Primary
Incidence (%)
Breast
13–22
Lung
15–19
Prostate
10–18
Lymphoma
8–10
Sarcoma
7.5–9
Kidney
6–7
Gastrointestinal
4–5
Melanoma
2–4
Myeloma
4.5–5
Unknown
4–11
Pathogenesis and Pathophysiology
Batson, through his cadaveric experiments, identified the low-pressure valveless vertebral-venous plexus, which extends from epidural and perivertebral veins to veins of the thoraco-abdominal wall and veins of the head and neck. Venous blood can bypass the portal, caval, and pulmonary veins via Valsalva, venous obstruction, or increased intra-thoracic and intra-abdominal pressure resulting in flow inversion to the vertebral-venous plexus. This provides a pathway for distant organs to spread disease to the spine [23, 24].
An alternative route for metastasis to the bony spine and epidural space is via arterial emboli through the rich vascular network that supplies the bony spine. The vertebral body has a large blood supply, while the posterior elements are less highly vascular. Spinal lesions arise more often from the vertebral body; [5] however, isolated involvement of the vertebral body is rarely observed—only in 3.8% of cases—while 75% of ESM involve the vertebral body, pedicle and posterior elements [25]. These tumors can grow within the anterior or posterior bony elements or spread to the epidural space via venous drainage [26]. In addition, invasion into the spine can occur via direct extension from the paraspinal region to the nerve roots through the neural foramina [1].
The pathophysiology by which spine metastasis causes neurologic injury is a matter of some debate. Spinal cord compression is associated with endogenous neurochemical changes that lead to neuronal injury. This compression was initially thought to result in arterial ischemia. Subsequent animal and human studies have demonstrated that compression and obstruction of the vertebral-venous plexus result in vasogenic spinal cord edema, venous hemorrhage, and ischemia [20, 27]. In addition to venous obstruction, spinal auto-regulatory mechanisms induce arteriolar dilatation and increased edema via induction of such enzymes as nitric oxide synthase. Cytokine production, e.g., PGF2, IL-1, IL-6, locally promotes an inflammatory response with vasodilatation and increased edema formation. In addition, animal studies display myelin loss secondary to ischemia and compression [28–31].
Presentation
Patients harboring spinal metastases can present in a variety of ways. Symptoms secondary to metastasis are the same symptoms by which a primary spinal malignancy is discovered. Also, 20% of patients with metastatic cancer have signs and symptoms of ESM as the initial manifestation of their disease [32]. Patients with bony spine metastasis with or without an epidural component commonly present with a prolonged period of persistent back pain with a median time course of 8 weeks. Unfortunately, although back pain is a frequent complaint among the general populace, the physician must consider spine metastasis in the differential diagnosis. This is especially true in older patients and patients with pain at the level of the thoracic spine, as pain at this level is uncommon in degenerative disc disease. Even with the diagnostic modalities available to the modern physician, patients are diagnosed very late in the course of their disease. Levack and colleagues performed a prospective observational study of 319 patients and found that 82% of patients at diagnosis of ESM were either unable to walk or only able to do so with help. 94% of these patients reported approximately a 3-month history of axial spine pain [33]. It is important to note that patients with compression secondary to epidural disease can still present with isolated axial spine pain without neurologic deficit or radicular symptoms. Epidural spinal compression cannot be excluded because a patient with back pain does not manifest myelopathy or radiculopathy.
Pain may occur for a variety of reasons including pathologic fracture, local compression resulting in axial spine pain, or via nerve root impingement resulting in radicular pain. Radicular pain affecting the upper or lower extremities is seen in cervical and lumbar disease, whereas thoracic cord lesions present with bilateral pain radiating around the chest or upper abdomen [34].
The location of pain can help guide the physician; however, pain can be a false localizing sign and may not always correlate directly with the level of the metastasis. The differential diagnosis must also include more common entities such as herniated disc disease, which can be distinguished by a history of trauma or other inciting event as a well an acute onset of pain, rather than the more common insidious onset of pain symptoms seen in spine metastasis [35, 36].
