3 Spine There are seven cervical vertebral bodies and eight cervical nerves. C1 is the atlas, a bony ring. C2 is the axis, with the dens extending superiorly. From C3 to C7 there is a gradual increase in size of the vertebral bodies. The uncinate processes are bilateral superior projections from the C3 to the C7 vertebral bodies that indent the disk and vertebral body above (posterolaterally), forming the uncovertebral joints. Laterally, the transverse foramen lies within the transverse process, and contains the vertebral artery. There is a normal slight increase in spinal cord size extending from C4 to C6. The neural foramina course anterolaterally at a 45-degree angle with a slight inferior course. These thus lie oblique to the standard sagittal and axial imaging planes. In the cervical region, the epidural venous plexus is prominent, with sparse epidural fat, the opposite to some extent of that in the lumbar spine. In regard to dermatomes, the hand is innervated by C6 (the thumb), C7 (the middle finger), and C8 (the little finger). Within any specific neural foramen, the nerve within the foramen is that corresponding numerically to the level below—for example, the C5 nerve lies within the foramen at C4–5. There are 12 thoracic vertebral bodies. The ribs articulate with the vertebrae both at the disk level and at the transverse process (although for the latter only at T1–T10). The exit foramina for the basivertebral veins (part of the internal vertebral venous plexus) lie posteriorly within the mid-vertebral body, with the channel within the vertebral body itself often visualized as a Y in the axial plane (in both the thoracic and lumbar regions). In the thoracic region, epidural fat is often prominent posterior to the thecal sac and dura. Transitional type vertebrae are not uncommon at the thoracolumbar junction. In the lumbosacral spine, there are five lumbar segments (vertebral bodies), five (fused) sacral segments, and four coccygeal bones (the coccyx). At all levels in the spine, the intervertebral disks are composed of a central gelatinous core (the nucleus pulposus, high signal-intensity [SI] on T2-weighted scans) with surrounding dense fibrous tissue (the annulus fibrosus, with low SI on T1- and T2-weighted scans). Because of the increased size of the intervertebral disks in the lumbar region, differentiation of the hydrated nucleus from the annulus fibrosus is easily recognized in normal disks. The bony parts of the lumbar vertebrae include the pedicles, transverse processes, articular pillars (pars interarticularis, superior and inferior articular facets), laminae, spinous processes, and vertebral body. The facet joints are diarthrodial synovial-lined and richly innervated. On axial imaging the superior articular facet forms a “cap” anterolaterally with the inferior articular facet posteromedial connecting to the lamina. The ligamenta flava (bilateral paired ligaments, which connect the lamina of adjacent vertebrae, and are present from C2 to S1) extend from the anterior aspect of the upper lamina to the posterior aspect of the lower lamina. The epidural venous plexus is less prominent than in the cervical spine. In regard to dermatomes, the foot is innervated by L4 (medial big toe), L5 (midfoot), and S1 (little toe). Within any specific neural foramen (for both the thoracic and lumbar spine), the nerve within the foramen is that corresponding numerically to the level above—for example, the L4 nerve lies within the foramen at L4–5. Transitional vertebrae at the lumbosacral junction are quite common, with an incidence near 10%. A single anterior spinal artery and two paired posterior spinal arteries supply the spinal cord. These vessels are fed by anterior and posterior radiculomedullary arteries. The major anterior radiculomedullary artery in the thoracic and lumbar region is the artery of Adamkiewicz, which usually arises between T9 and L1. There are typically no substantial anterior radiculomedullary feeders inferior to the artery of Adamkiewicz. In the sagittal plane, the conus is commonly described in textbooks and in the anatomy literature to be located at L1–2. This statement is somewhat misleading. Looking specifically at the location of the tip of the conus, from published studies, this ranges in normal individuals from 10% occurring at the upper third of L1 to 10% occurring at the upper third of L2, with the maximum percentage (25%) occurring at the lower third of L1. A common clinical statement from magnetic resonance (MR) is that the conus in normal patients should lie at the T12–L1 level, with this statement referring to the conus itself and not specifically the tip. The posterior longitudinal ligament (which extends from the body of the axis to the sacrum) lies posterior to the vertebral bodies and anterior to the thecal sac, being in the lumbar region about 1 mm thick (anteroposterior [AP]) and 5 mm wide (left to right). The facet joints of the upper lumbar spine are oriented predominantly in the sagittal plane, while