An essential step in EEG interpretation is the identification of features that are statistically deviant compared to normative data, a process that defines abnormalities and establishes their significance in appropriate clinical context. EEG patterns evolve with maturation; neonates reveal distinct features that regress within 4–6 weeks after birth to be replaced by patterns characteristic of infancy and early childhood. The patterns also vary with the child’s state at time of recording, the level of alertness during wakefulness, and cycling through stages of sleep. Recognizing the considerable variability of normative EEG features is thus a prerequisite to interpretation.
Once an abnormality is identified, the next goal of interpretation is to define its location and extent. While localization of abnormality is useful in most clinical settings, it gains particular relevance for patients with intractable epilepsy undergoing evaluation for resective surgery. Guidelines for localization were initially described by Adrian and Matthews1 and have been subsequently addressed in several comprehensive reviews.2,3,4,5 Principles of electric field theory were addressed by Nunez,6 the physiological substrates of EEG sources became better appreciated after the introduction of intracranial recordings.7,8 The pitfalls and caveats underlying many of the physical and physiological assumptions used in localization of epileptic sources were reviewed by Jayakar et al.9
The growing success of resective surgery over the past few decades increased the need for localizing accuracy. This led to the development of automated computational systems for 3-D source localization of spikes recorded on EEG. More recently, magnetoencephalography (MEG) and a hybrid technique where EEG spikes trigger activation of functional MRI signal have also been utilized. While these new methods are gaining popularity in many epilepsy surgery centers, the set of underlying assumptions are not generally well understood. The main focus of this review article is to discuss the principles of localizing seizure foci; the intent is not to reiterate the standard teachings of localization but rather to emphasize the limitations and pitfalls underlying many of the assumptions used in visual or automated analyses and the potential for misinterpretation.
Localization is facilitated by modeling the spike generator, the choice of a model is dictated by the distribution of the excitatory (negative) and inhibitory (positive) postsynaptic potentials within the generator. Since the majority of cortical neurons are arranged in parallel and activate synchronously by virtue of their interconnections, the respective potentials summate to generally produce layer of negativity on the superficial aspect of the cortex, and positivity on its deeper aspect, that is, a dipole layer.
The orientation of a generator greatly affects its field distribution on the scalp.4 If a generator is located over a superficial gyrus, the dipole is oriented vertically to the scalp, that is, it is a “vertical dipole” and is the commonest orientation observed on the scalp EEG. Electrodes overlying the vertically oriented generator record negative spikes with a bell-shaped negative potential distribution field. The generator is interpreted to be located under the peak, that is, the electrode revealing spikes of maximum negativity. A bell distribution with restricted field implies a superficial generator; broad fields imply that the generator extends over a wider cortical region or is located deep.
Less frequently, the generator may be located in the bank of a sulcus or in the interhemispheric fissure and is oriented tangential to the scalp, that is, it is a “horizontal dipole.” Its potential distribution curve is “S” shaped with a peak (negative) and a trough (positive). Unlike the scenario of a vertical dipole, the generator is not located under the peak or trough but lies in between; the wider apart are the peak and trough, and the deeper is its likely location. In children with benign rolandic epilepsy, the larger negative peak is distributed over the central/parietal region and the smaller trough over the frontal region consistent with an epileptic source in the rolandic sulcus.
Scenarios of surgical relevance include patients with interhemispheric foci such as those involving supplementary areas of the cortex. As a result of horizontal dipolar distribution, maximum spike negativity may project contralateral to the side of the focus. When the source is deep, the peak or trough may project at remote electrodes sites. For example, spikes from an anteromesial temporal focus may appear most prominent in frontopolar or supraorbital electrodes.10 Likewise basal temporal foci may project a positive trough at the vertex (Fig. 4–1). Recognizing these pitfalls allays consternation and may avert the need for extensive implantation of intracranial electrodes.
Figure 4–1.
Scalp EEG recording using an average montage derivation showing spikes with negativity over the right temporal region (T4/F8) and a concomitant positivity over the vertex, a horizontal dipolar distribution seen in mesial temporal foci. The spikes also propagate and appear to be synchronous over the left temporal region.