Weakness is the second or third most common complaint of patients with spine metastasis and is both a symptom and sign of disease. Subjective weakness may be a manifestation of axial or radicular pain without true weakness evident on examination and is present in a high percentage of patients with ESM. Objective weakness is seen in 84% of patients with compressive ESM. At the time of diagnosis, approximately 50% are ambulatory, 35% are paraparetic, and 15% are paraplegic. Rapid diagnosis and treatment are critical in these patients as 30% of those individuals presenting with weakness become paraplegic within one week [21].
Patients with spinal metastasis commonly present with numbness and paresthesias such that 51% have subjective sensory symptoms on presentation, and 78% of patients have sensory deficits found on examination [21]. These deficits can assist in localizing the metastatic lesion. Dermatomal sensory loss or reflex loss is more predictive than a sensory level, as the sensory level may be apparent between one and four levels below the level of disease. Patients with cervical and thoracic disease can also present with Lhermitte’s sign [37].
Bowel or bladder dysfunction is seen in as many as 57% of patients. Urinary retention is the most common form of dysfunction, more common than both urinary and fecal incontinence. The degree of autonomic abnormality often correlates with the severity of motor and sensory deficits and is considered a late finding. In addition to the aforementioned presenting signs and symptoms, patients may also present with other forms of autonomic dysfunction such as the absence of sweating below the lesion level and Horner’s syndrome as well as ataxia, spasticity, and syringomyelia [20, 21].
In assessing patients with ESM, it is important to differentiate between lesions causing myelopathy from spinal cord compression and those causing deficits from cauda equina syndrome (CES) . Patients with either lesion can present with back pain, weakness, sensory deficits, or bowel and bladder dysfunction. However, the former results in upper motor neuron signs such as clonus, Babinski sign, and hyperactive reflexes. On the other hand, the latter displays unique sensory deficits such as saddle anesthesia as well as lower motor neuron signs such as hypoactive reflexes and muscle wasting [38, 39].
Diagnostic Work-up
The diagnosis of spine metastasis is continually evolving as the diagnostic tools available to the physician continue to improve. Plain X-rays are a valuable tool in analyzing the bony spine. Plain radiographs detect bony erosion better in cortical bone than in cancellous bone. The pedicle is primarily composed of cortical bone as compared to the vertebral body, so metastasis to the pedicle is identified first on plain radiographs despite the higher degree of involvement in the vertebral body [16]. Metastatic tumors are commonly lytic lesions that present with vertebral body compression. Plain radiographs also show paraspinal soft-tissue shadows and pathological fracture-dislocation [40, 41]. Despite these advantages, false-negative plain radiographs occur in 10–17% of patients with ESM [18]. The osteoblastic tumors seen in prostate and breast metastases as well as paraspinal tumors that invade the neural foramen are difficult to identify on plain radiographs.
Bone scans are more sensitive in assessing metastatic disease than plain radiographs. Bone scans use technetium diphosphonate to identify diseased bone which present as “hot spots.” This diagnostic method has the advantage of providing a survey of the entire skeleton. Degenerative changes seen in elderly patients can show up as “hot spots” and complicate the diagnosis [42, 43]. As an alternative to a conventional bone scan, whole body positron emission tomography (PET) can be used to assess bony metastases. This imaging modality has been shown to have equal sensitivity and improved accuracy in detecting metastatic bone lesions when compared to a bone scan [44]. The improved accuracy is related to the mechanism by which the modalities detect tumor involvement—technetium scanning relies upon osteoblastic bone response to tumor, while PET measures glucose uptake in the tumor itself by the use of a radiotracer [45–48]. Consequently, PET scans are more likely to detect tumors that are at an earlier stage of growth, while bone scans are less likely to detect osteolytic and slow growing metastases [48, 49].
Myelography , as first brought forward by Jean Athanase Sicard , has been an important diagnostic technique in the evaluation of spinal metastasis [50]. Prior to the advent of MRI, myelography was the gold standard for evaluation of these tumors [51]. Myelography can still be used to identify the site and extent of metastasis when MRI is not readily available, a patient is unable to tolerate MRI, or MRI is contraindicated as in patients with ferromagnetic implants [41, 52]. The relationship of the metastasis to the spinal cord, dura, and nerve roots can also be discerned. Myelography is primarily performed via lumbar injection of radio-opaque dye. However, some metastases can present in multiple locations; a tumor causing complete obliteration of the spinal canal results in failure to identify additional rostral metastases, in which case a cisternal injection is required to complete the evaluation [41].
