Wide neck circumference:
Men > 17 in.
Women > 16 in.
Enlarged nasal turbinates
Deviated nasal septum
Midfacial hypoplasia
Narrow mandible or maxilla
Dental overjet/retrognathia or micrognathia
Crossbite and dental malocclusion (class 2)
High and narrow hard palate
Elongated and low-lying uvula
High Mallampati score ≥3
Prominent tonsillar pillars
Enlarged tonsils and adenoids
Macroglossia
Steep thyromental plane and/or short thyromental distance
Pathophysiology of OSA Disorders
The basic mechanism of OSA involves an imbalance between the factors that promote airway collapse and the factors that promote airway patency [15]. In predisposed individuals with a small airway and/or sleep-related pharyngeal neuromuscular dysfunction, the normal loss of upper airway dilator muscle (e.g., genioglossus muscle) tone results in upper airway collapse followed by an absence of airflow despite persistent inspiratory muscle efforts of the diaphragm and the accessory respiratory muscles of the chest and neck. The progressively increasing negative inspiratory pressures mounted to overcome the upper airway resistance/collapse induce so-called respiratory effort-related arousals (RERAs). The reestablishment of airway patency then allows for the compensatory ventilation to correct hypercapnia and hypoxemia. As the individual reenters sleep, the cycle of intermittent partial or complete upper airway obstruction reoccurs multiple times during the sleep period. The recurrent arousals associated with abnormal respiratory events result in sleep fragmentation, reduced sleep efficiency, and reduced rapid eye movement (REM) sleep, all leading to daytime neurocognitive dysfunction. The intermittent hypoxemia and arousals associated with these obstructive respiratory events enhance catecholamine release, oxidative damage, systemic inflammation, endothelial dysfunction, insulin resistance, and hypercoagulability, which may be the basis for the increased risk of cardiovascular comorbidities [coronary heart disease (CHD), stroke, congestive heart failure (CHF), and arrhythmia] and metabolic dysfunction [16]. Sleep stage and body position also influence the severity of OSA. The atonia of the oropharyngeal dilator muscles and the accessory respiratory muscles during REM sleep facilitates upper airway collapse and reduces tidal volumes, respectively. The supine position causes upper airway narrowing by facilitating the direct posterior displacement of the soft palate, tongue, and jaw, and it reduces tidal volumes by enhancing the upward displacement of the diaphragm, particularly in obese individuals with increased abdominal girth.
Clinical Features of OSA Disorders
OSA disorders classically present with loud persistent snoring and breathing pauses during sleep, usually observed by a bed partner, roommate, or household member. The patient may report choking, gasping, gagging, and snoring causing awakenings. Unlike paroxysmal nocturnal dyspnea associated with CHF, asthma, or panic disorders, dyspnea following an obstructive apneic event resolves spontaneously within a few seconds. Neurocognitive manifestations include subjective and objective hypersomnolence as well as impairments in attention/vigilance, delayed long-term visual and verbal memory, visuospatial/constructional abilities, and executive function [17]. OSA increases the risk of motor vehicle accidents, especially in noncommercial drivers [18]. Symptoms of OSA disorders mimic those of clinical depression including depressed mood, irritability, reduced energy level, insomnia, impaired sex drive, etc. [19]. Nocturia may occur due to cardiac release of atrial natriuretic peptide in response to wide fluctuations in intrathoracic pressure during the obstructive apneic event [20]. Physical findings in OSAS include increased BMI (≥28 kg/m2), wide neck circumference (≥17 in. in men and ≥16 in. in women), and predisposing craniofacial features [Table 3.1]. The Mallampati score and Friedman tongue position score both correlate well with OSA severity [21]. Cardiopulmonary examination may reveal the presence of cardiovascular comborbidities such as CHF and COPD.
Cardiovascular complications that may develop during the course of untreated OSA disorders include systemic hypertension, CHD, CHF, arrhythmias, and stroke due to the resulting increased adrenergic activity (norepinephrine release), endothelial dysfunction (reduced flow-mediated dilation), increased pituitary–adrenal axis activity (increased serum ACTH and cortisol levels), systemic inflammation (elevated C-reactive protein and tumor necrosis factor-α levels), and hypercoagulability [16]. Diabetes mellitus type 2 commonly develops in up to 22–63 % of OSA patients depending upon the SRBD severity [22]. Other endocrine derangements associated with OSA include hypogonadism (reduced luteinizing hormone, total and free testosterone, and SHBG) [23, 24], hypercortisolism [25], and reduced plasma insulin-like growth factor (IGF-I) [26].
