Sleepiness
Richard B. Berry
James D. Geyer
Paul R. Carney
Now blessings light on him that first invented this same sleep: it covers a man all over, thoughts and all, like a cloak; Tis meat for the hungry, drink for the thirsty, heat for the cold, and cold for the hot. Tis the current coin that purchases all the pleasures of the world cheap; and the balance that sets the king and the shepherd, the fool and the wise-man even. There is only one thing that I dislike in sleep; Tis that it resembles death; there’s very little difference between a man in his first sleep, and a man in his last sleep.
Miguel De Cervantes
Sleepiness is a prevalent and ill-defined symptom, experienced by almost everyone at some time. Sleepiness and fatigue have been used interchangeably in some publications, resulting in confusion and ambiguity. Sleep is to sleepiness as food is to hunger; sleepiness refers specifically to an increased likelihood of falling asleep. Fatigue refers to many different conditions, some of which do not include sleepiness; the term refers specifically to increased difficulty sustaining a high level of performance (1). Obviously, sleepiness is a normal human state and is desirable at times (e.g., bedtime). Just ask any insomniac!
Sleepiness is problematic when it disrupts daily living. Problem sleepiness is estimated to affect 0.5% to 25% of the population. Problem sleepiness has two primary causes: lifestyle factors, including sleep deprivation and medication effects, and sleep disorders, including sleep-disordered breathing, narcolepsy, and idiopathic hypersomnia.
This chapter reviews a historical perspective of sleepiness, the epidemiology of problem sleepiness, and determinants of sleepiness. A discussion of tools used to measure sleepiness, the consequences of sleepiness and sleep deprivation, and a brief overview of countermeasures are also included.
HISTORICAL PERSPECTIVE
In 1989, Allan Hobson wrote, “More has been learned about sleep in the past 60 years than in the preceding 6,000” (2). Although sleep and sleep disturbance have fascinated humans since the beginning of recorded time, it was not until the 19th century that the beginning of understanding about why we sleep and what happens if we do not began to emerge. In the 1920s, Nathanial Kleitman undertook sleep deprivation experiments and began to explore the consequences of sleepiness (3). Later that same decade, Hans Berger began recording brain electrical activity and noticed striking differences between the waking and sleeping electroencephalograms (EEGs) of humans (4). Understanding of the causes and consequences of sleepiness itself has recently assumed center stage in “Sleep Medicine,” fueled by rapid developments in the understanding of narcolepsy, improved tools to measure sleepiness, and the comprehension that sleepiness can be deadly in this era of rapidly moving vehicles.
EPIDEMIOLOGY OF PROBLEM SLEEPINESS
Several factors account for an increasing prevalence of sleepiness in the past century. Development of electricity (with reliable, inexpensive light), migration from the farm to the city (but away from the biologic tie to the rhythm of the sun), and the ability to travel across time zones have enabled us to be a 24-hour society. Up to one-third of this nation’s population works in shifts. Shift work has consistently been shown to result in poorer sleep quality and reduced daytime alertness (5). Increased intensity of hospital-based medical care and increased volume of air traffic are two examples of occupational sleep loss for physicians and air traffic controllers, respectively (6,7). Adolescents are increasingly required to report to school at inappropriately early hours, with reduction in nocturnal sleep time to <7 hours of sleep a night, at a time when their bodies need much more than that (8,9). In addition to these societal causes of increased sleepiness, the prevalence of obstructive sleep apnea (OSA), the most common sleep disorder associated with sleepiness is clearly increasing; reasons for this include the explosion
of obesity and the aging of the population (10). Taking these and other factors together, Bonnett and Arand (11) estimate that at least one-third of Americans are seriously, chronically sleep deprived and sleepy, and assert, “We must recognize the alertness function of sleep and the increasing consequences of sleepiness with the same vigor that we have come to recognize the society impact of alcohol.”
of obesity and the aging of the population (10). Taking these and other factors together, Bonnett and Arand (11) estimate that at least one-third of Americans are seriously, chronically sleep deprived and sleepy, and assert, “We must recognize the alertness function of sleep and the increasing consequences of sleepiness with the same vigor that we have come to recognize the society impact of alcohol.”