Computed tomography (CT) can be used either as a separate modality or in combination with myelography. CT imaging is primarily useful in assessing the bony elements surrounding the spinal cord. CT in combination with myelography can greatly improve the data available from each study alone and can provide better anatomical detail of the spinal axis and extent of the tumor both inside and outside the spinal canal [41, 53].
While the aforementioned modalities can be of value in assessing spinal metastasis, magnetic resonance imaging (MRI) is considered the modality of choice. MRI provides multi-planar imaging of the spine that is noninvasive. In addition, paravertebral soft-tissue masses and bone marrow involvement can also be detected [51]. MRI has been shown to be equivalent if not superior to CT myelography in detecting cord compression in ESM as well as cord atrophy [54]. MRI has been shown to be the most accurate and noninvasive method to assess the entire spinal axis so that the appropriate treatment modality can be initiated [55].
Laboratory and radiographic assessment are important in assessing systemic disease. Metabolic panels, blood counts, and prealbumin should be obtained to assess the nutritional status and immunological status of the patient. Renal dysfunction and liver dysfunction via metastasis or primary disease can be assessed via blood urea nitrogen and creatinine and liver function tests [41]. Tumor markers such as prostate specific antigen, serum and urine protein electrophoresis for myeloma, CA-125 for ovarian cancer, and CEA for colorectal cancer can assist with diagnosis [56]. Urinalysis, chest radiography, abdominal ultrasound, and CT imaging of the chest, abdomen, and pelvis are useful screening methods for systemic malignancy [41].
Prognosis
In discussing the various treatment options of spinal metastasis, multiple factors must be considered in order to determine the patient’s prognosis. Prognosis can be a key item in patient assessment that can influence how aggressive the treatment is for a particular patient. Tokuhashi generated such a system for assessing prognosis that is useful in based on length of survival (Table 6.2). This system includes such items as general condition, number of extraspinal metastasis, the number of spinal metastasis, the extent of metastasis to internal organs, the primary site of the tumor, and the degree of spinal cord injury. This scoring system has been correlated with prognosis such that patients with a score between 9 and 12 are predicted to survive greater than 12 months, while patients with a score from 0 to 5 are predicted to survive less than 3 months [57].
Table 6.2
Tokuhashi’s evaluation for prognosis
Score | |||
---|---|---|---|
Symptoms | 0 | 1 | 2 |
Karnofsky score | 10–40 | 50–70 | 80–100 |
Extraspinal metastasis | >3 | 1–2 | 0 |
Internal organ metastasis | Unresectable | Resectable | No metastasis |
Primary site of tumor | Lung, stomach | Kidney, liver, uterus | Thyroid, prostate, breast, rectum |
Spinal metastasis | >3 | 2 | 1 |
Spinal cord injury | Complete | Incomplete | None |
In general, the median time of survival after diagnosis of ESM is approximately 6 months. Patients who are ambulatory at the time of diagnosis display a median survival of 8–10 months as compared to 2–4 months for non-ambulatory patients. In addition, patients with slow growing cancers such as breast and prostate cancer tend to liver longer than faster growing cancers such as lung cancer. The former has a median survival of 9–10 months, while the latter has a median survival of 3 months [58, 59].
Pharmacotherapy
The treatment options for spinal metastasis are divided into three categories. These options include pharmacologic therapy, radiation therapy, and surgical resection with or without fusion. Treatment of the patient’s symptoms and adjuvant therapy, e.g., corticosteroids or chemotherapy, are two roles of pharmacologic therapy. The physician combines medications for analgesia as well as for the control of neuropathic pain with radiation therapy and/or surgical intervention. Opiates are the primary treatment for analgesia, while amytriptiline and gabapentin or pregabalin are effective treatment options for neuropathic pain. These medications are a key component to palliative therapy [34].