Diagnosis of OSA Disorders
Various tools have been validated for screening patients for OSAS (e.g., Epworth Sleepiness Scale, STOP-BANG, Berlin questionnaire, etc.). The STOP-BANG questionnaire, which assigns a point for each risk factor present, surpasses the others in terms of performance characteristics and simplicity of use as a screening tool [27]. The ICSD-3 diagnostic criteria for OSAS require the presence of characteristic nocturnal symptoms, neurocognitive dysfunction, mood disorder, and/or cardiovascular comorbidities plus a polysomnogram documenting an elevated respiratory disturbance index (RDI ≥ 5/h), which is calculated as the number of obstructive apneas, hypopneas, and RERAs per hour of sleep [1, 28]. A higher cutoff (RDI ≥ 15/h) is designated for asymptomatic individuals with none of the comorbid conditions listed. While the definition of obstructive apneas is straightforward (i.e., complete cessation of airflow for ≥10 s), published criteria for scoring obstructive hypopneas vary but generally require a ≥30 % reduction of airflow accompanied by either a ≥3–4 % O2 desaturation or an arousal [28]. RERAs reflect shifts in EEG waveform frequency to alpha, theta, or beta (except spindles) associated with <30 % reduction in airflow amplitude. Upper airway resistance syndrome (UARS) is a mild variant of OSA disorders characterized by the predominance of RERAs and the scarcity of obstructive apneas and hypopneas in a patient with daytime hypersomnolence. The diagnosis of UARS may require esophageal pressure monitoring, which can detect a series of progressively increasing negative inspiratory pressures leading to an arousal. [Note: The Center for Medicare and Medicaid Services, however, uses the AHI, which excludes RERAs, in determining whether OSAS therapies are reimbursed. The exclusion of RERAs may result in the underdiagnosis of OSAS, the underestimation of its severity, and, consequently, the undertreatment of its associated neurocognitive, cardiovascular, and metabolic dysfunction.] Meanwhile, home sleep testing (HST) using a type III portable monitoring device that records at least three respiratory parameters (i.e., airflow, respiratory effort, and oximetry) has been shown to have adequate diagnostic performance when administered in patients with a high pretest probability of moderate-to-severe OSA and with no significant comorbid cardiopulmonary, neurologic, or sleep disorders [29]. However, HST may miss milder forms of OSA including the UARS subtype, since RERAs cannot be detected without EEG monitoring. Peripheral arterial tonometry (PAT) analyzes the fluctuations in peripheral arterial blood flow, heart rate, and oxygen saturation associated with autonomic nervous system changes in response to sleep stage changes (REM vs. non-REM) and sleep-related breathing events. PAT technology has been validated in multiple studies using in-laboratory polysomnography as reference to have good diagnostic performance characteristics [30]. Overnight oximetry alone may be able detect the sawtooth desaturation pattern, or transient dips in SpO2 returning to baseline, characteristic of OSA. Although the O2 desaturation index (ODI, which is calculated as the number of times the oxygen saturation dips by 3–4 % divided by the number of hours of oximetry recording time) derived from an overnight oximetry correlates well with the severity of OSA, cases not associated with O2 desaturations (e.g., UARS) may go undiagnosed.
Therapy of OSA Disorders
CPAP is the treatment of choice for OSAS. CPAP functions as a mechanical splint which prevents airway collapse. To determine the optimal pressure level, the patient may either undergo an in-laboratory therapeutic PSG with CPAP titration or home auto-titrating CPAP therapeutic trial (Fig. 3.1). During the in-laboratory therapeutic CPAP titration, the technologist manually adjusts the pressure level to eliminate obstructive apneas, hypopneas, RERAs, and snoring detected via PSG (Fig. 3.2). Alternatively, the auto-titrating CPAP machine can detect apneas, airflow limitation, and snoring using a flow sensor and algorithmically adjust the pressure to eliminate them. Optimal CPAP levels obtained from manual vs. automatic CPAP titration are generally comparable. Meta-analytic studies seem to favor auto-CPAP over fixed CPAP in terms of adherence, sleep architectural changes, neurocognitive outcome (i.e., sleepiness), and patient preference [31, 32]. CPAP therapy has been associated with significant salutary effects on neurocognitive symptoms and cardiovascular complications [Table 3.2]. Despite its high efficacy, CPAP adherence rates are disappointingly low 30–60 % [33]. Barriers to CPAP adherence may include low confidence and motivation, cost, social stigma, lack of partner support, or adverse effects (e.g., poor mask fit, air leaks, facial skin irritation, claustrophobia, naso-oropharyngeal dryness, rhinitis, aerophagia, etc.).
