Disturbed sleep is among the most frequent health complaints that physicians encounter. More than one-half of adults in the United States experience at least intermittent sleep disturbance, and only 30% of adult Americans report consistently obtaining a sufficient amount of sleep. The Institute of Medicine has estimated that 50–70 million Americans suffer from a chronic disorder of sleep and wakefulness, which can adversely affect daytime functioning as well as physical and mental health. Over the last 20 years, the field of sleep medicine has emerged as a distinct specialty in response to the impact of sleep disorders and sleep deficiency on overall health.
Given the opportunity, most healthy young adults will sleep 7–8 h per night, although the timing, duration, and internal structure of sleep vary among individuals. In the United States, adults tend to have one consolidated sleep episode each night, although in some cultures sleep may be divided into a mid-afternoon nap and a shortened night sleep. This pattern changes considerably over the life span, as infants and young children sleep considerably more than older people.
The stages of human sleep are defined on the basis of characteristic patterns in the electroencephalogram (EEG), the electrooculogram (EOG—a measure of eye-movement activity), and the surface electromyogram (EMG) measured on the chin, neck, and legs. The continuous recording of these electrophysiologic parameters to define sleep and wakefulness is termed polysomnography.
Polysomnographic profiles define two basic states of sleep: (1) rapid eye movement (REM) sleep and (2) non–rapid eye movement (NREM) sleep. NREM sleep is further subdivided into three stages: N1, N2, and N3, characterized by increasing arousal threshold and slowing of the cortical EEG. REM sleep is characterized by a low-amplitude, mixed-frequency EEG similar to that of NREM stage N1 sleep. The EOG shows bursts of rapid eye movements similar to those seen during eyes-open wakefulness. EMG activity is absent in nearly all skeletal muscles, reflecting the brainstem-mediated muscle atonia that is characteristic of REM sleep.
Normal nocturnal sleep in adults displays a consistent organization from night to night (Fig. 24-1). After sleep onset, sleep usually progresses through NREM stages N1–N3 sleep within 45–60 min. NREM stage N3 sleep (also known as slow-wave sleep) predominates in the first third of the night and comprises 15–25% of total nocturnal sleep time in young adults. Sleep deprivation increases the rapidity of sleep onset and both the intensity and amount of slow-wave sleep.
FIGURE 24-1
Wake-sleep architecture. Alternating stages of wakefulness, the three stages of NREM sleep (N1–N3), and REM sleep (solid bars) occur over the course of the night for representative young and older adult men. Characteristic features of sleep in older people include reduction of N3 slow-wave sleep, frequent spontaneous awakenings, early sleep onset, and early morning awakening. NREM, non–rapid eye movement; REM, rapid eye movement. (From the Division of Sleep and Circadian Disorders, Brigham and Women’s Hospital.)

The first REM sleep episode usually occurs in the second hour of sleep. NREM and REM sleep alternate through the night with an average period of 90–110 min (the “ultradian” sleep cycle). Overall, in a healthy young adult, REM sleep constitutes 20–25% of total sleep, and NREM stages N1 and N2 constitute 50–60%.
Age has a profound impact on sleep state organization (Fig. 24-1). N3 sleep is most intense and prominent during childhood, decreasing with puberty and across the second and third decades of life. N3 sleep declines during adulthood to the point where it may be completely absent in older adults. The remaining NREM sleep becomes more fragmented, with many more frequent awakenings from NREM sleep. It is the increased frequency of awakenings, rather than a decreased ability to fall back asleep, that accounts for the increased wakefulness during the sleep episode in older people. While REM sleep may account for 50% of total sleep time in infancy, the percentage falls off sharply over the first postnatal year as a mature REM-NREM cycle develops; thereafter, REM sleep occupies about 25% of total sleep time.
Sleep deprivation degrades cognitive performance, particularly on tests that require continual vigilance. Paradoxically, older people are less vulnerable to the neurobehavioral performance impairment induced by acute sleep deprivation than young adults, maintaining their reaction time and sustaining vigilance with fewer lapses of attention. However, it is more difficult for older adults to obtain recovery sleep after staying awake all night, as the ability to sleep during the daytime declines with age.