Pain develops not only from bony infiltration but also from pathologic fractures. In addition to pain, fractures can lead to spinal instability requiring surgical intervention. Pharmacotherapy directed at bone turnover can provide a method in preventing pathologic compression fractures. Bisphosphonates inhibit osteoclast activity and bony resorption, decreasing the risk of pathologic fracture. Such therapy has shown a benefit in patients with bony metastasis from multiple myeloma and breast cancer [60, 61].
Corticosteroids have been shown in experimental models to reduce peritmoral vasogenic spinal cord edema and transiently improve neurologic function. After initiation of steroid therapy, patients have significant improvement in pain symptoms [34, 41, 62]. A randomized trial by Sorensen and colleagues compared outcome in patients receiving high-dose radiation therapy with or without dexamethasone. In the former group, 81% of patients were ambulatory after treatment and 59% of patients remained ambulatory after 6 months. In contrast, only 63% of patients in the latter group were ambulatory after treatment and 33% of patients remained ambulatory after 6 months. These differences displayed statistical significance identifying the importance of corticosteroids as adjuvant treatment in patients with ESM [63].
Studies have also focused on the effect of high-dose bolus dexamethasone (100 mg) versus moderate-dose bolus dexamethasone (10 mg) versus no corticosteroid treatment. These studies displayed equivalent efficacy between doses with regard to improvement in pain, ambulatory status, and bladder function. The physician must consider the side effect profile of corticosteroids, especially at higher dosages. Significant adverse side effects include severe psychoses, gastric ulcers, rectal bleeding, and gastrointestinal perforations [63–66]. Heimdal and colleagues performed a retrospective study of patients who received radiation therapy in combination with corticosteroids. All patients received pretreatment with antacids or H2 blockers prior to high-dose corticosteroid therapy. Despite preventive measures, two patients developed gastric perforations and two patients developed gastrointestinal bleeding, one of which proved fatal. A subsequent cohort of patients received a lower dose corticosteroid regimen of 16 mg tapered over 2 weeks. These patients did not experience serious side effects and the ambulatory outcome was similar to those patients receiving the high-dose corticosteroid regimen [66]. In addition, the use of corticosteroids has been analyzed in patients with less severe metastatic disease. Maranzano and colleagues analyzed 20 consecutive patients with ESM causing less than 50% narrowing of the spinal canal and no neurologic deficit in a phase II trial. Patients treated with corticosteroids and radiation therapy showed no additional survival benefit and equivalent return of neurologic function in comparison to patients treated with radiation therapy alone [67].
Chemotherapeutic agents can be a valuable treatment option in ESM. The primary use of these agents is dependent on the chemosensitivity or chemoresistance of the particular tumor. Treatment must be designed to maintain neurologic function and maximize quality of life. Consequently, chemotherapy is typically used as adjuvant therapy along with radiation therapy and/or surgical resection in tumors with uncertain or limited chemosensitivity. On the other hand, the role of chemotherapy in chemosensitive tumors has been a matter of debate. Patients with symptomatic chemosensitive metastases have most often been given chemotherapy in combination with other therapeutic modalities. However, patients with chemosensitive tumors have shown good neurologic improvement with chemotherapy alone. Especially early in the course of the disease, chemosensitive tumors are likely to respond to chemotherapy. These tumors include germ cell tumors and hematological malignancies, such as lymphoma [68, 69]. In addition, chemotherapy can be considered as a single mode of treatment for patients who have previously received radiation or surgery and are not candidates for further treatment [34].
Radiation Therapy
Historically, decompression via laminectomy was considered the primary treatment for spinal metastasis. Studies were conducted in the 1970s and 1980s comparing radiation therapy alone to laminectomy followed by adjuvant radiation therapy. These studies displayed similar rates of neurologic improvement. Consequently, radiation therapy became the standard as primary treatment, while surgery was reserved for patients who deteriorated during or failed to improve after radiation therapy [70–72]. As more advanced surgical techniques for resection and stabilization have been developed, the role of radiotherapy has also been modified.
