List of abbreviations
AD
autonomic dysreflexia
AIS
American Spinal Injury Association Impairment Scale
FDA
Food and Drug Administration
IDE
Investigational Device Exemption
L
lumbar (referring to spinal cord level)
scES
spinal cord epidural stimulation
SCI
spinal cord injury
T
thoracic (referring to spinal cord level)
S
sacral (referring to spinal cord level)
Introduction
Spinal cord injury (SCI) affects nearly 2 million people in the United States ( ) and results in profound neurologic impairment with widespread deficits to sensorimotor and autonomic systems. While the lack of ambulation is most widely recognized, there are many internal systems that are disrupted after SCI due to the critical role of the spinal cord in coordinating bodily functions. As a result, individuals with SCI endure a myriad of complications that result in increased mortality, morbidity, hospitalization, high burden of care and health care costs, and a drastically lowered quality of life ( ).
The autonomic nervous system serves to provide essential regulatory communication between the brain and internal organs, vascular supply, and numerous glands in order to maintain dynamic equilibrium and homeostasis of all systems. Sympathetic and parasympathetic systems (including the enteric system for the gastrointestinal tract) function to integrate complex reflex pathways between the target organ and integration centers within the nervous system ( ). Thus, autonomic disruption extends across multiple systems and drastically impacts overall health and quality of life. Alterations of urogenital and bowel dysfunctions are consistently rated as top consumer priorities for recovery post-SCI ( ; ; ). Acutely post-SCI, bladder dysfunction often manifests as “flaccid paralysis,” resulting in urinary retention necessitating bladder catheterization. Overtime, bladder dysfunction progresses into detrusor hyperreflexia and detrusor-sphincter dyssynergia, resulting in incontinence and incomplete emptying. Gastrointestinal disruptions after SCI are highly prevalent and include a wide-range of symptoms, including delayed gastric emptying, diminished propulsive transit, abdominal distention, pain, constipation, anal fissures, hemorrhoids, rectal prolapse, and sphincter/defecation disturbances ( ). Cardiovascular dysfunction associated with autonomic dysregulation contributes to increased morbidity and is the leading cause of mortality in acute and chronic SCI ( ). The disruption of supraspinal control over sympathetic pathways results in decreased sympathetic tone below the level of injury and impaired autonomic function and blood pressure regulation ( ). One critical complication that occurs in individuals with lesions above T6 is autonomic dysreflexia (AD), an exaggerated sympathetic nervous system response to a noxious stimulus below the level of injury, including bladder/bowel distention, urinary tract infections, fecal impaction, and orgasms ( ). As a result, increased cardiovascular dysregulation has a profound effect on the body as a whole and significantly interferes with motor and autonomic recovery post-injury.
Bladder dysfunction after SCI
Long-term deficits in bladder function after SCI manifest as detrusor hyperreflexia (bladder contractions at low volumes, causing incontinence and smooth muscle hypertrophy), detrusor-sphincter dyssynergia (uncoordinated bladder and external urethral sphincter contractions, causing inefficient emptying and smooth muscle hypertrophy), decreased compliance (unable to store urine under appropriately low pressures) and loss of continence ( Fig. 1 ) requiring lifelong management, maintenance, and health care visits ( ). Major urological concerns contributing to increased morbidity and mortality include repeated lower urinary tract infections that can lead to sepsis, chronic vesico-ureteral reflux and hydronephrosis with progression to renal failure as a result of high-intravesical pressures, and inter-related cardiovascular complications such as AD ( ) that limits bladder storage ( ). Current therapies to improve the efficiency of bladder voiding, management and continence after SCI include catheterization, pharmacologic and surgical interventions, functional electrical stimulation, as well as urethral stents. In particular, neurogenic detrusor overactivity and detrusor sphincter dyssynergia are commonly managed with anti-muscarinic drugs and Botox injections, and while these pharmacotherapy agents do not prevent or reverse the changes, they primarily focus on improving storage outcomes and do not address the emptying phase. Furthermore, anti-muscarinic agents can have unpleasant side effects such as dry mouth and constipation, exacerbating existing bowel dysfunction. The primary goal in managing neurogenic bladder is to protect the upper urinary tract and reduce the incidence of infections, followed by achieving continence. As such, when conservative management fails, surgeries to reduce high bladder pressures and chronic urinary incontinence include urinary diversion and lower urinary tract reconstruction approaches ( ). As urinary complications continue to impact long-term morbidity in this population, additional therapeutic and rehabilitative approaches are needed that aim to improve function by targeting the recovery of underlying impairments.

