metabolism [26], but instead to the preservation of a large-scale fronto-parietal network encompassing the polymodal associative cortices [27], and its connections with thalamic nuclei. This was suggested by the recovery of this thalamo-cortical activity in a VS/UWS patient who had recovered consciousness [26]. In VS/UWS, where awareness is impaired while wakefulness is spared, PET studies showed that patients were characterized by a reduced global metabolism compared with healthy subjects, but notably, the recovery of consciousness did not necessarily coincide with resumption of global metabolic activity [17]. Resting-state PET voxel-based studies have further demonstrated that impairment of awareness was related to impairments in specific brain areas, consisting of a large-scale fronto-parietal network encompassing the polymodal associative cortices (Fig. 43.2) [27]. In addition, a peculiar disconnection between primary sensory areas and higher order associative cortices, which are thought to be required for conscious perception [4], has been demonstrated in VS/UWS patients during passive auditory and noxious stimulations [28]. In contrast, function in known arousal structures including the reticular formation in the brainstem, the hypothalamus and the basal forebrain appeared to remain relatively intact in patients with VS/UWS [10]. In this context, recent PET studies during resting-state and passive paradigms have detected relative hyperactivity in the reticular formation of the brainstem in VS/UWS in contrast to hypo-activity between the reticular formation and the precuneus compared to controls, suggesting the existence of a functional link between the arousal system and the associative cortices in the genesis of perception awareness [29]. In line with their clinical condition, MCS patients showed a partial preservation of this large-scale associative fronto-parietal network [30]. More important, PET studies employing passive noxious stimuli have elicited the activation of association areas related to pain processing in MCS patients in a similar network as in normal controls, suggesting a potential pain perception capacity in this patient category [31]. Similar findings of fronto-parietal deactivation have been found in other conditions of impaired awareness, such as in somnambulism as well as in absence and complex partial (mainly in the temporal lobe) seizures [7–9], whereas temporal lobe seizures without loss of consciousness were not accompanied by these widespread changes [8]. The above-mentioned studies have suggested a key role of an extensive fronto-parietal associative cortex for the emergence of awareness. The higher order associative fronto-parietal network mentioned above, commonly impaired during altered states of consciousness, has been recently functionally subdivided into two different networks: external awareness and internal awareness network [32]. The first one, also called task-positive network, is a lateral fronto-parietal network routinely exhibiting activity increases during attention-demanding cognitive tasks. The second, better known as default mode network (DMN), is a mesial fronto-parietal network commonly involved in self-related processes [33]. These two networks are functionally anti-correlated, (that is, when one network is activated the other one is not, and vice versa) and clearly linked to spontaneous mentation [32]. Several studies using even-related functional magnetic resonance imaging (fMRI) experiments have, in fact, demonstrated that awareness of the environment and awareness of the self have different anatomical substrates, namely the external and internal awareness network, respectively. For example, by giving subjects somatosensory laser stimuli on the back of their hand, it has been shown that at a certain intensity, one stimulus could be perceived only when the external awareness network was activated. Conversely, a stimulus at the same intensity could not be consciously perceived when the internal awareness network was activated, suggesting a role for the baseline brain activity fluctuations in the consciousness perception of the external world [34]. A number of auto-referential stimuli, furthermore, pointed to the critical role of the midline structures (DMN) for self or internal awareness [35–38]. In addition, the