After sleep deprivation, NREM sleep is generally recovered first, followed by REM sleep. However, because REM sleep tends to be most prominent in the second half of the night, sleep truncation (e.g., by an alarm clock) results in selective REM sleep deprivation. This may increase REM sleep pressure to the point where the first REM sleep may occur much earlier in the nightly sleep episode. Because several disorders (see below) also cause sleep fragmentation, it is important that the patient have sufficient sleep opportunity (at least 8 h per night) for several nights prior to a diagnostic polysomnogram.
There is growing evidence that sleep deficiency in humans may cause glucose intolerance and contribute to the development of diabetes, obesity, and the metabolic syndrome, as well as impaired immune responses, accelerated atherosclerosis, and increased risk of cardiac disease and stroke. For these reasons, the Institute of Medicine declared sleep deficiency and sleep disorders “an unmet public health problem.”
Two principal neural systems govern the expression of the sleep and wakefulness. The ascending arousal system, illustrated in green in Fig. 24-2, consists of clusters of nerve cells extending from the upper pons to the hypothalamus and basal forebrain that activate the cerebral cortex, thalamus (which is necessary to relay sensory information to the cortex), and other forebrain regions. The ascending arousal neurons use monoamines (norepinephrine, dopamine, serotonin, and histamine), glutamate, or acetylcholine as neurotransmitters to activate their target neurons. Additional arousal-promoting neurons in the hypothalamus use the peptide neurotransmitter orexin (also known as hypocretin, shown in blue) to reinforce activity in the other arousal cell groups.
FIGURE 24-2
Relationship of drugs for insomnia with wake-sleep systems. The arousal system in the brain (green) includes monoaminergic, glutamatergic, and cholinergic neurons in the brainstem that activate neurons in the hypothalamus, thalamus, basal forebrain, and cerebral cortex. Orexin neurons (blue) in the hypothalamus, which are lost in narcolepsy, reinforce and stabilize arousal by activating other components of the arousal system. The sleep-promoting system (red) consists of GABAergic neurons in the preoptic area, lateral hypothalamus, and brainstem that inhibit the components of the arousal system, thus allowing sleep to occur. Drugs used to treat insomnia include those that block the effects of arousal system neurotransmitters (green and blue) and those that enhance the effects of γ-aminobutyric acid (GABA) produced by the sleep system (red).

Damage to the arousal system at the level of the rostral pons and lower midbrain causes coma, indicating that the ascending arousal influence from this level is critical in maintaining wakefulness. Damage to the hypothalamic branch of the arousal system causes profound sleepiness, but usually not coma. Specific loss of the orexin neurons produces the sleep disorder narcolepsy (see below).
The arousal system is turned off during sleep by inhibitory inputs from cell groups in the sleep-promoting system, shown in Fig. 24-2 in red. These neurons in the preoptic area, lateral hypothalamus, and pons use γ-aminobutyric acid (GABA) to inhibit the arousal system. Many sleep-promoting neurons are themselves inhibited by inputs from the arousal system. This mutual inhibition between the arousal- and sleep-promoting systems forms a neural circuit akin to what electrical engineers call a “flip-flop switch.” A switch of this type tends to promote rapid transitions between the on (wake) and off (sleep) states, while avoiding intermediate states. The relatively rapid transitions between waking and sleeping states, as seen in the EEG of humans and animals, is consistent with this model.
Neurons in the ventrolateral preoptic nucleus, one of the key sleep-promoting sites, are lost during normal human aging, correlating with reduced ability to maintain sleep (sleep fragmentation). The ventrolateral preoptic neurons are also injured in Alzheimer’s disease, which may in part account for the poor sleep quality in those patients.
Transitions between NREM and REM sleep appear to be governed by a similar switch in the brainstem. GABAergic REM-Off neurons have been identified in the lower midbrain that inhibit REM-On neurons in the upper pons. The REM-On group contains both GABAergic neurons that inhibit the REM-Off group (thus satisfying the conditions for a REM flip-flop switch) as well as glutamatergic neurons that project widely in the central nervous system (CNS) to cause the key phenomena associated with REM sleep. REM-On neurons that project to the medulla and spinal cord activate inhibitory (GABA and glycine-containing) interneurons, which in turn hyperpolarize the motor neurons, producing the atonia of REM sleep. REM-On neurons that project to the forebrain may be important in producing dreams.