Bowel dysfunction after SCI
Complications with bowel storage and evacuation are highly prevalent after SCI, with 95% of patients reporting issues with constipation ( ). Also, 75% report episodes of fecal incontinence with 30% considering bowel disorders more burdensome than bladder ( ) and having a substantial negative impact on quality of life, social integration, and overall independence ( ). Neurogenic bowel dysfunction can be characterized by the presence of increased colonic wall and anal tone along with weak abdominal musculature, resulting in fecal retention and constipation. A significant risk of incontinence can occur from an inability to adequately engage the external anal sphincter and pelvic floor musculature ( ). While gastrointestinal transit is primarily influenced by local enteric reflexes, motility patterns can be modulated by extrinsic innervation derived from sympathetic, parasympathetic and vagal inputs. Due to difficulties with elimination, prolonged colonic transit time, and impaired motor dexterity ( ), large amounts of time are devoted to bowel care programs (for some, up to 2 h) ( ; ). Many individuals with SCI are also dependent on caregiver assistance. As a result, many choose to conduct their programs every other day ( ), which may increase the risk of constipation, fecal impaction, colorectal distension, and episodes of AD. Conservative bowel regimen approaches include the use of laxatives and stool softeners to obtain a desired consistency, followed by rectal stimulation with either suppositories, enemas, or digital stimulation. Digital stimulation involves rectal pressure with a gloved finger to activate the recto-colic reflex, generating coordinated colonic peristaltic activity and increased motility ( ). Consequently, there is tremendous interest to use neuromodulatory strategies to achieve same or better effects.
Cardiovascular dysfunction and interactions with other autonomic systems after SCI
Cardiovascular diseases are the leading causes of morbidity and mortality among the SCI population ( ). Hemodynamic and metabolic cardiovascular effects occur in both the acute and chronic phases of SCI. Disruptions of sympathetic pathways to the heart and vasculature cause autonomic dysfunction and baroreflex impairment resulting in a reduced ability of cardiovascular system to maintain stable blood pressure after SCI. Dysregulation leads to persistent hypotension, bradycardia, orthostatic hypotension, and episodes of AD which drastically impacts quality of life by affecting health and disrupting engagement in daily activities ( ). Combinations of sympathetic agonists and venous compression devices are the primary measures currently used for symptomatic management. Manifestations of the reduced quality of life experienced is evidenced by reductions in peripheral blood flow, resulting in an increased risk of pressure ulcers, parasympathetic imbalance increasing vasovagal syncope, fatigue and cognitive dysfunction, and symptomatic orthostasis ( ). With time, post-injury blood pressure instability becomes more prominent and persists in many individuals with SCI for the rest of their lives ( ). AD, characterized by episodes of acute hypertension, is generated by unmodulated sympathetic reflexes below the injury level, and is typically accompanied by compensatory baroreceptor-mediated bradycardia ( ). Distention or contraction of hollow organs, such as bladder and bowel, are the most common triggers of AD ( ). Such below-level peripheral afferent stimuli reach the isolated spinal cord and activate a massive unmodulated sympathetic reflex causing widespread vasoconstriction, presenting as severe hypertension. With high-level SCI, the additional interruption of descending modulatory autonomic pathways that normally inhibit sympathetic preganglionic neurons during hypertension allows AD to persist until the stimulus is removed ( ) ( Fig. 2 ). Management and mitigation of unstable blood pressure is therefore a high consumer priority in SCI care; however, therapies available that have demonstrated the ability to resolve chronic cardiovascular dysfunction are lacking ( ; ). With time, post-injury episodes of AD become more prominent and persist long-term. The ability to recover bladder function such as, increasing bladder capacity, minimizing detrusor instability and improving bladder pressure and emptying, is limited by such severe fluctuations in blood pressure. Furthermore, improvements in bowel function are challenged by regular occurrences of AD symptoms during bowel programs, evoked by distention ( ) ( Fig. 3 ). The extreme dysregulation of the cardiovascular system underscores the importance of addressing these multi-faceted autonomic complications.


