CLINICAL CASE | Neurosyphilis and Loss of Vibration Sense and Limb Proprioception
A 36-year-old man was admitted to the hospital for unsteadiness of gait and several other sensory and motor signs, including pain and limb strength impairments. Sensation to touch, pinprick, and temperature was normal. Perception of vibration and limb position sense were absent. When he was asked to stand upright with his eyes closed, he swayed and lost balance. This is a positive “Romberg sign.” His gait was broad based, clumsy, and staggering. MRI of his brain was normal but MRI of his spinal cord showed an intense signal in the dorsal columns, bilaterally (Figure 4–1A), that appeared to be the same as CSF.
The man had an untreated syphilitic infection for 10 or more years. He was diagnosed with neurosyphilis, also called tabes dorsalis, on the basis of several laboratory and neurological tests, including the MRI and sensory loss indicated above. This is the advanced stage of syphilis, when it infects the nervous system.
Try to answer the following questions based on your reading of the chapter, inspection of the images, and consideration of the neurological signs.
1. What functional system becomes impaired when there is neuronal degeneration in the dorsal columns?
2. Why does the patient demonstrate Romberg’s sign?
Key neurological signs and corresponding damaged brain structures NeurosyphilisSyphilis is normally treated with penicillin. Left untreated, the infectious agent—the spirochete, Treponema pallidum—infects the nervous system. In time, this can result in dysfunction or degeneration of its neuronal targets. Common target neurons are the dorsal root ganglion sensory neurons, which are important for mechanosensation. Especially vulnerable is limb position sense (or limb proprioception), which is signaled by muscle and joint sensory receptors, and vibration sense, which is signaled by Pacinian corpuscles. At autopsy, tabetic patients can show degeneration of the dorsal columns. This can be revealed by staining histological sections of the spinal cord for myelin. Oligodendrocytes also degenerate in regions where axons have degenerated, therefore showing demyelination (Figure 4–1B). The region of intense signal on the MRI demonstrates damaged dorsal column fibers.
Loss of limb proprioception and vibration senseBoth of these senses are mediated by dorsal root ganglion neurons that have a large-diameter axon, which project rostrally within the dorsal columns. In the absence of limb proprioceptive afferents, patients rely on vision to compensate for the loss of sensory awareness of their limbs. This explains the loss of balance when the patient closed his eyes. Touch is preserved in this patient; there may be diminished sensitivity, but this was not tested. Dorsal root ganglion mechanosensory neurons with a small-diameter axon may play more of a role in touch after degeneration of dorsal root ganglion neurons with large-diameter axons. This residual sensation is termed crude touch.
ReferenceStepper F, Schroth G, Sturzenegger M. Neurosyphilis mimicking Miller-Fisher syndrome: A case report and MRI findings. Neurology. Jul 1998;51(1):269-271.
FIGURE 4–1.
Degenerative changes in the dorsal columns with neurosyphilis. A. MRI (T2-weighted) at the level of the second thoracic vertebra. B. A section through the spinal cord of a person that had neurosyphilis while alive. This is a myelin-stained section. The white regions in the dorsal column correspond to demyelination because of axonal degeneration. A schematic drawing of the spinal cord is shown on top and highlights the degenerated region in the dorsal column (red) and the area of the histological image (box) in B. Note, the dorsal spinal cord surface is down in these illustrations. (A, Reproduced with permission from Stepper F, Schroth G, Sturzenegger M. Neurosyphilis mimicking Miller-Fisher syndrome: a case report and MRI findings. Neurology. 1998;51[1]: 269-271.)

The somatic sensory systems mediate our bodily sensations, including mechanical sensations, protective senses, as well as a wide range of visceral sensory experiences. Apart from our basic sensory capabilities—like discriminating textures and shapes of grasped objects or ensuring that we do not become hurt when we hold something too hot—somatic sensations are also critical for many integrative functions. Consider the capacity of touch to quiet the cry of a newborn baby or awaken us from a deep sleep. Somatic sensory information is critical for controlling movements, from the simplest reflexes—such as the stretch or withdrawal reflexes—to fine voluntary movements. Recall how awkward speech and facial muscle control become when sensation of our jaw and lips is blocked by local anesthetic injection in preparation for dental work. Somatic sensations are clinically important: pain typically brings a person to the doctor; touch, vibratory sense, a mechanosensory component, and pin prick are routinely used to probe sensory function in humans suspected of having peripheral nerve or central nervous system damage.