decreased anti-correlation of these two networks in unconscious states, such as anaesthesia [6], deep sleep [39] and DOC patients [40, 41], suggests somehow the functional contribution of this anti-correlated pattern to conscious cognition [42]. Frequently and increasingly used in the analysis of DOC, resting-state fMRI is a non-invasive technique which investigates the spontaneous temporal coherence in blood oxygen level-dependent (BOLD) fluctuations related to the amount of synchronized neural activity existing between different location of the brain (i.e. functional connectivity or correlation) in the absence of input or output tasks [43]. Besides the external and internal awareness networks mentioned above, several other resting-state networks of high spatial consistency across subjects have been detected, such as the auditory network, visual network, salience network and cerebellum network [44]. Among those, the DMN is the one that has mostly attracted the scientific attention. The DMN, in fact, has been thought to be implicated in cognitive processes and consciousness, probably because of its link to internally oriented cognitive content, such as mind wandering and autobiographical memory recall [45–47]. Recent resting-state fMRI studies have detected decreased DMN connectivity in patients in coma, VS/UWS and MCS [48]. Such reduced connectivity was shown to be correlated to the level of consciousness, mostly affecting the precuneus, a brain area considered to be a critical hub within this network [10]. Similar decreases in DMN connectivity were observed in healthy controls during sedation, general anaesthesia [6] and deep sleep [49]. However, other studies have shown the persistence of coherent DMN connectivity in some patients with VS/UWS as well as in a case of anesthetized monkey [50], in contrast with its complete absence in a case of brain death [41]. The above evidence suggests that the DMN may not be a mere reflection of conscious mental activity and that other phenomena may indeed play a role in the relation between consciousness and the DMN. For example, it was recently reported that along with decreased DMN connectivity, DOC patients showed an increased “pathological” correlation, compared to healthy controls, between the DMN and regions belonging to the external awareness network (hence normally anti-correlated in conscious controls), such as the insula and dorsolateral prefrontal cortex (Fig. 43.3), and stronger in VS/UWS than in MCS patients [51]. This pathological hyper-connectivity suggests the role of a network unbalance sustaining unconsciousness possibly caused by a natural brain topology reorganization strategy of functional connectivity [51]. Taking this into account, hypo–connectivity is therefore only an aspect of a possibly multifaceted and more complex dysfunctional architecture of brain connectivity in altered consciousness, and not necessarily the global hallmark of these conditions. This being also recently supported by the detection of hyper-connectivity patterns also in states of pharmacological coma, such as general anaesthesia [52]. Recently, more networks at resting state have been investigated in DOC, such as the bilateral fronto-parietal or executive control networks, salience, sensorimotor, auditory, visual systems and the cerebellar network. It was found that besides the DMN, the bilateral executive control networks and the auditory system were also significantly less identifiable (in terms of spatial and neural properties) in patients with DOC compared to healthy controls and showed consciousness-level-dependent decreases in functional connectivity across the spectrum of DOC. Furthermore, with machine learning classification trained on the identification of these ten networks as neuronal or not, it was even possible to separate healthy controls from patients in DOC and VS/UWS with high accuracy [53, 54]. One of the near-perspective future challenges will be to interpret resting-state patterns according to studied population in order to unravel the relationship (correlation and anti-correlation) between the resting-state networks in different level of consciousness, and to better comprehend its functional and clinical meaning in general and at also at single-subject level, leading to a more accurate diagnosis [42].