In contrast to calculations of the prevalence of sleep deprivation, surveys of humans have found the endorsement of sleepiness varies from 0.5% to 36% (12). Young adults (who often have young children and multiple jobs), the elderly, and shift workers are more likely to report sleepiness and to be sleepy by objective testing. In a population-based study in Finland, 11% of women and 7% of men reported sleepiness almost every day (13); very similar findings were reported from a large study in Sweden (14).
DETERMINANTS OF SLEEPINESS
Like most things in biology and nature, the variables affecting sleepiness and alertness are multiple and vary among individuals. Our understanding of the causes of sleepiness has lagged because of confusion about how to define and measure it. However, among the determinants of sleepiness are prior sleep, time of day, genetics, individual characteristics, medications, and disease, including both intrinsic sleep disorders and many medical conditions.
Prior Sleep
Both the amount of sleep that humans need and their ability to cope with inadequate amounts of it vary among individuals. Conventional wisdom urges 8 hours of sleep a night, but adolescents clearly need more than that (8,9). Kripke et al. reignited the debate about sleep need and its consequences with their American Cancer Society survey of more than a million individuals, demonstrating that lowest mortality rates were observed in those who slept 7 hours a night, and that those who reported sleeping ≥8 hours or ≤6 hours had increased mortality. It is likely that there are more subtle consequences of inadequate sleep time than mortality, however (see the section “Consequences”). Although there is tremendous variability in human sleep need, all of us get sleepier the longer we are awake and less sleepy the longer we sleep. Sleep is a biologic need like food or water and is necessary for survival. Homeostatic pressure is a term that has been applied to the increasing tendency to sleep with increasing time spent awake (15,16). In other words, the longer we stay awake, the sleepier we get. Although it is difficult to measure homeostatic pressure other than by measuring sleep latency (SL), characteristic EEG changes do occur with sleep deprivation: slow-wave sleep (SWS) increases, spindling decreases (17), and rapid eye movement (REM) sleep is likely to occur earlier. Homeostatic pressure (or “process S”) interacts with circadian rhythm (or “process C”) in the “two-process” model of sleep (18,19).
Time of Day
Sleepiness varies by time of day, in a predictable, modifiable circadian rhythm. Kleitman was probably the first to report that people who stayed up all night were less sleepy the following morning than they were in the middle of their sleepless night (3). The suprachiasmatic nucleus is the biologic clock that regulates hour-to-hour waking behavior. In forced desynchrony protocols, which theoretically eliminate or mask the homeostatic influence on sleep, subjective sleepiness and vigilance are lowest at about 6 AM, shortly after the nadir in core body temperature. There is also a midafternoon dip in core body temperature and alertness, which corresponds to the siesta time that many cultures routinely enjoy. A simplified way of understanding the two-process model is that homeostatic pressure (process S) makes us fall asleep, but circadian pressure (process C) helps us to stay asleep. SWS (deep nonrapid eye movement [NREM]) is thought to reflect homeostatic pressure, whereas REM sleep is regulated by circadian rhythm.
Genetics
Many sleep disorders, including narcolepsy, sleep apnea, and restless legs syndrome (RLS) have a genetic component. It is increasingly clear that sleep need has a genetic basis as well. The population-based Finnish twin study of >12,000 people yielded an estimate that about one-third of sleepiness is genetic (20). Using a rat model, Franken and colleagues have calculated that SWS need is under genetic control (21). Although the gene (or genes) that governs the heritable aspect of sleepiness and sleep need has not been discovered, it is likely that orexins (hypocretins) will be implicated as significant heritable regulators of sleep in the general population. There is a wide range of evidence indicating that the orexins are involved in the regulation of the sleep-wake cycle (22).