The REM sleep switch receives cholinergic input, which favors transitions to REM sleep, and monoaminergic (norepinephrine and serotonin) input that prevents REM sleep. As a result, drugs that increase monoamine tone (e.g., serotonin or norepinephrine reuptake inhibitors) tend to reduce the amount of REM sleep. Damage to the neurons that promote REM sleep atonia can produce REM sleep behavior disorder, a condition in which patients act out their dreams (see below).
The gradual increase in sleep drive with prolonged wakefulness, followed by deeper slow-wave sleep and prolonged sleep episodes, demonstrates that there is a homeostatic mechanism that regulates sleep. The neurochemistry of sleep homeostasis is only partially understood, but with prolonged wakefulness, adenosine levels rise in parts of the brain. Adenosine may act through A1 receptors to directly inhibit many arousal-promoting brain regions. In addition, adenosine promotes sleep through A2a receptors; inhibition of these receptors by caffeine is one of the chief ways in which people fight sleepiness. Other humoral factors, such as prostaglandin D2, have also been implicated in this process. Both adenosine and prostaglandin D2 activate the sleep-promoting neurons in the ventrolateral preoptic nucleus.
Allostasis is the physiologic response to a threat that cannot be managed by homeostatic mechanisms (e.g., the presence of physical danger or psychological threat). These stress responses can severely impact the need for and ability to sleep. For example, insomnia is very common in patients with anxiety and other psychiatric disorders. Stress-induced insomnia is even more common, affecting most people at some time in their lives. Positron emission tomography (PET) studies in patients with chronic insomnia show hyperactivation of the components of the ascending arousal system, as well as their targets in the limbic system in the forebrain (e.g., cingulate cortex and amygdala). The limbic areas are not only targets for the arousal system, but they also send excitatory outputs back to the arousal system, which contributes to a vicious cycle of anxiety about wakefulness that makes it more difficult to sleep. Approaches to treating insomnia rely on drugs that either inhibit the output of the ascending arousal system (green and blue in Fig. 24-2) or potentiate the output of the sleep-promoting system (red in Fig. 24-2). However, behavioral approaches (cognitive behavioral therapy and sleep hygiene) that may reduce forebrain limbic activity at bedtime are often equally or more successful.
Sleep is also regulated by a strong circadian timing signal, driven by the suprachiasmatic nuclei (SCN) of the hypothalamus, as described below. The SCN sends outputs to key sites in the hypothalamus, which impose 24-h rhythms on a wide range of behaviors and body systems, including the wake-sleep cycle.
The wake-sleep cycle is the most evident of many 24-h rhythms in humans. Prominent daily variations also occur in endocrine, thermoregulatory, cardiac, pulmonary, renal, immune, gastrointestinal, and neurobehavioral functions. At the molecular level, endogenous circadian rhythmicity is driven by self-sustaining transcriptional/translational feedback loops. In evaluating daily rhythms in humans, it is important to distinguish between diurnal components passively evoked by periodic environmental or behavioral changes (e.g., the increase in blood pressure and heart rate that occurs upon assumption of the upright posture) and circadian rhythms actively driven by an endogenous oscillatory process (e.g., the circadian variations in adrenal cortisol and pineal melatonin secretion that persist across a variety of environmental and behavioral conditions).
While it is now recognized that most cells in the body have circadian clocks that regulate diverse physiologic processes, most of these disparate clocks are unable to maintain the synchronization with each other that is required to produce useful 24-h rhythms aligned with the external light-dark cycle. The neurons in the SCN are interconnected with one another in such a way as to produce a near-24-h synchronous rhythm of neural activity that is then transmitted to the rest of the body. Bilateral destruction of the SCN results in a loss of most endogenous circadian rhythms including wake-sleep behavior and rhythms in endocrine and metabolic systems. The genetically determined period of this endogenous neural oscillator, which averages ~24.15 h in humans, is normally synchronized to the 24-h period of the environmental light-dark cycle through direct input from intrinsically photosensitive ganglion cells in the retina to the SCN. Humans are exquisitely sensitive to the resetting effects of light, particularly the shorter wavelengths (~460–500 nm) of the visible spectrum. Small differences in circadian period contribute to variations in diurnal preference in young adults (with the circadian period shorter in those who typically go to bed and rise earlier compared to those who typically go to bed and wake up later), whereas changes in homeostatic sleep regulation may underlie the age-related tendency toward earlier sleep-wake timing.