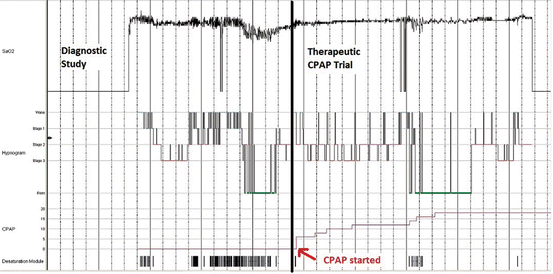
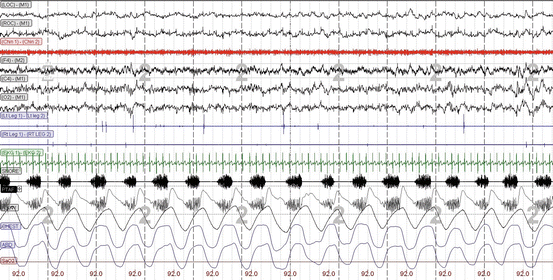
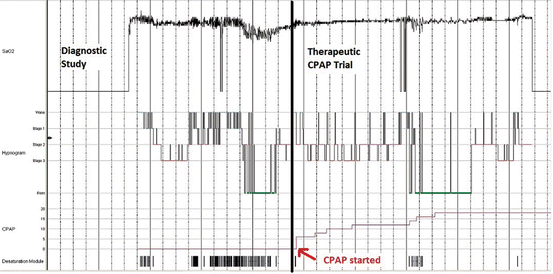
Fig. 3.1
Hypnogram of a split-night sleep study in a patient with obstructive sleep apnea. The diagnostic portion (period before the arrow) reveals recurrent nocturnal oxygen desaturations following a sawtooth pattern, with worsening during rapid eye movement (REM) sleep associated with frequent sleep–wake transitions. The therapeutic portion (period after the arrow) demonstrates resolution of nocturnal O2 desaturation with increasing continuous positive airway pressure (CPAP) levels, reduced sleep–wake transitions, and rebound of REM sleep
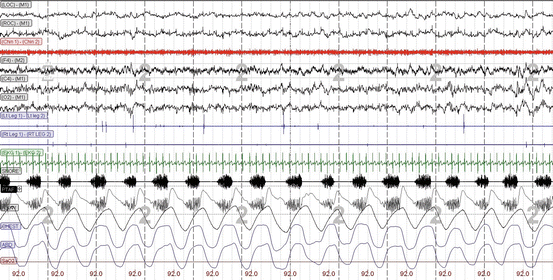
Fig. 3.2
A 30-s diagnostic polysomnogram epoch showing habitual snoring detected in the snore microphone (SNORE) as well as the nasal pressure transducer (PTAF) channels, in the absence of arousals or oxygen desaturation (SpO2). LOC left oculogram, ROC right oculogram, M1 left mastoid (reference electrode), Chin 1−Chin 2 chin electromyogram (EMG), F4 right frontal lobe EEG, C4 right central/vertex EEG, O 2 right occipital lobe EEG, LtLeg1−LtLeg2 left leg EMG, Rt Leg 1−RT LEG 2 right leg EMG, SNORE snore microphone, PTAF nasal pressure transducer airflow, FLOW nasal thermistor airflow, CHEST thoracic respiratory effort by respiratory inductive plethysmography (RIP), ABD abdominal respiratory effort by RIP, SaO 2 arterial oxygen saturation by pulse oximetry
Table 3.2
Salutary effects of continuous positive airway pressure (CPAP) therapy for obstructive sleep apnea
Organ system | Salutary effects of CPAP |
---|---|
Central nervous system | ↑ Neurocognitive function |
↑ Sleep quality | |
↓ Arousal index | |
↓ Stage 1 sleep | |
↑ Stages N3 (slow wave) sleep | |
↑ Stage rapid eye movement (REM) sleep | |
↑ Sleep efficiency | |
↓ Daytime sleepiness | |
↑ Driving simulator performance (↓ motor vehicle crashes) | |
↑ Vigilance | |
↑ Subjective work performance | |
↑ Self-reported health status | |
Cardiovascular system | ↓ Systemic hypertension |
↑ LV systolic and diastolic function | |
↑ LV end-diastolic diameter | |
↓ Pulmonary hypertension | |
Endocrine system | ↑ Luteinizing hormone and testosterone |
↓ ACTH and cortisol | |
↓ Atrial natriuretic peptide | |
↑ Aldosterone | |
↑ Insulin-like growth factor-1 | |
↑ Insulin sensitivity | |
↓ Prolactin level | |
Autonomic system | ↓ Norepinephrine |
Hematologic system | ↓ Hypercoagulability |
Immunologic system | ↓ C-reactive protein |
↓ Tumor necrosis factor-α | |
↓ IL-6 and IL-8 |
In OSA patients who refuse or cannot tolerate CPAP, the efficacy, benefits, and risks of alternative therapies [e.g., positional, oral appliance (OA), nasal expiratory positive pressure (EPAP), oral pressure (OP), and surgical therapies] should be discussed [34]. Although less efficacious in normalizing the AHI, positional therapy, or avoidance of the supine position with the use of a device (e.g., t-shirt with tennis balls inserted in a back pocket), may be tried for those with positional OSA [35]. Positional OSA is characterized by a 50 % reduction in AHI from supine to non-supine position and a non-supine AHI <10 events/h. Poor long-term positional device adherence may limit its efficacy. There are two types of OA devices: mandibular advancing devices (MADs) and the tongue-retaining devices (TRDs). MADs mechanically protrude the jaw, thereby