Medications and Neuropharmacology of Sleep
Almost every medication has an effect on sleep structure or daytime alertness, and evaluation of patients with sleep complaints or problems should include a critical investigation of medication history. A comprehensive review of effects of all drugs is beyond the scope of this chapter. Instead, we will focus on a review of the pharmacology of sleep and sleepiness and a discussion of the associated medications most pertinent to physicians.
Pharmacology of Sleep
At least three systems control and regulate sleep: circadian/homeostatic pressure, brain centers, and neurotransmitters. Neurotransmitters of sleep and wakefulness augment or mediate the propensity for sleeping and waking states. Table 8-1 lists some of the putative mediators of wake, NREM, and REM sleep (23).
Classic examples of effects of medications on wakefulness are that first-generation antihistamines promote sedation and drowsiness and catecholamine stimulants (e.g., isoproterenol) promote wakefulness. Examples of drug effects on NREM sleep include the fact that adenosine receptor blockers (e.g., theophylline, caffeine) promote wakefulness and gamma-aminobutyric acid receptor agonists (e.g., benzodiazepines) promote sleep; serotonin reuptake antagonists (SSRIs) can cause insomnia. Examples of pharmacologic effects on REM sleep are that nicotinic drugs (e.g., nicotine patches) promote REM sleep and dreaming. Anticholinergic drugs (e.g., tricyclic antidepressants) depress REM sleep. Most medications affect sleep structure or daytime alertness in some way.
Pulmonary Medications and Sleep
For individuals with hypoxemia due to chronic obstructive pulmonary disease (COPD) or sleep-disordered breathing, oxygen may improve sleep quality but does not improve daytime alertness. Theophylline is associated with increased sleep complaints in patients with COPD, asthma, and cystic fibrosis. Although there is evidence on both sides of the issue, studies claiming theophylline improves sleep frequently lack placebo groups and have high dropout rates (24). Corticosteroid use is associated with insomnia in asthmatics, in patients with optic neuritis, and in cancer patients. Polysomnographic (PSG) data on patients taking oral steroids demonstrate decreased REM sleep and increased wake after sleep onset (25). The effects of inhaled steroids on sleep have not been studied, but they are unlikely to disrupt sleep. Inhaled ipratropium bromide improves both sleep quality and Sao2 in patients with COPD (26).
TABLE 8-1 NEUROTRANSMITTERS OF SLEEP AND WAKEFULNESS | ||||||||||||||||||||||||||||
---|---|---|---|---|---|---|---|---|---|---|---|---|---|---|---|---|---|---|---|---|---|---|---|---|---|---|---|---|
|
Antihistamines
The effects of antihistamines on sleep and alertness are incompletely studied (27). The Food and Drug Administration classifies antihistamines as sedating or nonsedating. PSG data on the effects of antihistamines on sleep structure are very sparse. However, the available evidence suggests that disruption of sleep architecture and increased sedation is common with first-generation antihistamines due to their high lipophilicity (28). Sedating antihistamines shorten SL compared to placebo, cause measurably reduced alertness, may prolong total sleep time, impair driving, and affect performance on neuropsychological tests. Second-generation antihistamines cause less clinically significant sedative effects, but comprehensive studies of their effects on humans are lacking. Table 8-2 lists common sedating (first-generation) and nonsedating (second-generation) antihistamines.
With regard to administration of many medications, chronobiology is important. For example, in patients with asthma, oral corticosteroids are more effective if given at 3 PM, theophylline is more effective if given in the evening, and long-acting beta-agonists at bedtime reduce nocturnal awakenings.