Fig. 43.2
Brain areas where metabolism is impaired in vegetative/unresponsive wakefulness syndrome (VS/UWS) patients compared to controls (areas in red), superimposed in a 2D image, axial, coronal and sagittal view. FWE p 0.05 corrected

Fig. 43.3
In vegetative state/unresponsive wakefulness syndrome (VS/UWS), the anterior cingulate cortex (ACC) and posterior cingulate cortex (PCC) are hypo-connected to the default mode network (DMN, in blue) and hyper-connected to the fronto-insular cortex (in red). Superimposed on a 3D template, lateral and mesial sagittal view. Correlation from random effect (p < 0.01) and clustered corrected (p < 0.05) results based on general linear model maps with seed region of interest comparing VS/UWS to healthy controls. Adapted from [51]
Functional Neuroimaging and Detection of Awareness in Disorders of Consciousness
Detection of potential awareness in uncommunicative brain-damaged patients raises important ethical and medical concerns, regarding end-of-life decisions and palliative treatment [5]. Clinicians are offered with various clinical scales to detect sings of awareness at the bedside [55]. The Coma Recovery Scale-Revised (CRS-R) [17] is one of the most sensitive tools to diagnose and differentiate between patients in VS/UWS and MCS because it assesses all the defining criteria for MCS, such as visual pursuit and command following [56]. Nonetheless, not only is it made of different behavioural components, but it also includes directives to look for these behaviours. For example, when visual pursuit was tested by means of a moving object, a moving person and a moving mirror, more patients tracked their image in the mirror compared to the other two stimuli and were hence considered to be in a MCS [57]. Similarly, to score sound localization with the CRS-R, the patients need to orient their head or eyes towards the source of the sound. When the patients’ own names were used, more patients oriented their head or eyes towards the examiner compared to the meaningless sound of a ringing bell [58]. These studies imply that self-referential stimuli are more effective to explore patients’ responsiveness and can influence the diagnostic process [also see 59]. Nevertheless, it is worth stressing that what one can actually assess at the bedside of the patient is responsiveness. As responsiveness represents only an indirect evidence of consciousness (i.e. the lack of responsiveness does not necessarily imply lack of consciousness) reliance on these behavioural markers presents significant challenges and may lead to misdiagnoses. Clinical studies have shown that up to 40 % of patients with a diagnosis of VS/UWS may in fact retain some level of awareness [60–62], and the main causes of misdiagnosis are associated with patient’s disabilities, such as paralysis and aphasia, fluctuation in arousal level, difficulty differentiating reflexive from involuntary movements, the presence of drugs’ side effects and the non-use of standardized and sensitive clinical scales such as the CRS-R. One way to approach the problem is to detect awareness independently of responsiveness, that is to measure patients’ brain responses and activation during sensitive experimental manipulations (which do not require motor responsiveness) and compare them with that of healthy controls. If the cerebral activation pattern is indistinguishable between the two groups, then one has good reasons to believe that the extracted statistical maps reflect the same construct [63]. Possible concerns about the degree of confidence on functional neuroimaging results are recently raised, especially when one considers that it is not possible to have subjective reports by the patients [e.g. 64]. In addition, our limited understanding of the complex dynamic neuronal mechanisms underlying consciousness and its reluctance to quantification in the absence of communication [65] makes it difficult to establish any strong claim about consciousness in non-communicating patients. Nevertheless, the use of these technologies (such as neuroimaging and electrophysiology) has shed light on the grey zones between the different clinical entities of consciousness and has revealed that not all patients considered as VS/UWS are actually unaware [20, 66]. Due to the excellent spatial resolution that is offered by functional magnetic resonance (fMRI) and PET, here we will focus on studies employing these neuroimaging methods referring to the main paradigms used to detect residual consciousness in patients with DOC: active and passive paradigms.
Active paradigms refer to mental imagery tasks which measure wilful modulation of brain signal in specific brain areas, aiming to detect ability to follow command. Command-following abilities in patients with DOC is of major importance as its presence or not can distinguish, respectively, the clinical entities of MCS and VS/UWS, with related ethical and medical issues. Hence, active paradigms in fMRI can be used to detect potential command-following abilities in those patients that are not able to show it through their motor behaviour, improving possible misdiagnosis in this category of patients that could occur when based only on the clinical approach.