Spinal somatic sensory systems receive information from the limbs, neck, and trunk, while the trigeminal systems receive information from the head. The spinal and trigeminal systems remain distinct as they travel to the cerebral cortex, contacting separate populations of neurons at each processing stage. However, even though the ascending spinal and trigeminal pathways are anatomically distinct, their general organization is remarkably similar.
This and the next two chapters focus on the somatic sensory systems. The first two chapters consider the spinal systems mediating mechanosensation and the protective senses, because they have distinctive anatomical organizations. The third chapter examines the trigeminal and viscerosensory systems. We consider these two somatic sensory functions together because they are both mediated in large part by particular cranial nerves and their key central nervous system processing centers are closely aligned.
In this chapter, somatic sensations and the overall functional organization of the spinal sensory systems are discussed first. Then the regional anatomy of the mechanosensory system is examined at different levels through the nervous system, beginning with the morphology of the somatic sensory receptor neurons and continuing to the cerebral cortex.
The somatic senses consist of many distinct components that can be further subdivided, termed modalities and submodalities. This diversity adds to the richness of somatic sensations (Table 4–1). A somatic sensory submodality is thought to be mediated by a single type of sensory receptor.
Modality and submodality | Receptor type | Fiber diameter (μm) | Group | Myelination |
---|---|---|---|---|
Touch | ||||
Texture/superficial | Meissner’s and Merkel’s receptors | 6-12 | A-β (2) | Myelinated |
Deep pressure | Mechanoreceptors | |||
Vibration | Pacinian | |||
Sensual touch | Mechanoreceptors | 0.2-1.5 | C | Unmyelinated |
Limb proprioception | ||||
Static/dynamic (kinesthesia) | Muscle stretch and tendon force (primary and secondary; Golgi tendon organs) | 13-20; 6-12 | A-α (1), A-β (2) | Myelinated |
Thermal Sense | ||||
Cold | Cold receptors | 1-5 | A-δ (3), C (4) | Myelinated; unmyelinated |
Warmth | Warmth receptors | 0.2-1.5 | ||
Pain | ||||
Sharp (pricking; fast) | Nociceptors | 1-5 | A-δ (3), C (4) | Myelinated; unmyelinated Myelinated; unmyelinated |
Dull (burning; slow) | 0.2-1.5 | |||
Itch | Pruritic receptor | 0.2-1.5 | C (4) | Unmyelinated |
Visceral sensation | ||||
Blood pressure, chemosensory; ion sensing, etc. | Mechano, thermo, chemoreceptors | Various; not well understood | Various; not well understood | Myelinated; unmyelinated |
Touch comprises distinct superficial and deep submodalities that allow us to sense smooth and rough textures, the shapes of objects, and the pressure exerted by objects pressed onto the skin over muscle (deep pressure). Vibration sensitivity is used routinely for sensory testing. People with impairments in these modalities experience clumsiness and incoordination. Sensual touch is a soothing, but poorly localized, form of touch. It is important more in affecting our emotions than discrimination. These various submodalities are mediated by different types of mechanoreceptors.
Proprioception is our sense of limb position and limb movement (kinesthesia). Whereas vision supplements proprioception, healthy individuals are also keenly aware of where their limbs are relative to the body axis, gravity, and to each other. Together with touch, proprioception is critical for movement control.
Thermal senses, separate warmth and cold, provide critical information about the safety and comfort of our environment, as well as enable us to maintain our body temperature within narrow limits.
Pain alerts the individual of tissue damage, present or impending. Pain is mediated by specific nociceptors. It comprises sharp pricking pain and a dull burning pain.
Itch is triggered selectively by chemical irritation of the skin, especially in response to particular tissue inflammatory agents. Itch provokes the urge to scratch, thereby tending to remove the offending substance.