Nicotine
Cardiac Medications
In general, antihypertensive agents may decrease the duration of REM sleep. Beta blockers, alpha agonists, and alpha antagonists can lead to sedation, which may be
transient and is dose related. Diuretics may cause sleep disruption secondary to nocturia. Compared with placebo, lipophilic beta blockers increase REM latency, reduce REM sleep, increase wakefulness, deplete endogenous melatonin stores, and may be associated with nightmares (31). 3-Hydroxy-3-methylglutaryl-coenzyme A reductase inhibitors (“statins”) are associated with insomnia. The Sleep Heart Health Study reported that the use of any medications for treatment of congestive heart failure was an independent risk factor for sleepiness (32).
transient and is dose related. Diuretics may cause sleep disruption secondary to nocturia. Compared with placebo, lipophilic beta blockers increase REM latency, reduce REM sleep, increase wakefulness, deplete endogenous melatonin stores, and may be associated with nightmares (31). 3-Hydroxy-3-methylglutaryl-coenzyme A reductase inhibitors (“statins”) are associated with insomnia. The Sleep Heart Health Study reported that the use of any medications for treatment of congestive heart failure was an independent risk factor for sleepiness (32).
TABLE 8-2 SEDATING AND NONSEDATING ANTIHISTAMINES | ||||||||||||||||
---|---|---|---|---|---|---|---|---|---|---|---|---|---|---|---|---|
|
Antidepressants
Most antidepressants suppress REM sleep and increase REM latency (exceptions to this are nefazodone and bupropion). In addition, antidepressants can exacerbate RLS. Rapid withdrawal may lead to nightmares and parasomnias. Both insomnia and daytime sedation are common side effects of the SSRIs.
Illness
Primary sleep disorders such as narcolepsy, idiopathic hypersomnia, and sleep apnea are associated with sleepiness. These and other primary disorders are extensively covered elsewhere in this volume. The relationship between “nonsleep” disorders and sleep is complex and bidirectional. Sleepy people perceive that their health is poorer than those who are not sleepy, and people who are sick are more likely to complain of sleepiness (33). Clearly, much of the sleepiness associated with acute and chronic illness results from medication (32) and from inadequate or low-quality sleep. Among those medical conditions that are infamous for being associated with daytime sleepiness are Parkinson’s disease (34), chronic renal failure (35), and dementia (36).
Illness likely mediates at least part of its effect on daytime sleepiness by affecting quality of sleep. Clearly, duration of sleep is important, but the number of arousals from sleep and the proportion of sleep spent in more restorative stages are often adversely affected by medical illness.
Environmental Influences
Given that sleepiness is the ability or tendency to fall asleep, environmental influences need to be taken into account. Activity, bright light, noise, temperature, posture, and stress can prevent or delay sleep onset in a person who is truly sleepy (37). With regard to behavior and posture, Johns has analyzed the components of the Epworth Sleepiness Scale (ESS) (see below) and concluded that lying down to rest in the afternoon and watching TV are most sleep promoting, whereas sitting and talking with someone and in a car stopped for traffic are least “somnific” (38). Much is known about the circadian rhythm in internal temperature and its relationship to sleep (in brief, sleepiness occurs as temperature falls), but the influence of environmental temperature on sleep is less well defined. Extremes of temperature clearly disrupt sleep, and REM sleep appears to be more vulnerable to temperature extremes than is NREM. Cold (21°C) is more disruptive than warm (34°C-37°C), and passive external warming (e.g., hot baths) has been shown to promote sleep in some studies (39). Historically, and for theoretical reasons, exercise just prior to sleep was discouraged; in actuality, late-night exercise has not been shown to impair sleep (40) and exercise, in general, is associated with improved sleep (41).