In the fMRI setting, two main paradigms have been shown to activate robustly and reproducibly specific brain regions, namely imaging of playing a tennis match (encompassing mainly supplementary motor areas) and imaging visiting his/her own house (encompassing mainly parahippocampal cortex) [67]. When these paradigms were employed in a behaviourally unresponsive patient, the activation pattern of this patient was indistinguishable from that in a healthy control, showing that this patient was actually capable of understanding and following these mental imagery commands [67]. Thus, the behavioural diagnosis was challenged and the patient was no longer considered to be in a VS/UWS. Moreover, a few months after the fMRI finding of activation pattern, the same patient emerged out of the VS/UWS also clinically. We can say, therefore, that in this case functional imaging could detect signs of awareness in the patient earlier than the bedside clinical assessment. Nevertheless, this was the first evidence of dissociation between extreme behavioural motor impairment and identifiable preserved higher cognitive abilities detectable only by functional imaging techniques. A further study examined this phenomenon on a much larger cohort of patients, aiming at detecting the frequency of preserved awareness in behaviourally unresponsive patients [68]. It turned out that of 54 patients with DOC, five (3 VS/UWS and 2 MCS—two which did not show any signs of consciousness at behavioural assessment) were able to wilfully modulate their brain activity; furthermore, one behaviourally diagnosed VS/UWS patient was able to use this technique to communicate by correctly responding with yes (by imagining playing tennis) or no (by imagining visiting the rooms of his house) to autobiographical questions during the fMRI scanning [68]. Thus, this study showed that the case described by Owen et al. in 2006 was not an isolated case, and that approximately 17 % of patients diagnosed as in VS/UWS after behavioural assessment can follow commands when such commands showed a change in BOLD response, rather than overt motoric behaviour. Other mental tasks for detecting response to command in patients with DOC have been employed. For example, by employing fMRI during covert picture naming as a command-following task in a cohort of patients with DOC, complete network expected for the task as in normal controls was evidenced in the patient with LIS, in two of five MCS patients and in one of two VS/UWS patients [69]. By instructing patients behaviourally considered in a VS/UWS to move their hand, Bekischtein et al. [70] detected activation in the premotor cortex contralateral to the instructed hand, consistent with movement preparation. Similarly, by employing a hierarchical mental imagery (imaging playing tennis and swimming) fMRI approach, Bardin et al. [71] detected residual cognitive capacities in patients with DOC, confirming the dissociation between bedside and fMRI-based command-following and communication abilities. More recently, a further study using selective auditory attention showed that three behaviourally unresponsive patients (two MCS and one VS/UWS) were able to convey their ability to follow commands, and one in VS/UWS was even able to use attention to correctly communicate answers to several binary questions [72], pointing out how different tasks, which allow to overcome the motor unresponsiveness of brain-injured patients by tackling different cognitive aspects, might detect residual covert awareness in this patient category. However, legitimate concerns about the degree of confidence in these methods have emerged. Mental imagery paradigms rely on the fact that a patient can understand the commands and is able to wilfully execute them. In fact, there are many cases when this is not possible, even if the patient retains some degree of consciousness. For example, a patient might not understand the task because he is deaf or aphasic, a patient might not be compliant due to the side effect of medications interfering with vigilance or motor ability or simply because he is sleepy at the moment of the mental imagery task, given that the fluctuation level of vigilance is very often shown in several cases of MCS. Indeed, out of 31 MCS patients described in the study by Monti et al. [68], only one was able to wilfully modulate his brain activity, and out of six cases of behaviourally MCS described by Bardin, only four showed contingent brain activation in the fMRI. Hence, it is worth stressing that absence of command-related brain activation does not allow to infer that awareness is not present [71], and this could lead to possible false-negative results [73]. In order to overcome some of the above-mentioned limitations, residual cognitive function in patients with DOC can be further assessed employing passive and resting-state paradigms.