The timing and internal architecture of sleep are directly coupled to the output of the endogenous circadian pacemaker. Paradoxically, the endogenous circadian rhythm for wake propensity peaks just before the habitual bedtime, whereas that of sleep propensity peaks near the habitual wake time. These rhythms are thus timed to oppose the rise of sleep tendency throughout the usual waking day and the decline of sleep propensity during the habitual sleep episode, respectively. Misalignment of the endogenous circadian pacemaker with the desired wake-sleep cycle can, therefore, induce insomnia, decreased alertness, and impaired performance evident in night-shift workers and airline travelers.
Polysomnographic staging of sleep correlates with behavioral changes during specific states and stages. During the transitional state (stage N1) between wakefulness and deeper sleep, individuals may respond to faint auditory or visual signals. Formation of short-term memories is inhibited at the onset of NREM stage N1 sleep, which may explain why individuals aroused from that transitional sleep stage frequently lack situational awareness. After sleep deprivation, such transitions may intrude upon behavioral wakefulness notwithstanding attempts to remain continuously awake (see “Shift-Work Disorder,” below).
Awakenings from REM sleep are associated with recall of vivid dream imagery over 80% of the time, especially later in the night. Imagery may also be reported after NREM sleep interruptions. Certain disorders may occur during specific sleep stages and are described below under “Parasomnias.” These include sleepwalking, night terrors, and enuresis (bed wetting), which occur most commonly in children during deep (N3) NREM sleep, and REM sleep behavior disorder, which occurs mainly among older men who fail to maintain full atonia during REM sleep, and often call out, thrash around, or even act out entire dreams.
All major physiologic systems are influenced by sleep. Blood pressure and heart rate decrease during NREM sleep, particularly during N3 sleep. During REM sleep, bursts of eye movements are associated with large variations in both blood pressure and heart rate mediated by the autonomic nervous system. Cardiac dysrhythmias may occur selectively during REM sleep. Respiratory function also changes. In comparison to relaxed wakefulness, respiratory rate becomes slower but more regular during NREM sleep (especially N3 sleep) and becomes irregular during bursts of eye movements in REM sleep. Decreases in minute ventilation during NREM sleep are out of proportion to the decrease in metabolic rate, resulting in a slightly higher Pco2.
Endocrine function also varies with sleep. N3 sleep is associated with secretion of growth hormone in men, while sleep in general is associated with augmented secretion of prolactin in both men and women. Sleep has a complex effect on the secretion of luteinizing hormone (LH): during puberty, sleep is associated with increased LH secretion, whereas sleep in the postpubertal female inhibits LH secretion in the early follicular phase of the menstrual cycle. Sleep onset (and probably N3 sleep) is associated with inhibition of thyroid-stimulating hormone and of the adrenocorticotropic hormone–cortisol axis, an effect that is superimposed on the prominent circadian rhythms in the two systems.
The pineal hormone melatonin is secreted predominantly at night in both day- and night-active species, reflecting the direct modulation of pineal activity by a circuitous neural pathway that links the SCN to the sympathetic nervous system, which innervates the pineal gland. Melatonin secretion does not require sleep, but melatonin secretion is inhibited by ambient light, an effect mediated by the neural connection from the retina to the pineal gland via the SCN. Sleep efficiency is highest when the sleep episode coincides with endogenous melatonin secretion. Administration of exogenous melatonin can hasten sleep onset and increase sleep efficiency when administered at a time when endogenous melatonin levels are low, such as in the afternoon or evening or at the desired bedtime in patients with delayed sleep-wake phase disorder, but it does not increase sleep efficiency if administered when endogenous melatonin levels are elevated. This may explain why melatonin is often ineffective in the treatment of patients with primary insomnia.
Sleep is accompanied by alterations of thermoregulatory function. NREM sleep is associated with an increase in the firing of warm-responsive neurons in the preoptic area and a fall in body temperature; conversely, skin warming without increasing core body temperature has been found to increase NREM sleep. REM sleep is associated with reduced thermoregulatory responsiveness.
APPROACH TO THE PATIENT: Sleep Disorders
Patients may seek help from a physician because of: (1) sleepiness or tiredness during the day; (2) difficulty initiating or maintaining sleep at night (insomnia); or (3) unusual behaviors during sleep itself (parasomnias).