pulling the tongue and soft palate anteriorly and increasing the pharyngeal size [36]. TRDs suction the tongue anteriorly to prevent retrolingual space collapse. OA therapy is more effective for those who have mild-to-moderate OSA, positional OSA, and lower BMI, particularly if 50–75 % mandibular protrusion is achieved [37]. Nasal EPAP devices adhere to the nostrils during sleep and function as one-way valves that allow minimal impedance to inspiratory flow but provide some resistance to expiratory flow. Although initial clinical trials demonstrated significant reductions in AHI particularly in mild-to-moderate OSA with lower BMI [38, 39], a more recent trial of nasal EPAP on patients withdrawn from CPAP therapy revealed recurrence of hypersomnolence and sleep-disordered breathing [40]. OP therapy is a novel device consisting of a suction hose and a mouthpiece that provides gentle suction in the oral cavity during sleep. The negative pressure in the oral cavity pulls the soft palate anteriorly toward the tongue, thereby opening up the retropalatal and retrolingual spaces. Early clinical trials on OP therapy for CPAP-intolerant patients show modest yet significant improvements in the AHI [41]. Surgical therapies include various otorhinolaryngologic procedures [42] as well as bariatric surgery [43, 44]. Although adenotonsillectomy is the treatment of choice for children with OSAS, otorhinolaryngologic procedures have not been as effective in treating OSA in adults. Uvulopalatopharyngoplasty (UPPP), the most commonly performed surgical procedure in adults with OSA, involves the removal of the uvula, tonsils, and part of the soft palate and reduces the severity of OSA by only 33 % [45]. Maxillomandibular advancement more effectively reduces the AHI by 87 % but requires a Le Fort I with bilateral sagittal split ramus osteotomies [45]. Tracheostomy can cure OSA by bypassing the upper airway obstruction but is rarely performed for this indication. Bariatric surgery reduces the severity of OSAS in majority of patients, cures the disease in a minority [44], and likely reduces the CPAP level requirements. Medications that are supposed to enhance ventilator drive (e.g., theophylline and progesterone), respiratory muscle function (e.g., growth hormone and anabolic steroids), pharyngeal muscle tone (e.g., serotonin reuptake inhibitors), and weight loss (e.g., phentermine and topiramate) are not reliably effective in ameliorating the respiratory and neurocognitive abnormalities in OSA [46]. On the other hand, wakefulness-promoting agents such as modafinil and armodafinil may be prescribed as adjuncts for those patients who have persistent subjective (e.g., based on the Epworth sleepiness scale) hypersomnolence despite adequate CPAP adherence (defined as average CPAP use of ≥4 h/night for ≥70 % of the nights in a 30-day period) [47, 48]. O2 therapy may improve nocturnal oxygenation but may also prolong the duration of respiratory events [49]. Sympathomimetic stimulants have cardiovascular side effects (e.g., tachycardia and hypertension) and are not approved for use in OSA. Exercise training [50] and playing wind instruments (e.g., didgeridoo [51]) achieved minimal to modest amelioration of OSA in unduplicated clinical trials. Further research on the efficacy of combining different modalities of non-CPAP therapies can determine whether this approach can improves outcomes in OSAS.