Passive paradigms measure brain responses to external sensory stimulation (e.g. auditory, somatosensory and visual) while the subject is not performing any task. As previously mentioned, the administration of auditory stimuli in VS/UWS patients elicited PET activation in the primary auditory cortex of these patients, whereas a wider spread of activation in the secondary auditory cortex and associative cortices, contingent to their clinical condition, was detected in MCS patients [74]. By using PET, painful stimulation of the median nerve elicited the entire ‘pain matrix’ (including the anterior cingulate cortex and insular areas) in patients with MCS, whereas only activation in the lower level subcortical areas was detected in VS/UWS patients [28, 31], indicating that MCS patients are more likely to experience the administered stimuli as painful, with related practical and clinical issues regarding the use of painkillers in this patient category. Similarly, in a passive visual fMRI task, that is presenting pictures of different emotional valences, visual activation similar to healthy controls was found in MCS patients [75]. More recently, a visual cognition study applied to a MCS patient showed brain activations undistinguishable from those observed in healthy and aware controls, suggesting that the patient retained the ability to access his own visual representations [76]. The above studies once again confirm the importance of the associative areas for the emergence of awareness and showed an overlap between clinical and neuroimaging findings. However, the possible dissociation between clinical and neuroimaging assessment has been also shown by means of passive paradigms. For example, a study combining PET and fMRI and using a more complex auditory task (manipulating words at different levels of auditory intelligibility and semantic ambiguity) detected a residual auditory and speech processing in a patient behaviourally considered to be in a VS/UWS [77]. Also, a passive auditory fMRI study using narratives played forward and backwards showed one of three patients in a VS/UWS, and one of four MCS patients showed similar brain activation as that elicited in normal controls [78]. Another salient auditory stimulus which has been preferred because of its attention-grabbing properties is the patient’s own name. It has been in fact reported that the subject’s own name (SON) activated the cerebral cortex more extensively than non-self-referential emotional stimuli in patients with MCS [30] and the SON spoken by a familiar voice (SON-FV), as opposed to an unfamiliar voice, elicited stronger event-related potential (ERP) responses [79]. Furthermore, it is shown that the SON showed higher sensitivity to elicit sound localization reflex in DOC patients [58]. The SON-FV, therefore, has been used in order to maximize the chances of detecting residual brain function in DOC patients using fMRI. With a SON-FV paradigm, two of seven patients in UWS and all four patients in MCS not only showed activation of the primary auditory cortex but also in higher order associative auditory areas, which are thought to be implicated in the conscious processing of the incoming stimuli. Notably, these two patients in UWS subsequently recovered to MCS, as observed three months after their fMRI scan. This pointed out the possible prognostic value of such fMRI paradigm [80]. Taken together, these paradigms have shown that auditory, visual and somatosensory activation is restricted to lower level sensory regions in patients in UWS, while brain activation is widespread in MCS reaching higher level associative areas. In some cases, they have shown activation in higher level associative areas in patients behaviourally diagnosed as VS/UWS, suggesting the potential of this tool to detect covert awareness in behaviourally unresponsive patients or, at least in some cases, before the clinical manifestations of the recovery. The passive paradigms can often overcome some limitation of the previously described active task, such as the possibility that a patient is aphasic and therefore unable to understand a task. However, in other cases, such as when a patient has sensory deficits (e.g. deafness or impaired vigilance possibly related to drug side effects), these paradigms might bring false-negative results and other acquisitions should be used, such as the resting-state fMRI. The use of neuroimaging techniques during resting state in patients with DOC has been more specifically described in the previous paragraph.
Electroencephalography (EEG) and transcranial magnetic stimulation (TMS)