Obtaining a careful history is essential. In particular, the duration, severity, and consistency of the symptoms are important, along with the patient’s estimate of the consequences of the sleep disorder on waking function. Information from a bed partner or family member is often helpful because some patients may be unaware of symptoms such as heavy snoring or may underreport symptoms such as falling asleep at work or while driving. Physicians should inquire about when the patient typically goes to bed, when they fall asleep and wake up, whether they awaken during sleep, whether they feel rested in the morning, and whether they nap during the day. Depending on the primary complaint, it may be useful to ask about snoring, witnessed apneas, restless sensations in the legs, movements during sleep, depression, anxiety, and behaviors around the sleep episode. The physical exam may provide evidence of a small airway, large tonsils, or a neurologic or medical disorder that contributes to the main complaint.
It is important to remember that, rarely, seizures may occur exclusively during sleep, mimicking a primary sleep disorder; such sleep-related seizures typically occur during episodes of NREM sleep and may take the form of generalized tonic-clonic movements (sometimes with urinary incontinence or tongue biting) or stereotyped movements in partial complex epilepsy (Chap. 31).
It is often helpful for the patient to complete a daily sleep log for 1–2 weeks to define the timing and amounts of sleep. When relevant, the log can also include information on levels of alertness, work times, and drug and alcohol use, including caffeine and hypnotics.
Polysomnography is necessary for the diagnosis of several disorders such as sleep apnea, narcolepsy, and periodic limb movement disorder. A conventional polysomnogram performed in a clinical sleep laboratory allows measurement of sleep stages, respiratory effort and airflow, oxygen saturation, limb movements, heart rhythm, and additional parameters. A home sleep test usually focuses on just respiratory measures and is helpful in patients with a moderate to high likelihood of having obstructive sleep apnea. The multiple sleep latency test (MSLT) is used to measure a patient’s propensity to sleep during the day and can provide crucial evidence for diagnosing narcolepsy and some other causes of sleepiness. The maintenance of wakefulness test is used to measure a patient’s ability to sustain wakefulness during the daytime and can provide important evidence for evaluating the efficacy of therapies for improving sleepiness in conditions such as narcolepsy and obstructive sleep apnea.
Up to 25% of the adult population has persistent daytime sleepiness that impairs an individual’s ability to perform optimally in school, at work, while driving, and in other conditions that require alertness. Sleepy students often have trouble staying alert and performing well in school, and sleepy adults struggle to stay awake and focused on their work. More than half of Americans have fallen asleep while driving. An estimated 1.2 million motor vehicle crashes per year are due to drowsy drivers, causing about 20% of all serious crash injuries and deaths. One needn’t fall asleep to have an accident, as the inattention and slowed responses of drowsy drivers are a major contributor. Reaction time is equally impaired by 24 h of sleep loss as by a blood alcohol concentration of 0.10 g/dL.
Identifying and quantifying sleepiness can be challenging. First, patients may describe themselves as “sleepy,” “fatigued,” or “tired,” and the meanings of these words may differ between patients. For clinical purposes, it is best to use the term “sleepiness” to describe a propensity to fall asleep; whereas “fatigue” is best used to describe a feeling of low physical or mental energy but without a tendency to actually sleep. Sleepiness is usually most evident when the patient is sedentary, whereas fatigue may interfere with more active pursuits. Sleepiness generally occurs with disorders that reduce the quality or quantity of sleep or that interfere with the neural mechanisms of arousal, whereas fatigue is more common in inflammatory disorders such as cancer, multiple sclerosis (Chap. 45), fibromyalgia, chronic fatigue syndrome (Chap. 59), or endocrine deficiencies such as hypothyroidism or Addison’s disease. Second, sleepiness can affect judgment in a manner analogous to ethanol, such that patients may have limited insight into the condition and the extent of their functional impairment. Finally, patients may be reluctant to admit that sleepiness is a problem because they may have become unfamiliar with feeling fully alert and because sleepiness is sometimes viewed pejoratively as reflecting poor motivation or bad sleep habits.
Table 24-1 outlines the diagnostic and therapeutic approach to the patient with a complaint of excessive daytime sleepiness.