Prognosis for patients with spinal metastasis receiving radiotherapy is highly dependent upon the radiosensitivity of the primary tumor. The most radiosensitive tumors that commonly metastasize to the spine include breast cancer, small cell lung cancer, prostate cancer, myeloma, and lymphoma. Patients with these tumors tend to show improved functional recovery and better tumor control rates as compared to patients with radioresistent tumors such as melanoma and renal cell carcinoma. Patients with radioresistent tumors can, however, still obtain significant pain control and quality of life improvement from radiotherapy [73–75].
A variety of techniques are available for the effective delivery of radiotherapy. Such techniques include but are not limited to conventional radiotherapy and stereotactic ablative radiation therapy. In general, the use of radiotherapy is limited by the level of radiation tolerance of the spinal cord. Hypertension, advanced age, prior spinal cord pathology, combination chemotherapy, and immunosuppression are factors which lower the spinal cord’s tolerance to radiation [76]. This tolerance level is not fully understood, which makes it necessary to limit the radiation dose provided to the spinal cord in order to prevent such serious complications as radiation myelitis or myelopathy. When radiation myelopathy occurs, patients have progressive rostral spread of sensorimotor symptoms within months of radiation therapy. Within 1 year of symptomatic onset, MRI displays cord swelling as high T2 signal within the cord with gadolinium enhancement. The combination of clinical and imaging characteristics is used to differentiate between radiation toxicity and tumor recurrence [77–80].
Although utilized with decreasing frequency now, conventional external beam radiation therapy (EBRT) has been historically considered an excellent treatment option for pain associated with bony spinal metastases commonly seen in lung, breast, and prostate cancer. This pain can be treated with various dose schedules with equal efficacy [81–83]. Notably, a retrospective analysis of 1300 patients compared five different treatment regimens between 8 Gy in 1 fraction and 40 Gy in 20 fractions. Functional outcome was similar between all groups; however, the more protracted regimens were associated with a lower rate of local recurrence [84]. A protracted regimen is subsequently advantageous in patients with a longer life expectancy, versus a short course of radiation for palliation for short life expectances [85].
Stereotactic ablative body radiation therapy (SABR) provides an alternate modality for focused high-dose radiation to the tumor while minimizing radiation to the adjacent spinal cord, and has become the dominant modality of radiation delivery to spinal metastases. Accurate targeting requires localization of multiple radiation beams to converge on the lesion of interest at a high dose. The typical dose ranges from 8 to 18 Gy. This treatment can be administered over multiple sessions, which makes outpatient treatment convenient. Gerstzen and colleagues presented 500 consecutive lesions treated with high-dose (15–22.5 Gy) single-fraction SABR with a median follow-up of 21 months. They reported long-term tumor control of 88% overall with 100% for breast, lung, and renal metastases [86]. A series by Bate and colleagues followed 57 patients treated with SABR with or without surgery, the SABR-only group achieved local tumor control of 96% overall [87]. In another study, the tumor control rate was 100% in lesions without previous irradiation [88]. SABR following surgery should be considered when mechanical stability and local tumor control both are required. Laufer and colleagues reported 186 patients with ESM treated with local debulking and spinal stabilization plus SABR, and compared radiotherapy groups. Both groups of high-dose therapy following decompression surgery achieved local recurrence rates below 10% [89]. Radiosurgery is considered safer for recurrent tumors than traditional methods as repeat traditional radiotherapy poses a significant risk at surpassing the radiation tolerance of the spinal cord. Moreover, recent review of the literature advocates SABR as the first-line treatment for palliative symptom control for those with symptomatic radioresistant tumors with no neurologic deficit (Fig. 6.1a–c) [90]. Overall, SABR is becoming a common method for delivering safe doses of radiation to spinal tumors. Radiosurgery, like other forms of radiotherapy, does not address the issue of spinal instability.