Like fMRI, EEG recordings in patients with DOC can evaluate different aspects of cognitive residual function and provide means to communicate with the outside world without motor output. Standard recordings in the neurological department offer a first global view of the brain function of a patient and can detect abnormal activity and therefore guide treatment [81]. In resting conditions, various EEG paradigms have made an effort to differentiate between the clinical entities of patients with DOC. After a severe brain injury, of whatever origin, the EEG can be altered and may display abnormalities. A visible common effect is a slowing of the brain activity proportional to the severity of the injury. Therefore, the predominant rhythm is no longer posterior alpha (related to the awake stages in healthy adult individuals) but diffuse theta or delta (normally present in the slow stages of sleep in healthy adult individuals). In some cases, alpha or theta activity can be observed, but its activity differs from a normal adult alpha activity [82]. Measures of signal complexity such as the bispectral index (a measure of the depth of anaesthesia) were shown to discriminate between unresponsive and MCS patients at the group level [83]. The bi-spectral index was also positively correlated with behavioural scores of awareness at the time of testing and was associated with outcome at one-year post-trauma. However, at the single-subject level, establishing a diagnosis solely based on a single standard EEG is difficult since the patterns are not specific to the aetiology and the same subject can have different patterns in a short interval of time. A study based on patients in persistent VS/UWS concluded that it was not possible to reliably use EEG as a diagnostic tool due to its heterogeneous and varying aspects [84]. Despite the limited diagnostic role of standard EEG recordings, prognostic statements are possible but challenging as the same pattern can be found in varying brain pathologies. EEG information needs to be constantly integrated by the clinical information in order to have plausible insights into the prognosis [81]. In this context, active command mental paradigms combined with EEG [85–87] appear to be useful in the diagnosis of DOC. They have, in fact, allowed both detection of voluntary brain function in VS/UWS and functional communication in patients with complete LIS. A recent study showed that out of 16 studied patients, three were in VS/UWS on the basis of repeated specialist behavioural assessments, and were found to be aware and capable of substantially and consistently modulating their EEG responses upon command [87]. However, as pointed out above, no diagnostic information can be inferred from a negative result, as it does not necessarily imply the lack of consciousness. Indeed, patients behaviourally in a MCS might still not be able to understand and follow instructions. In this context, EEG combined with TMS (TMS/EEG) may be especially useful to assess the level of consciousness in DOC patients, because it does not rely on a subject’s ability to process sensory stimuli, or to understand and follow instructions. TMS/EEG allows to stimulate a subset of cortical neurons in a non-invasive way, and to measure the effects produced by this perturbation in the whole brain [88, 89]. For patients in VS/UWS, when stimulating a superficial region of the cerebral cortex, TMS induced either no response or a simple response and a local EEG response, indicating an impairment of effective connectivity [90, 91], similar to the one observed in deep sleep and anaesthesia [89, 92]. In contrast, for patients in MCS, TMS triggered more complex EEG activations that sequentially involved different cortical areas, similar to activations showed in healthy awake subjects. Of note, an MCS patient assessed during a period of no responsiveness still showed complex and widespread brain responses to TMS, even though he did not demonstrate any behavioural sign of consciousness during clinical assessment [91]. Furthermore, an empirical measure of brain complexity, the perturbational complexity index (PCI), which measures the amount of information contained in the integrated response to a direct TMS perturbation, has recently been introduced [88]. The PCI was tested on a large data set of TMS-evoked potentials recorded from healthy subjects during wakefulness, dreaming, non-rapid eye movement (NREM) sleep, and different levels of sedation induced by different anaesthetic agents as well as from brain-injured patients who had emerged from coma (overall, 208 sessions in 52 subjects). PCI provided a data-driven metric that can discriminate level of consciousness in single subjects under different conditions of consciousness: wakefulness; dreaming; the LIS; the MCS; the EMCS; intermediate levels of sedation; NREM sleep; midazolam-, xenon- and propofol-induced loss of consciousness; and the VS/UWS [88]. It appears that not a single unconscious subject (regardless of its aetiology) has a PCI above 0.31, and no awake healthy subject has a PCI below 0.44. This allows a single-subject approach, necessary for a clinical use of this tool. Because this technique is handy, not invasive, does not require patients’ cooperation and works at the single-subject level, it appears to be a promising tool for the diagnosis of patients with DOC [88].