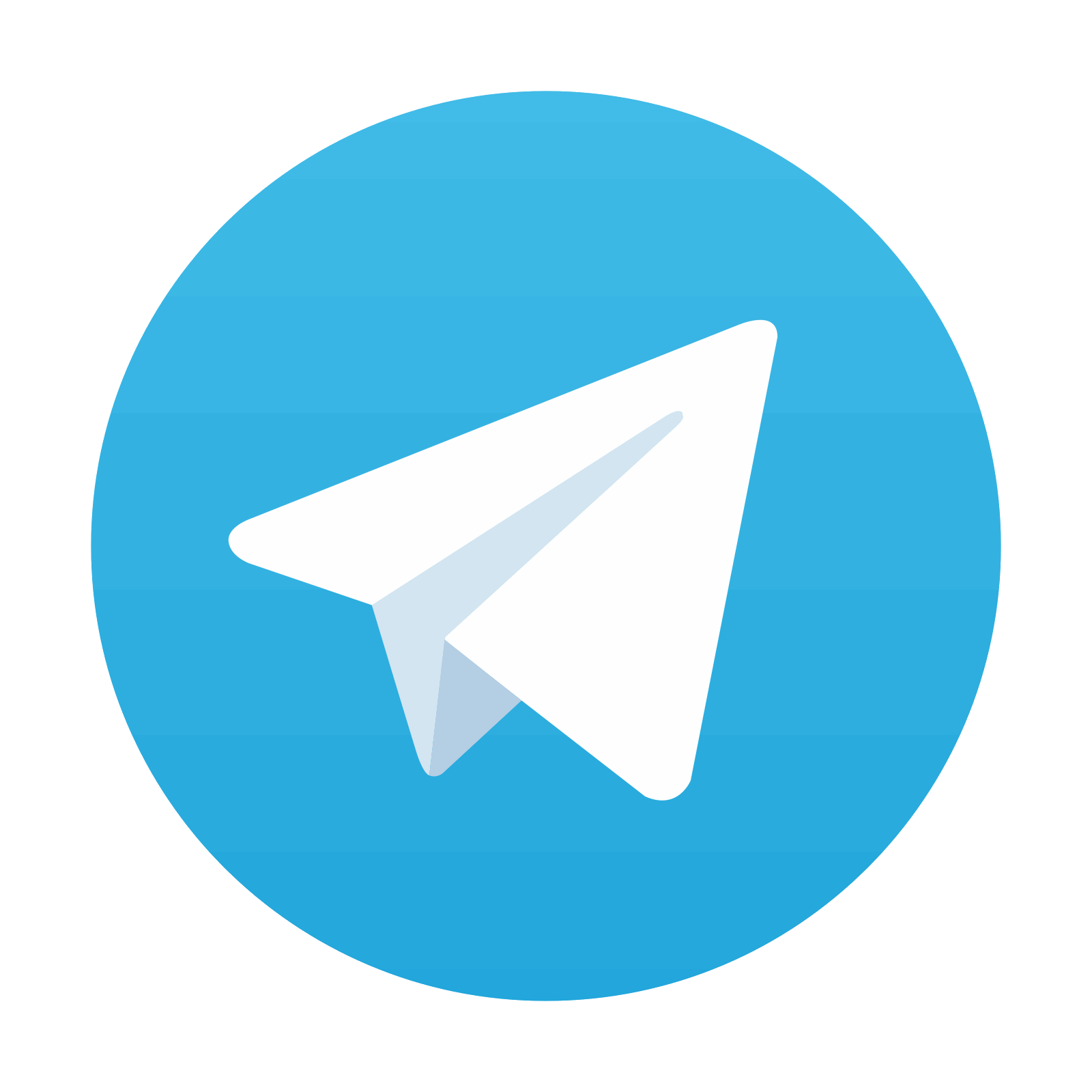
Stay updated, free articles. Join our Telegram channel

Full access? Get Clinical Tree
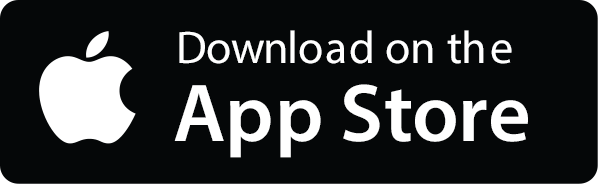
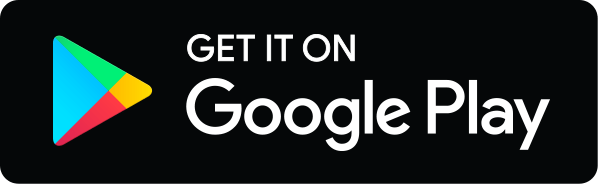