Fig. 6.1
a–c 61-year-old male presenting with a 2-month history of progressively worsening neck pain. The patient was neurologically intact on examination. He was recently diagnosed with metastatic thyroid carcinoma; a relatively radioresistant tumor. Pretreatment sagittal (a) and axial (b) T1 post-gadolinium MR image displaying a tumor in the left C2 vertebral body (green arrow). c CTV (red circle) and PTV (purple circle) for SABR treatment planning
Surgical Management
The role of surgery in the treatment of spine metastasis has changed as the techniques available for spinal reconstruction have improved. Despite the variety of options in a surgeon’s armamentarium, the ability to maintain a patient’s quality of life remains of utmost importance and extensive spinal instrumentation correlates with a longer and more painful recovery period, in addition to the recovery required for treatment of the primary disease. Consequently, prognosis is a key factor in deciding the aggressiveness of treatment for a particular patient.
Various scoring systems have been proposed to help guide treatment strategies and the role of surgical intervention . Tomita and colleagues clarified the correlation between length of survival and surgical treatment goals. In this study, they analyzed the growth rate of primary tumor, the presence of visceral metastasis, and the presence and number of bone metastasis. Slow growth tumors such as breast and thyroid cancer equated to 1 point; moderate growth tumors such as renal cell carcinoma equated to 2 points; rapid growth tumors such as lung and gastrointestinal cancer equated to 4 points. Visceral metastases equated to 2 points if they were treatable, while untreatable lesions equated to 4 points. Finally, solitary or isolated bony metastasis equated to 1 point, while multiple metastases equated to 2 points. The group then separated patients into prognostic scores from 2 to 10. Patients with a prognostic score of 2–3 had a treatment goal of long-term local control via wide or marginal excision, e.g., en bloc spondylectomy, with a mean survival time of 38.2 months. Patients with a prognostic score of 4–5 had a treatment goal of middle term local control via intralesional excision with a mean survival time of 21.5 months. Patients with a prognostic score of 6–7 had a treatment goal of short-term palliation via simple decompression and stabilization with a mean survival time of 10.1 months. Finally, patients with a prognostic score of 8–10 had supportive care only with a mean survival time of 5.3 months [7].
Bilsky and coworkers developed a scoring system that integrates neurologic assessment, oncologic assessment, assessment of mechanical instability, and an assessment of systemic disease burden and medical co-morbidity (NOMS). The neurologic component addresses myelopathy, radiculopathy, and degree of epidural compression. Oncologic assessment evaluates the radiosensitivity of the tumor. Radiosensitive tumors include multiple myeloma and lymphoma while highly radioresistant tumors include renal cell carcinoma, thyroid carcinoma, melanoma, and sarcoma [91].
On the other hand, the Spinal Instability Score Neoplastic Score (aka, SINS) can help the surgeon predict spinal stability with respect to neoplastic lesions. A composite score of six different factors will help determine if a spine is stable (score 0–6), intermediate (score 7–12), or unstable (score 13–18). Those factors are: location of lesion in the spine, mechanical or postural pain, bone lesion quality, spinal alignment, vertebral body involvement (i.e., collapse), and the posterolateral involvement of spinal elements [92].
The simplest therapeutic option—percutaneous vertebroplasty or kyphoplasty—is a valuable option for treating pain from lesions either isolated to the vertebral body or lesions with a mild epidural component. In this treatment, the surgeon injects polymethylmethacrylate (PMMA) either directly into the vertebral body in vertebroplasty or after expansion of the collapsed vertebral body with a balloon in kyphoplasty. The surgeon usually injects bone cement via a transpedicular route; however, anterolateral, intercostovertebral, and posterolateral routes are used in the cervical, thoracic, and lumbar regions, respectively. Patients can experience pain relief within 24–48 h following therapy and have shown to maintain improved pain control upon 2-year follow-up examination [93, 94].