those of the lower lumbar spine are oriented more in the coronal plane. On off-midline sagittal images, the dorsal root ganglion (and ventral root) is seen to lie in the superior portion of the neural foramen. In the lumbar region in particular, the sagittal plane is important for evaluation of foraminal narrowing, equal or greater in value than the axial plane. In regard to the neural foramen, the margins are composed of the disk and vertebral body anteriorly, the pedicles superiorly and inferiorly, and the facet joints posteriorly. In the axial plane, the bony canal and the thecal sac are well visualized, with the vertebral body anteriorly, pedicles laterally, and lamina posteriorly. Because of its complexity, a description of the normal appearance of the spine on MR, specifically looking at the different sequences and parameter weighting, is important. T1-weighted images depict fat (and specifically the vertebral body marrow) as high SI, the spinal cord and intervertebral disks as intermediate SI (on very high quality images, gray and white matter within the cord can be distinguished on the basis of SI, on both T1- and T2-weighted scans), and cerebrospinal fluid (CSF) as low SI. Normally hydrated (nondegenerated) disks will appear slightly hypointense to the vertebral marrow. The normal ligamentum flavum is well seen, with intermediate SI. On thin section axial imaging, particularly in the lumbar spine, positioning of the slice relative to the vertebral body, end-plate, and disk can be differentiated on the basis of signal intensity, which changes from slightly high signal intensity (the fatty marrow of the vertebral body), to low (the endplate), to intermediate (the intervertebral disk). These structures can also be differentiated in a similar fashion on axial T2-weighted scans. Sagittal T1-weighted images (obtained without any obliquity) depict the neural foramina poorly due to their oblique orientation (except in the lower lumbar spine). T1-weighted scans typically can be acquired in a short scan time (2 to 4 minutes), with high signal-to-noise ratio (SNR) and high spatial resolution. Their general utility is for detection of structural abnormalities, marrow infiltration, degenerative disease, and abnormal contrast enhancement (following administration of a gadolinium chelate). On T2-weighted images (specifically as typically acquired today, with fast spin echo technique), CSF and hydrated disks will have high SI, spinal cord intermediate SI, muscle low SI, and fat (including specifically that within the vertebral body marrow) intermediate to high SI. In the sagittal plane in adults, a central band of low SI is seen in normal disks, best visualized in the lumbar region due to the size of the disks. This structure, the “intranuclear cleft,” is due to fibrous transformation and is observed in all normal disks in patients over the age of 30. Lack of visualization of the intranuclear cleft should be considered abnormal, with such absence common in early degenerative disk disease. For improved visualization of soft tissue and marrow abnormalities on the basis of T2 changes, fat saturation should be employed in combination with T2-weighted fast spin echo imaging. The thecal sac is well assessed on T2-weighted images, with this scan technique also important for detection of spinal cord abnormalities (edema, gliosis, demyelination, and neoplasia). Low flip angle gradient echo imaging is commonly employed in the cervical spine for axial T2-weighted imaging. On these scans, CSF and the intervertebral disks have high SI, the cord intermediate SI, and the marrow usually low SI (due to magnetic susceptibility effects, unless the scan is acquired with three-dimensional [3D] technique and a very short echo time [TE]). Good gray–white matter differentiation is seen within the cord. The utility of these scans is for detection of degenerative changes (disk herniation, canal compromise, foraminal stenosis) and evaluation of intrinsic cord abnormalities (multiple sclerosis [MS], tumors, edema, and hemorrhage). There may be a slight exaggeration of canal and foraminal stenosis due to magnetic susceptibility effects, in particular if the TE is substantially greater than 10 msec. This technique is (as with all gradient echo techniques) sensitive to, and commonly markedly degraded by, the presence of metal. On MR there is a complex temporal evolution of vertebral body and disk appearance, in the infant, which is beyond the scope of this textbook. In the time frame from young adulthood to advanced age, the appearance of vertebral body marrow on MR is determined by the combination of red and yellow marrow, the former hematologically active marrow with lower T1 signal intensity when compared to yellow (fatty) marrow. With normal aging, there is a conversion from red to yellow marrow, which is reflected by higher signal intensity on T1-weighted scans of the vertebral marrow. With increasing age, both diffuse and focal replacement of red by yellow marrow occurs. Focal