Visceral sensation provides not only awareness of the internal state of our body but also the information for regulating many bodily functions, such as blood pressure and breathing. Many aspects of visceral sensation are never conscious, such as arterial pressure, and others are only so under special circumstances, such as nausea and fullness.
Many of these modalities and submodalities are engaged during routine activity. For example, in picking up a cup of coffee, you use proprioception in identifying the location of your hand as you reach to grasp the handle; contact with the cup is detected by touch. If the cup is warm, your temperature sense is recruited, and if it is hot, you experience pain. After consuming caffeine in the coffee, your heart may beat faster, which is sensed by both visceral sensory receptors and mechanoreceptors in the chest.
Touch and limb position sense are mediated by the dorsal column–medial lemniscal system (Figure 4–2), named after its two principal components. After a lesion of the dorsal column–medial lemniscal system, the person’s tactile thresholds become elevated and discriminative capabilities are markedly reduced. An individual with such a lesion, for example, may not be able to distinguish gradations of rough and smooth (eg, grades of sandpaper). Moreover, this individual also would have difficulty maintaining balance with his or her eyes closed because of the absence of leg position sense. This set of impairments occurs in tabes dorsalis, an advanced stage of neurosyphilis, because dorsal root ganglion neurons with large-diameter axons degenerate. Fortunately, tabes dorsalis is rare today because of antibiotics. A crude sense of touch remains after a dorsal column lesion, indicating that other spinal pathways receive input from mechanoreceptor neurons but that this information is not organized for fine discriminations. This is discussed briefly in Chapter 5 with pain and temperature senses.
FIGURE 4–2
Organization of the dorsal column–medial lemniscal system. A. Dorsal view of the brain stem without the cerebellum, illustrating the course of the dorsal column–medial lemniscal system. A2 shows the dorsal root ganglion neuron and the organization of the primary afferent fiber. The sensory receptor illustrated in A2 is a mechanoreceptor, a Pacinian corpuscle. The inset shows views of lateral and medial surfaces of the cerebral cortex. B. The dorsal column-medial lemniscal pathway, as viewed by a series of transverse slices through the brain stem and a coronal slice through the thalamus and cerebral cortex.


A three neuron circuit transmits sensory information from the periphery to the cerebral cortex. Figure 4–2A overlays the circuit on the views of the spinal cord, dorsal brain stem, and thalamus; Figure 4–2B presents the circuit in relation to a sequence of slices through the spinal cord and the brain. Mechanoreceptors, a specialized kind of dorsal root ganglion neuron, provide the major sensory input to the dorsal column–medial lemniscal system. The central branches of mechanoreceptors synapse both in the spinal cord, which is primarily important for bringing information to spinal motor circuits for reflex function, and in the medulla. The synapse in the medulla is located in the first major relay in the dorsal column–medial lemniscal system, in the dorsal column nuclei. Here, the first-order neurons in the pathway, the primary mechanosensory neurons, synapse on the second-order neurons in the central nervous system (Figure 4–2). The axons of the second-order neurons decussate in the medulla. These axons travel in the medial lemniscus, which transmits information primarily to the ventral posterior lateral nucleus of the thalamus. Thalamic neurons in this nucleus project their axons to the primary somatic sensory cortex in the postcentral gyrus (Figure 4–2). This cortical area is important in localization of mechanical stimuli and in identifying the quality of such stimuli.
From the primary somatic sensory cortex, information is transmitted to higher-order cortical areas located ventrally and dorsally that play a role in more complex aspects of touch and position sense. Cortical areas that are located ventrally, including the secondary somatic sensory cortex (see Figure 4–2, inset), are important for recognizing objects by touch and grasp alone, or “what” something is. Dorsal areas, including area 5 (see Figure 4–12), are important in spatial localization, or “where” something is located. The dorsal areas are also important in using mechanosensory information for guiding hand and arm movements. We will learn that the visual and auditory cortical areas also have dorsal, or “where,” and ventral, “what,” areas.