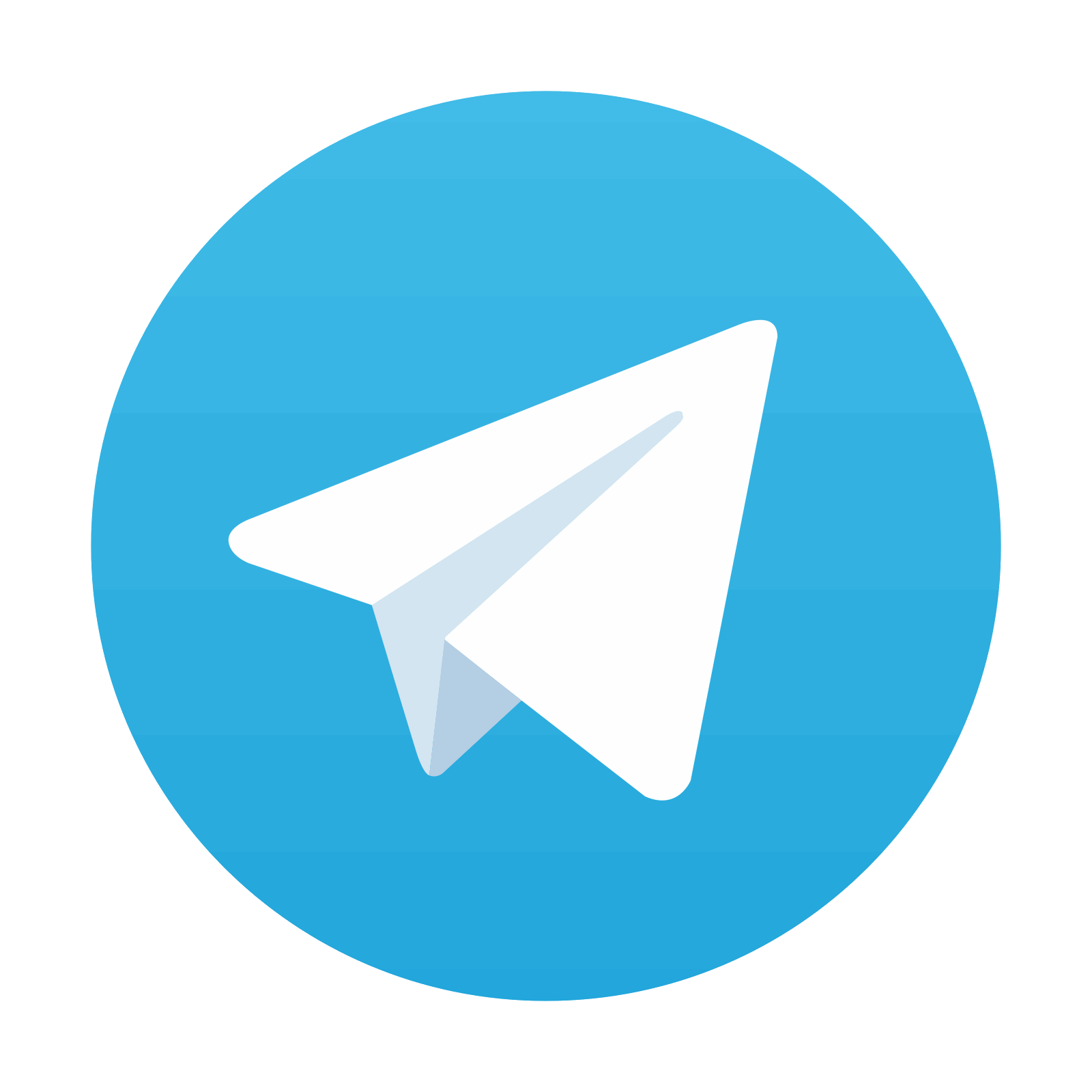
Stay updated, free articles. Join our Telegram channel

Full access? Get Clinical Tree
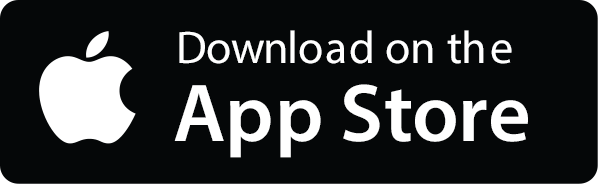
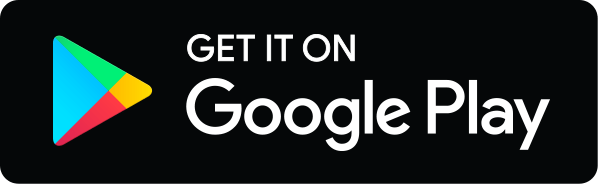