CSA Syndromes
Definition of CSA Syndrome
CSA syndromes are characterized by periodic episodes of absent or reduced airflow despite airway patency, interspersed between normal or crescendo–decrescendo breathing pattern. The ICSD-3 lists six subtypes of CSA syndrome in the adult: CSA with Cheyne–Stokes breathing, medication or substance-related CSA, CSA due to high-altitude periodic breathing, CSA due to medical disorder without Cheyne–Stokes, adult primary CSA, and treatment-emergent CSA [primary CSA syndromes of infancy and prematurity are discussed in pediatric SRBDs]. Although the older literature subclassifies CSA into nonhypercapnic vs. hypercapnic subtypes based on ventilatory chemoresponsiveness, the ICSD-3 classifies the hypercapnic subtype under “sleep-related hypoventilation disorders.”
Epidemiology of CSA Syndrome
The overall prevalence of CSA syndromes is not known but likely depends on the etiology or associated comorbidity. Because of its association with highly prevalent conditions (i.e., CHF, atrial fibrillation, and stroke), the most common subtype is CSA with Cheyne–Stokes breathing, which can occur in up to 40 % of patients with CHF [52]. Men are at a greater risk for CSA than women due to their increased ventilatory chemoresponsiveness [53]. Thirty percent of chronic (≥2 month) users of long-acting opioid (e.g., oxycodone, hydrocodone, methadone, etc.) can develop medication-related CSA [54]. The incidence of periodic breathing increases with the altitude: 25 % at 2,500 m (~8,000 ft) and 100 % at 4,000 m (~13,000 ft) [1]. CSA may also be associated with other medical disorders such as diabetes [55], hypothyroidism [56], and Chiari malformation [57]. Treatment-emergent CSA, or CSA unmasked during PAP therapy for OSA, can occur transiently due to the initial reduction of PCO2 below the apneic threshold, the reflex apnea associated with lung hyperinflation (Hering–Breuer reflex) due to excessive PAP, or the concomitant use of long-acting opioid medication and may persist in about half of patients with underlying increased ventilatory chemoresponsiveness [58]. Primary CSA in an adult is quite rare and should only be diagnosed when all other secondary causes have been ruled out.
Pathophysiology of CSA Syndrome
The basis for CSA with Cheyne–Stokes breathing is a heightened ventilatory response to carbon dioxide even after adjusting for body surface area, lung function, and oxygen consumption [59]. In patients with heightened ventilatory chemoresponsiveness, the expected slight increase in PCO2 during sleep triggers an exaggerated ventilatory response [Fig. 3.3]. This exaggerated response (hyperventilation) relative to the magnitude of the disturbance (sleep-related increment in PCO2) represents the increased loop gain in CSA syndromes [60]. The drop in PCO2 below the apneic threshold (PCO2 level below which apnea ensues) following the hyperventilation ushers in the central apnea–hypopnea phase of the periodic breathing pattern. The central apnea/hypopnea is then followed by a drop in O2 saturation and a rise in PCO2. The rising PCO2 and the subsequent arousal from the exaggerated ventilatory response trigger the release of catecholamines (i.e., norepinephrine) which are believed to further enhance ventilatory chemoresponsiveness, thereby perpetuating the cycle of periodic breathing. Meanwhile, comparison studies at high altitude of Himalayan Sherpas vs. Caucasian lowlanders found that the propensity for high-altitude CSA may be due to an increased peripheral hypoxic (rather than central hypercapnic) ventilator chemoresponsiveness [61]. The mechanism of chronic opioid-induced CSA is unclear, with studies on chronic methadone-induced CSA showing conflicting effects on ventilatory responses to O2 and CO2 [62].