The rest of this chapter takes a regional approach to the spinal mechanosensory system. Progressing in sequence from the periphery to the cerebral cortex, the chapter examines the key components of the dorsal column–medial lemniscal system. Knowledge of the regional anatomy is important for understanding how injury to a discrete portion of the central nervous system affects different functional systems.
The dorsal root ganglion neurons, named for the dorsal root ganglia in which their cell bodies are located, are pseudounipolar neurons (Figure 4–2A2). A single axon emerges from the cell body and bifurcates; one axonal branch is directed toward the periphery, where it innervates the skin or other tissues, and the other, directed centrally, synapses on central nervous system neurons. The peripheral and central axon branches of dorsal root ganglion neurons are often called primary sensory (or afferent) fibers.
The peripheral axon terminal is the receptive portion of the neuron. Here, stimulus energy is transduced into neural signals by membrane receptor-channel complexes that respond to a particular stimulus energy (eg, mecanical or thermal). Mechanoreceptors are activated when mechanical energy is conducted from the body surface, where stimulation occurs, to the membrane of the receptors, where stretch-activated channels are located. Mechanoreceptors for limb position sense are sensitive to muscle or tendon stretch as well as mechanical changes in the tissues around muscles and joints.
Mechanoreceptors have encapsulated axon terminals. Five major types of encapsulated sensory receptor neurons are located in the skin and underlying deep tissue that mediate mechanosensations: Ruffini’s corpuscles, Merkel’s receptors, Meissner’s corpuscles, Pacinian corpuscles, and hair receptors (Figure 4–3A). Merkel’s receptors and Meissner’s corpuscles are located at the epidermis-dermis border. These receptors are sensitive to stimulation within a very small region of overlying skin; hence they have very small receptive fields. These receptors are important for fine tactile discrimination, such as reading Braille. Ruffini’s and Pacinian corpuscles are located in the dermis. Ruffini’s corpuscles are sensitive to skin stretch and are important in discriminating the shape of grasped objects. Pacinian corpuscles are the most sensitive mechanoreceptor, responding to skin displacement of as little as 500 nM. Merkel’s receptors and Pacinian corpuscles are rapidly adapting, responding to changes in the stimulus, such as when it comes on or shuts off. Meissner’s corpuscles and Ruffini’s receptors are slowly adapting, firing action potentials for the duration of the stimulus. Hair receptors may be either slowly or rapidly adapting. Each primary sensory fiber has multiple terminal branches and, therefore, multiple receptive endings.
FIGURE 4–3
A. The morphology of peripheral somatic sensory receptors on hairy skin (left) and hairless, or glabrous, skin (right). B. The muscle spindle organ (top inset) is a stretch receptor located within the muscle. It receives an efferent innervation from the spinal cord that maintains receptor sensitivity during muscle contraction. Specialized motor neurons, termed gamma motor neurons, innervate muscle fibers (intrafusal fibers) within the receptor’s capsule. The synapse between the gamma motor neuron and the intrafusal fiber is termed the gamma motor ending. C. The Golgi tendon organ, located within tendons, is most sensitive to active force generated by contracting muscle. (A, Adapted from Light AR, Perl ER. Peripheral sensory systems. In: Dyck P, Thomas PK, Lambert EH, Bruge R, eds. Peripheral Neuropathy, 3rd ed. Vol 1. Philadelphia, PA: W. B. Saunders; 1993. B, Adapted from Schmidt RF. Fundamentals of Neurophysiology, 3rd ed. Berlin, Heidelberg, New York: Springer; 1985.)