In epileptic substrates of cortical malformations, the pyramidal neurons have a disorganized arrangement. Without the parallel alignment, the field potentials from neighboring neuronal groups may cancel each other instead of summating into an ordered dipole layer. Such spike foci behave as “closed” fields11 to be undetectable on scalp recordings or present as several scattered “microfoci” within a region. Computation of these sources may require higher order or multiple dipole models.6,12
The magnitude of the field potential recorded on the scalp varies inversely to the square of the distance of the recording electrode from the generator. The drop-off is not uniform in all directions but depends on the inhomogeneity, that is, varying conductivities and shapes of the media (cerebrospinal fluid [CSF], skull, scalp) across which the current traverses. Whenever currents approach media with differing conductivity, they “bend” toward the path of least resistance. The CSF, skull, and the diploic space between the skull tables act to shunt current away from the scalp electrodes and further attenuate activity recorded on the scalp. Scalp amplitudes are generally 8- to 20-fold lower, the attenuation being greater for generators that involve only a small cortical surface.6,13 As an estimate, spike discharges may not be seen on the scalp unless one square inch or more of cortex is synchronously involved.13 The fast activity at ictal onset is even less likely to synchronize over adequately large cortical areas and thus often goes undetected on scalp recordings. This is potentially misleading since sites to which the seizure propagates may reveal higher amplitude slower seizure sequence; the focal “attenuation” of background at seizure onset may be the only clue to the focus.
Currents from the generator also preferentially flow toward skull defects and foramina because of their lower resistance. Mesial temporal foci may appear prominent over frontopolar regions or the ear reference may be significantly contaminated by distant generators. Even sphenoidal electrodes are not specific for mesial temporal foci and have been shown to reveal prominent spikes in patients with orbitofrontal foci.10
Interictal spikes are known to propagate from the primary sites of origin and reveal a wide field of distribution. Propagated discharges cannot be localized by defining an apparent peak/trough of a single-dipole source but must through identifying a lead from the focus. The time difference between the source and propagated sites may be subtle and not readily evident to visual examination (Fig. 4–1). Identification of subtle leads is facilitated by using faster speed screen displays, via use of a reference subtraction derivation14 or computational techniques.15
Additional cues may come from studying the spike morphology. The prominent negative peak with a widespread field is often preceded by a small positive trough with a more localized distribution; this initial component albeit small is useful to identify the focus. Similarly, the focus is more likely to reveal multiphasic spike morphology compared to broader sharp waves at propagated sites.7
For computational 3-D source analyses of propagated discharges, more complex models such as moving dipoles, spatiotemporal dipole, or distributed current density models are better suited than a single-dipole model.
Propagated spike or seizure discharges have been shown to “skip” regions and appear as well-circumscribed “pseudofoci” at considerable distances from the primary focus.16,17,18 Such propagations can occur along all known major fiber pathways and may even occur across regions without known anatomic connectivity. In situations where a primary focus located in basal or mesial regions propagates to the lateral convexity, the secondarily activated “pseudofocus” may easily be mistaken for the primary generator. Differentiating the primary focus from pseudofoci is not always possible.
The presence of background abnormalities, especially the lack of fast activity in the same region strongly suggests that the observed focus is the primary one. Likewise, the focal attenuation at or near ictal onset generally does not “skip” regions and unlike spike or beta discharges is a useful indicator of the primary focus.