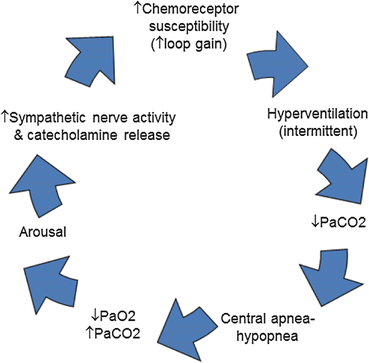
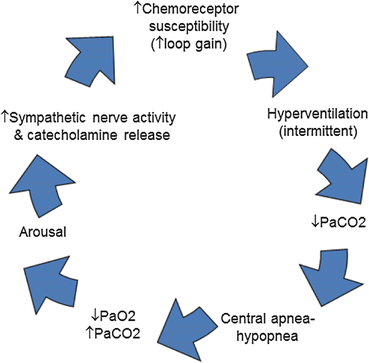
Fig. 3.3
Pathophysiology of central sleep apnea: enhanced ventilatory chemoresponsiveness (↑ loop gain) triggers intermittent hyperventilation in response to slight increases in PCO2 above a lower intrinsic apneic threshold. The drop in PCO2 below the apneic threshold results in central apnea or hypopnea which is then followed by a drop in SpO2 and a rise in PCO2. The rising PCO2 is associated with catecholamine release (i.e., norepinephrine), which further enhances ventilatory chemoresponsiveness, thereby perpetuating the cycle of periodic breathing [Modified from Köhnlein T, Welte T, Tan LB, et al. Central sleep apnea syndrome in patients with chronic heart disease: a critical review of the current literature. Thorax 2002; 57:547–554, with permission.]
Clinical Features of CSA Syndromes
CSA with Cheyne–Stokes breathing is characterized by cycles of waxing and waning tidal volumes in a crescendo–decrescendo pattern during sleep (and at times even during wakefulness), associated with fragmented sleep and frequent arousals. In contrast to OSA, in which arousals terminate the obstructive respiratory events, arousals from Cheyne–Stokes breathing usually occur at the peak of the crescendo phase of the cycle. The period from the start of one crescendo–decrescendo cycle to the start of the next cycle, or cycle length, correlates positively with the left ventricular function [63]. CSA due to high altitude may also present with a similar waxing and waning pattern, but the cycle length is generally shorter (20 s) [64], since most high-altitude climbers are presumed to have normal cardiac function. Other types of CSA are generally characterized by periodic breathing or alternating pauses and resumption of breathing without the crescendo–decrescendo pattern. The central apneas and associated arousals promote symptoms such as paroxysmal nocturnal dyspnea, insomnia, and daytime hypersomnolence. The clinical manifestations of CSA may also be based on its etiology. For example, patients with Cheyne–Stokes breathing pattern may present with signs and symptoms of CHF such as orthopnea, S3 or S4 gallop, bibasilar crackles, and or bipedal edema.
Diagnosis of CSA Syndromes
The diagnosis of CSA syndromes requires the presence of clinical features (i.e., hypersomnolence, insomnia, nocturnal awakenings from dyspnea, snoring, and witness apneas), polysomnographic predominance of central apneas or hypopneas (central apnea–hypopnea index ≥5/h), as well as the predisposing condition (e.g., CHF, atrial arrhythmia, and stroke; recent ascent to high altitude; intake of opioid medication; CPAP therapy for OSAS; other associated medical or neurologic conditions; etc.) (Fig. 3.4) [1, 28]. The diagnosis of CSA with Cheyne–Stokes breathing also requires the presence of crescendo–decrescendo breathing pattern. Primary CSA syndrome is diagnosed only after all other types and etiologies of CSA have been ruled out.