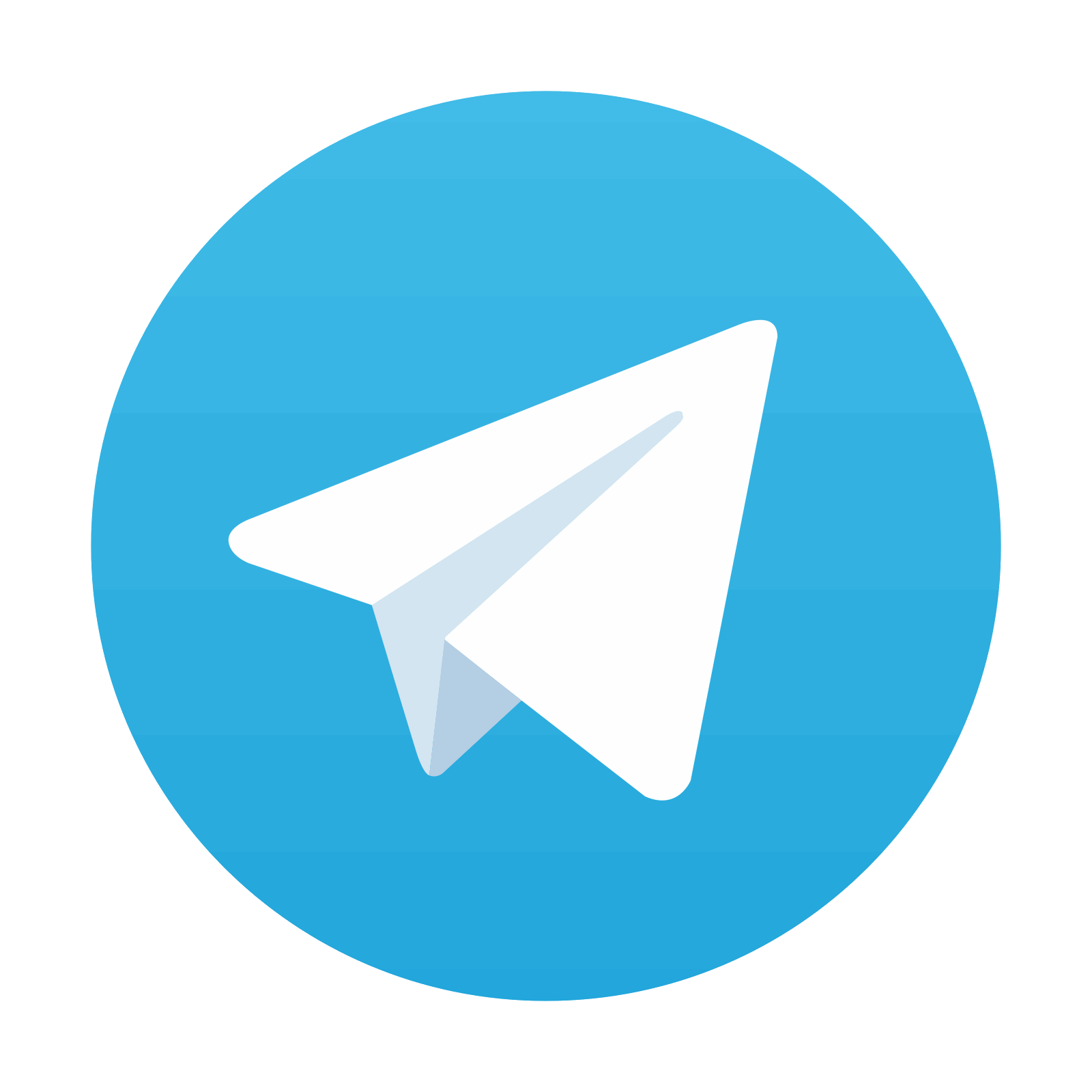
Stay updated, free articles. Join our Telegram channel

Full access? Get Clinical Tree
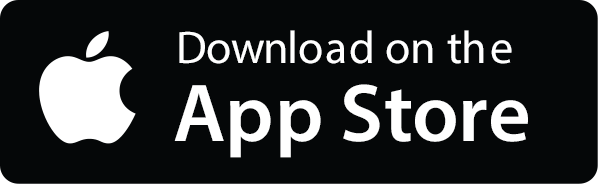
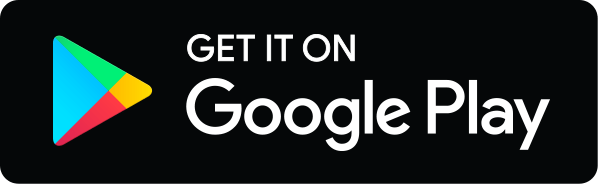