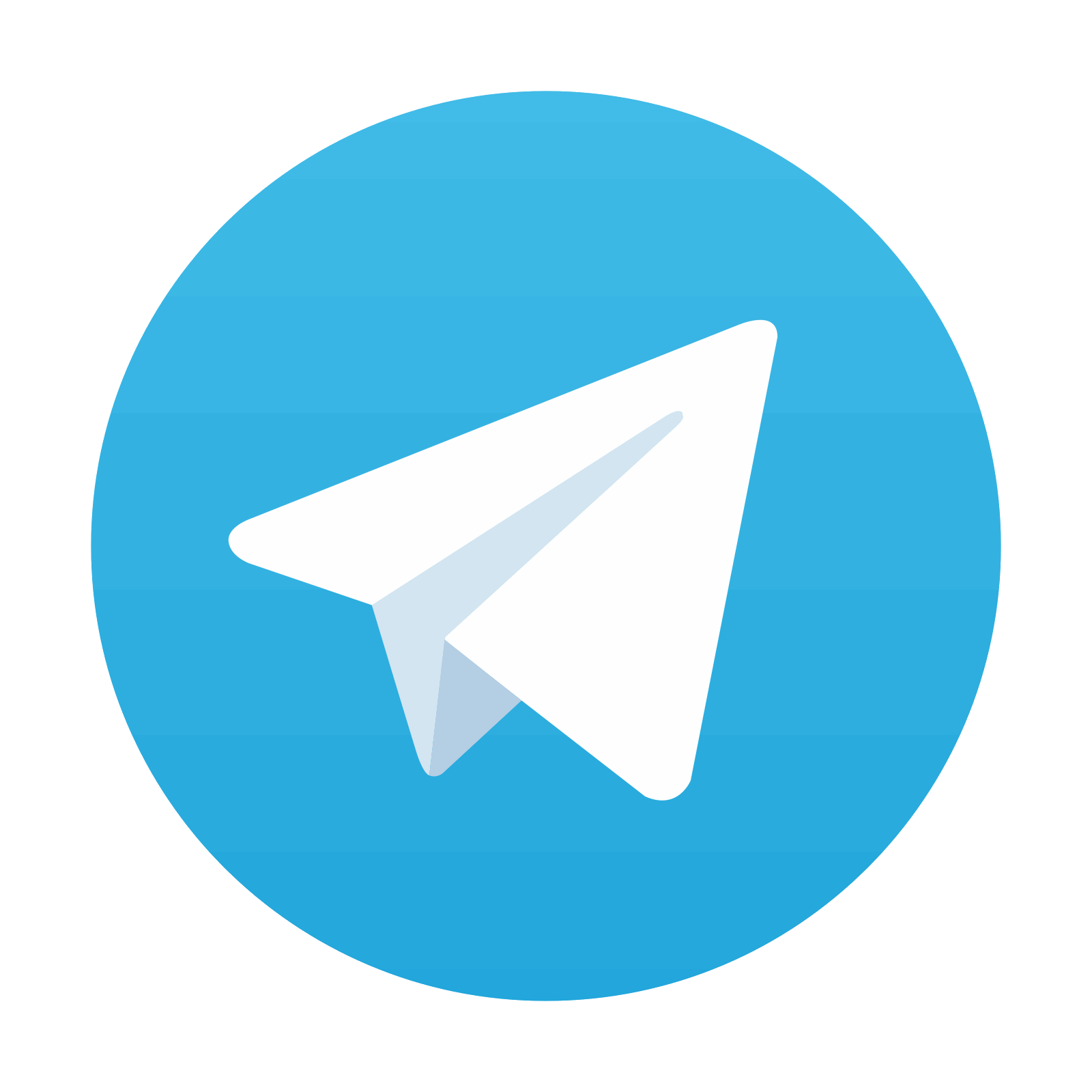
Stay updated, free articles. Join our Telegram channel

Full access? Get Clinical Tree
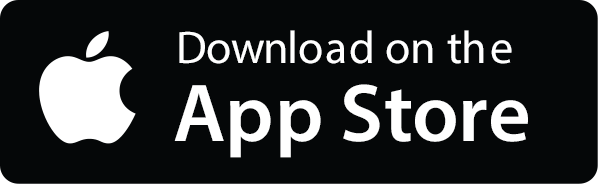
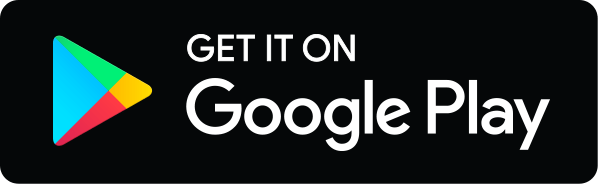