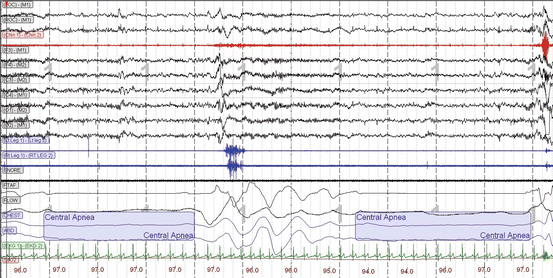
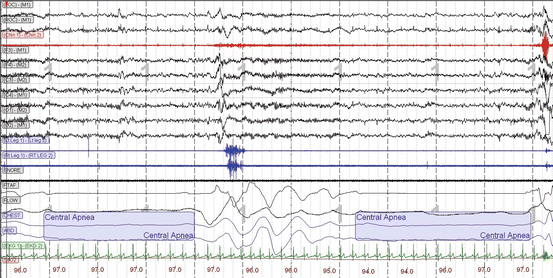
Fig. 3.4
A 30-s diagnostic polysomnogram epoch showing central apneas with absence of airflow in both the nasal pressure transducer (PTAF) and the nasal thermistor (FLOW) channels accompanied by the absence of thoracic (CHEST) and abdominal (ABD) respiratory efforts [LOC left oculogram, ROC right oculogram, M1 left mastoid (reference electrode), Chin 1−Chin 2 chin electromyogram (EMG), F4 right frontal lobe EEG, C4 right central/vertex EEG, O 2 right occipital lobe EEG, LtLeg1−LtLeg2 left leg EMG, Rt Leg 1−RT Leg 2 right leg EMG, SNORE snore microphone, PTAF nasal pressure transducer airflow, FLOW nasal thermistor airflow, CHEST thoracic respiratory effort by respiratory inductive plethysmography (RIP), ABD abdominal respiratory effort by RIP, SaO 2 arterial oxygen saturation by pulse oximetry]
Therapy of CSA Syndromes
Management of CSA syndromes should begin with the treatment of the underlying predisposing conditions. For example, decompensated CHF should be treated optimally (e.g., diuretics, vasodilators, beta-blockers, coronary revascularization, cardiac transplantation, etc.) in order to ameliorate Cheyne–Stokes breathing. Intensive pain management and detoxification may be required to reduce long-acting opioid use. Descent to low altitude and O2 therapy are the mainstay therapies for high-altitude periodic breathing, although acetazolamide may be used if immediate descent is not feasible.
Various modes of PAP therapy may be prescribed for CSA. CPAP is often initially tried, but finding the optimal fixed pressure level for an already patent airway, in the face of fluctuating waxing and waning tidal volumes, can be challenging. A randomized controlled trial found CPAP to be unsuccessful in reducing the central apnea index in up to 47 % of patients with CHF and Cheyne–Stokes breathing pattern [65]. Although CPAP is associated with improvements in surrogate cardiopulmonary measures (i.e., central apnea index, nocturnal oxygenation, left ventricular ejection fraction, norepinephrine levels, and 6-min walk distance), a post hoc analysis of the Canadian Positive Airway Pressure trial suggests that CPAP may prolong survival only in those whose central apnea index improves with this therapy [65, 66]. Adaptive support servo-ventilation (ASV), a bilevel mode that delivers an expiratory positive airway pressure (EPAP) to maintain airway patency and an automatically adjusting pressure support above the EPAP that adapts to changes in tidal volume, appears to be superior to CPAP in correcting CSA and Cheyne–Stokes breathing [67, 68], although long-term cardiovascular and mortality benefits have yet to be demonstrated. O2 therapy has been demonstrated to ameliorate CSA by blunting the hypoxic ventilatory drive, particularly in those with nocturnal hypoxemia [69]. Raising the PCO2 levels (via T-piece air plus CO2 sideflow, PAP gas modulator, or enhanced mask/tube dead space) can reduce central apneas [70] but may be associated with an increase in catecholamine levels [71]. Although theophylline [72], a nonspecific phosphodiesterase inhibitor that stimulates ventilation centrally, and acetazolamide [73], a carbonic anhydrase inhibitor that induces metabolic acidosis and triggers compensatory ventilation, can ameliorate CSA, their side effects often preclude their use in patients with CSA with Cheyne–Stokes breathing and comorbid cardiovascular disease.
SRHV Disorders
Definition of SRHV Disorders
SRHV disorders are characterized by sustained hypercapnia resulting from reduced rate of fresh gas transported to and from the alveoli during sleep. The ICSD-3 etiologically classifies them into four adult subtypes: obesity hypoventilation syndrome (OHS), SRHV due to medical disorder, SRVH due to medication or substance, and idiopathic central alveolar hypoventilation [1]. [Congenital central alveolar hypoventilation syndrome and late-onset central hypoventilation with hypothalamic dysfunction are discussed under pediatric SRBDs.]