Introduction of spinal cord stimulation
Melzack and Wall’s publication of the Gate Theory ( ) transformed our understanding of pain mechanisms and the associated clinical management, leading to the realization that pain was the result of complex dynamic processes in the nervous system and not simply the result of activity in a hard-wired system. The concept that damage to the nervous system can itself result in chronic pain led to a gradual shift from ablative and destructive surgical approaches, such as cutting nerves, toward reversible neuromodulatory strategies such as therapeutic electrical stimulation of the dorsal spinal cord. The theory that nociceptive impulses, carried by Aδ and C fibers, could be inhibited by electrical stimulation of thick myelinated Aβ fibers provided the first scientific basis for the use of stimulation for pain. At that time, implanted electrode-induced electrical stimulation of the dorsal columns was initially introduced in 1967 in an effort to treat chronic intractable pain ( ). Its use was expanded to treat a wide variety of pain disorders following improvements in sensorimotor function in the multiple sclerosis population ( ) and was first used to treat neuropathic pain in SCI in the early 1970s ( ; ). Subsequently, the US Food and Drug Administration (FDA) approved the use of spinal cord stimulation in 1989 for the treatment of chronic intractable pain of the trunk or limbs and now accounts for the majority of all neuromodulation interventions ( ).
Initial applications of spinal cord epidural stimulation (scES), which served to clinically control severe spasticity in SCI ( ), led to the examination of whether scES had the capacity to drive locomotor capabilities through excitation of the lumbosacral motor circuitry ( ). By activating sophisticated spinal networks below the level of injury, scES functions by reactivating spared neural pathways and bridging supraspinal connectivity across the lesion with the goal of improving recovery after SCI. In persons with motor complete SCI, rhythmic, locomotor-like EMG activity and tonic motor patterns of the lower limbs from the supine position (in the absence of afferent input) were induced with scES ( ). Harkema and colleagues then demonstrated that a critical level of excitability, provided by scES and coupled with intense training that provides appropriate task-specific sensory cues, was necessary to facilitate standing and