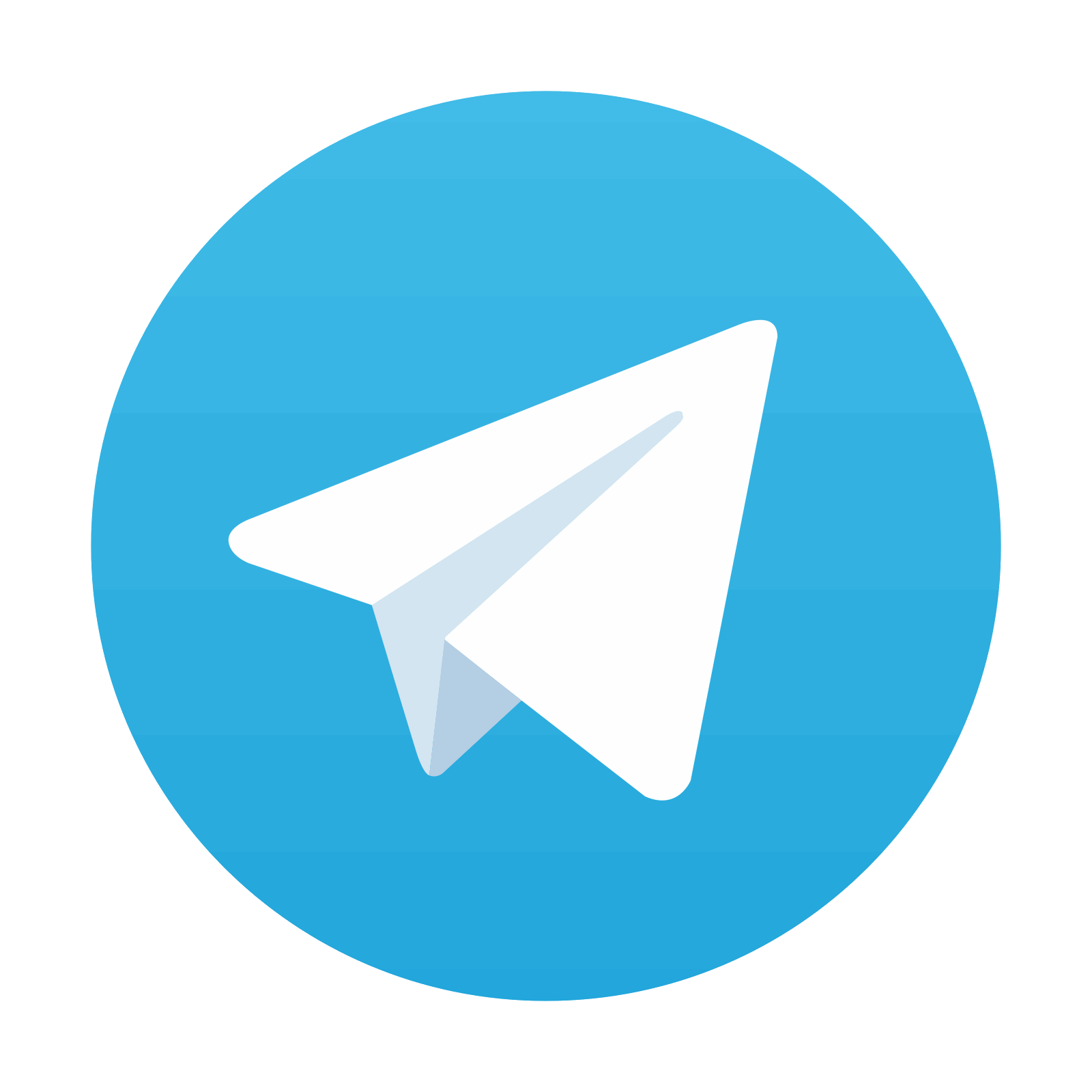
Stay updated, free articles. Join our Telegram channel

Full access? Get Clinical Tree
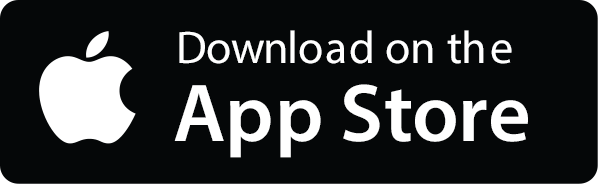
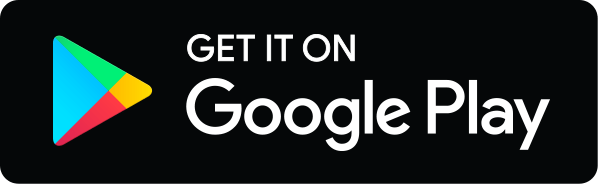