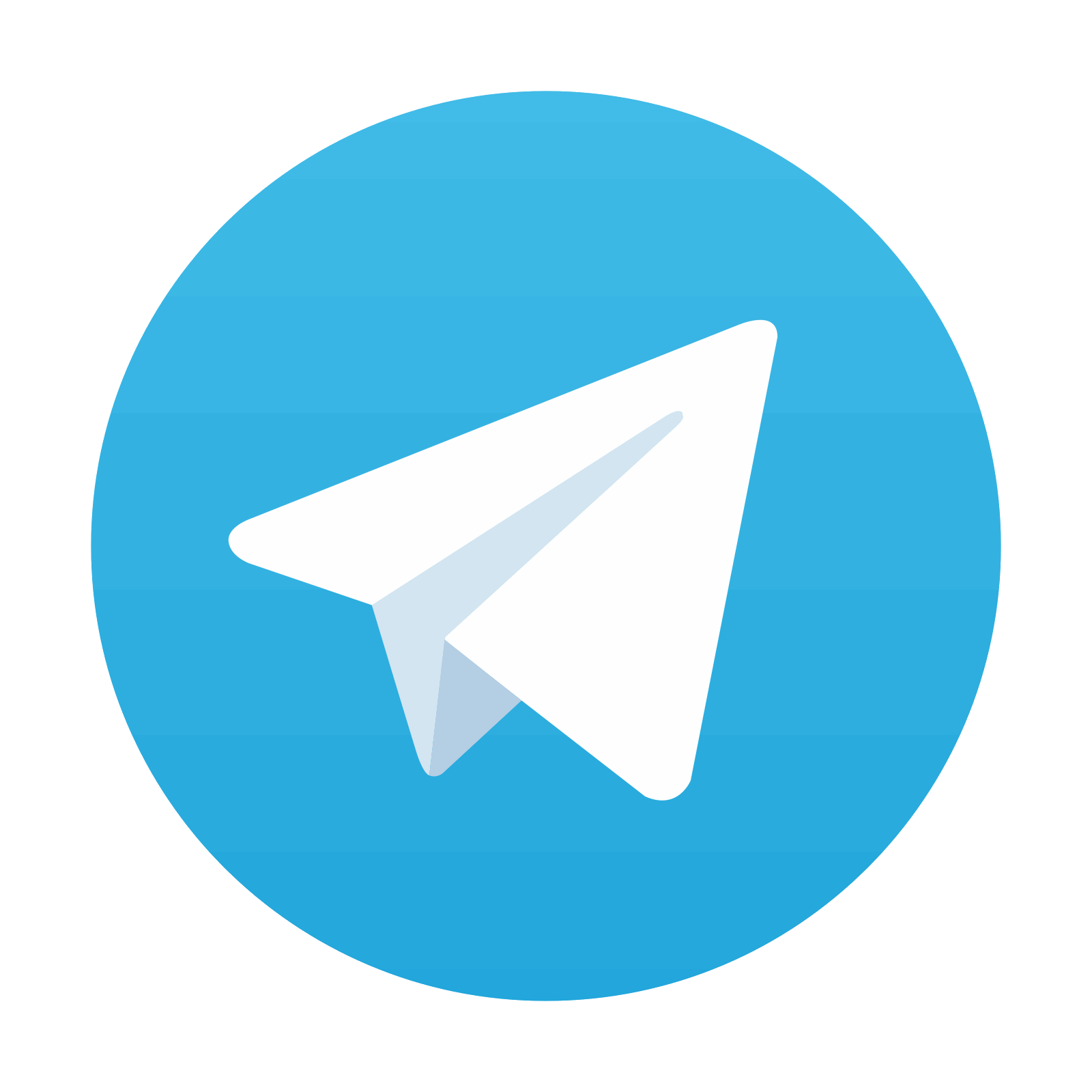
Stay updated, free articles. Join our Telegram channel

Full access? Get Clinical Tree
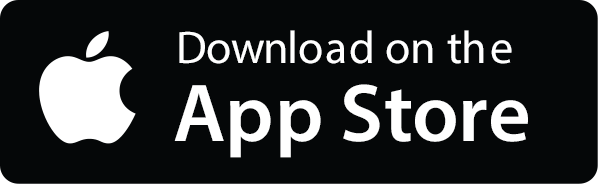
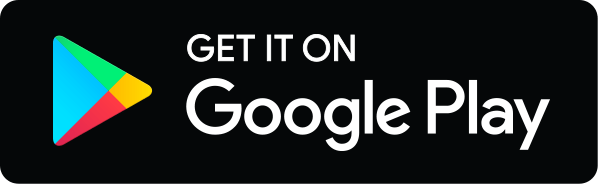