stepping, as well as intentional movement in clinically motor complete SCI ( ; ). The combination of locomotor training (stepping and standing) plus scES not only enhanced coordinated and controlled voluntary motor behavior, but was also reported by individuals to improve physiologic outcomes such as bladder, bowel, and sexual function ( ; ; ), as depicted in Fig. 4 . A summary of spinal cord stimulation approaches is provided in Table 1 .

STIM type | Visceral target | Study model | Author/date | Journal |
---|---|---|---|---|
Epidural | Bladder | Rats | PLOS One | |
Rats | Exp Neurol | |||
Rats a | Exp Neurol | |||
Rats | Front Sys Neurosci | |||
Rats | Sci Reports | |||
Rats a | Sci Reports | |||
Pigs a | IEEE Trans Neural Syst Rehabil Eng | |||
Human | Acta Neurochir (Wien) | |||
Human | J Pain Symptom manage | |||
Human | Sci Reports | |||
Human | Front Physiol | |||
Human | Oper Neurosurg | |||
Human | J Neurotrauma | |||
Human | Front Sys Neurosci | |||
Bowel | Rats | Sci Reports | ||
Pigs a | IEEE Trans Neural Syst Rehabil Eng | |||
Human | Front Physiol | |||
Human | J Neurotrauma | |||
Cardiovascular | Rats, Rhesus Macaques | Nature | ||
Human | Neurosurgery | |||
Human | Front Human Neurosci | |||
Human | JAMA Neurol | |||
Human | Front Physiol | |||
Human | JAMA Neurol | |||
Human | Clinical Auton Res | |||
Human | Exp Physiol | |||
Human | Front Neurosci | |||
Human | Front Sys Neurosci | |||
Human | J Neurotrauma | |||
Human | Nature | |||
Intra-spinal | Bladder | Mice | AJP, Renal Physiology | |
Rats a | Neurosci Lett | |||
Cats a | Brain Res | |||
Cats a | Exp Br Res | |||
Cats | Exp Neurol | |||
Cats | J Neural Eng | |||
Cats | J Neural Eng | |||
Dogs | Stereotact Funct Neurosurg | |||
Human | Arch Surg | |||
Human | Paraplegia | |||
Cardiovascular | Rats a | ( ) | Journal of Pain | |
Bowel | Cats a | Brain Res | ||
Transcutaneous | Bladder | Mice | (trans-spinal) | J Neural Eng |
Rhesus Macaques a | Exp Neurol | |||
Human | J Neurotrauma | |||
Human | Front Neurosci | |||
Human | Sci Reports | |||
Human | Front Sys Neurosci | |||
Bowel | Human | (magnetic) | J Rehab Medicine | |
Human | (magnetic) | Arch Phys Med Rehab | ||
Cardiovascular | Rats | Neurotherapeutics | ||
Human | J Neurotrauma |
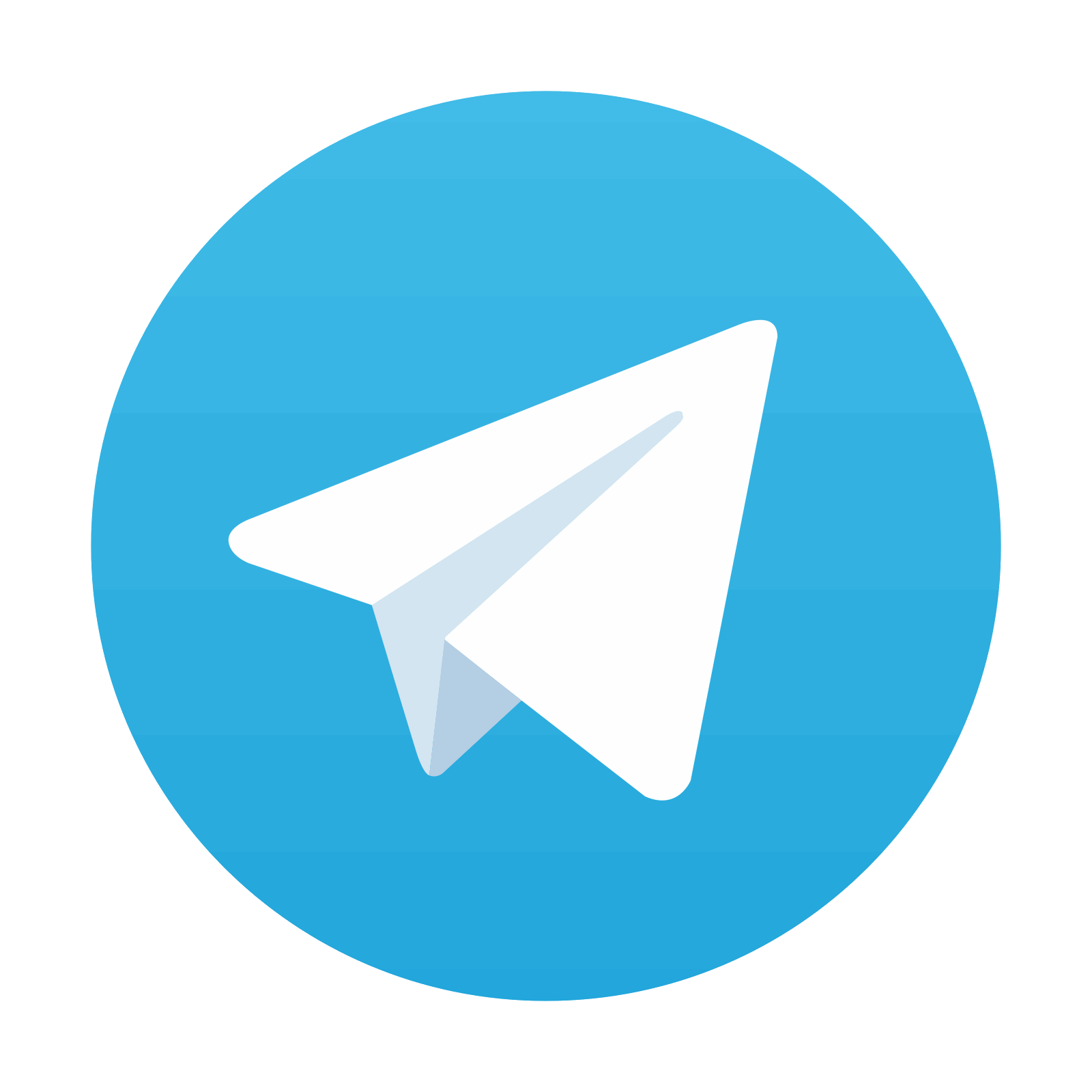
Stay updated, free articles. Join our Telegram channel

Full access? Get Clinical Tree
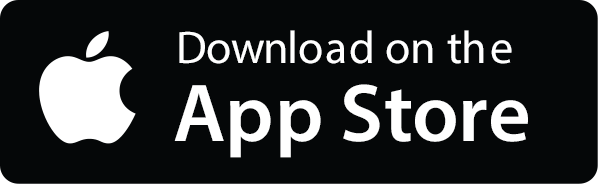
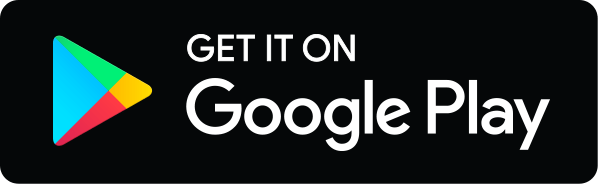