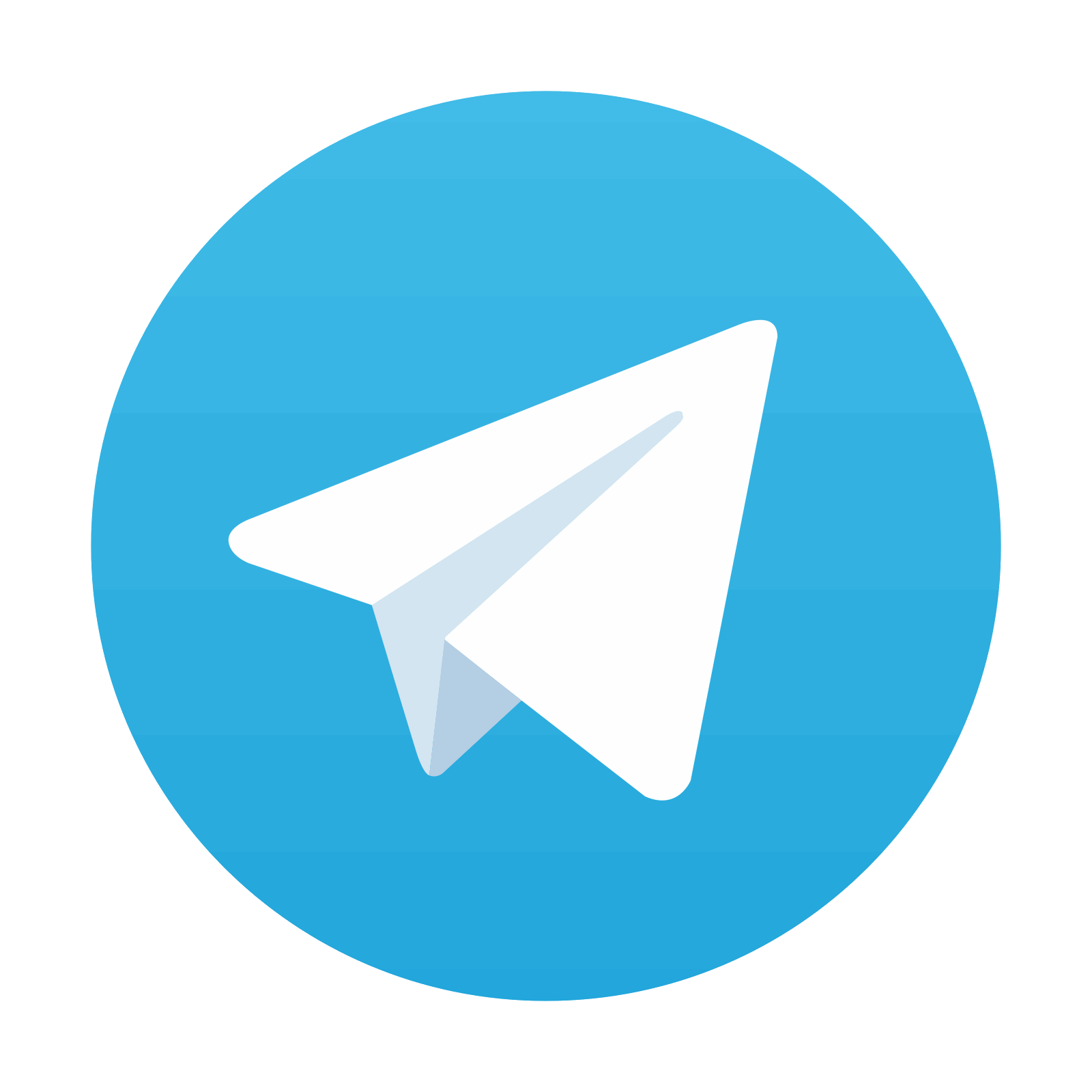
Stay updated, free articles. Join our Telegram channel

Full access? Get Clinical Tree
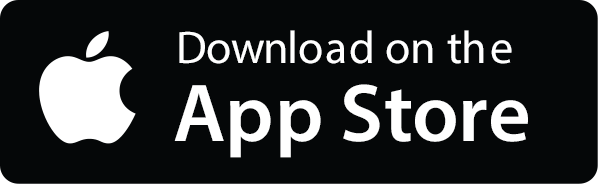
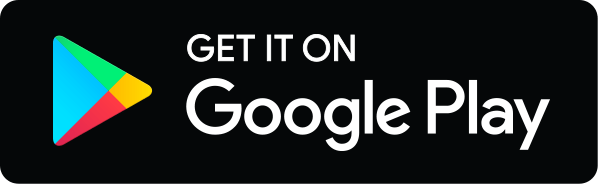