fat, as the latter is termed, is easily seen (due to its high signal intensity), and commonly encountered, on T1-weighted scans. Focal fat is seen more often in the elderly, and is identified as such by its isointensity to fat on all MR pulse sequences. Attention should be paid to a few additional specifics in regard to MR acquisition technique, in order to obtain high-quality images. For both sagittal and axial acquisitions, if the phase encoding gradient is in the AP dimension, placement of a coronal saturation pulse (pre-saturation slab) is necessary to eliminate motion artifacts that might otherwise substantially degrade the image. In the cervical spine this includes motion of the mouth and swallowing. In the thoracic spine, cardiac motion can otherwise be a substantial problem. And in the lumbar spine motion artifacts originate from the aorta, vena cava, internal organs, and anterior abdominal fat (with respiration). For spine imaging the maximum slice thickness that should be acquired in any plane and any portion of the spine is 3 mm (Fig. 3.1). On fast spin echo T2-weighted scans, signal loss within the thecal sac is common due to CSF pulsation, with this problem being most prominent in the cervical region (on axial images) and to a lesser extent in the thoracic region (on both axial and sagittal images). Axial images for optimal clinical interpretation should be angled parallel to the disk space, with state-of-the-art MR systems offering accurate and automatic vertebral body numbering as well as angling of axial images parallel to the disk space in both the AP and right to left dimensions. Image normalization is advocated in the lumbar spine in particular for routine use on axial images. Because of the use in MR of surface coils for spine imaging, structures located superficially, and thus close to the coil, will have artifactual high SI. When the window and center are chosen for display of the spinal canal, the posterior soft tissue structures are often obscured, being depicted with very high signal intensity due to their proximity to the surface coil. Image normalization is a post-processing feature that takes into account the sensitivity of the coil, and enables the visualization of structures both close and distant relative to the receiver coil, providing more homogeneous signal intensity across the field of view. It is important also to realize that today receiver coil coverage is integrated and continuous, allowing imaging of the spine in its entirety without gaps or image registration problems. Signal intensity drop offat the edge of the field of view, in the craniocaudal dimension, should not occur, and is indicative of an operator error. Thus, anatomic regions that were difficult to image in the past due to technical issues, including specifically the cervicothoracic and the thoracolumbar junctions, are well visualized on modern scanners (Fig. 3.2). Fig. 3.1 Right paracentral disk herniation at L5–S1, evaluated on high-resolution thin section imaging at 3T. One problem in MR regarding the evaluation of disk herniations is the large thickness of the slice relative to the disk and relevant portion of the affected nerves. Illustrated are contiguous high in-plane spatial resolution 2-mm thick axial images. Here, the right S1 nerve root (arrows) can be followed on each section, initially exiting from the thecal sac, subsequently being compressed and displaced (both posteriorly and laterally) to varying degrees by the adjacent disk herniation, and subsequently recovering its normal shape just prior to its exit in the neural foramen. Following intravenous contrast enhancement, normal enhancing structures include, in particular, the venous plexus. The external vertebral plexus is a network of veins along the anterior vertebral body, laminae, and spinous, transverse, and articular processes. The internal vertebral plexus is a network of veins in the epidural space (the “epidural venous plexus”), both anteriorly and posteriorly. The basivertebral veins, seen on sagittal imaging centrally within the vertebral body, drain posteriorly into the anterior internal vertebral plexus. The anterior plexus is larger, with longitudinal veins on each side of posterior longitudinal ligament, which taper at the disk space level. Displacement and engorgement often accompany a disk herniation. These venous structures all drain via intervertebral veins that accompany spinal nerves within the neural foramina. An additional normal enhancing structure is the dorsal root ganglion. The most common indication for administration of intravenous contrast in spine MR imaging is in the postoperative back (predominantly the lumbar spine) for the differentiation of scar from disk. On scans obtained within 20 minutes following contrast injection, scar enhances whereas recurrent or residual disk material does not. There will be prominent enhancement of the normal epidural venous plexus, which also improves depiction of the neural foramina and abnormal soft tissue therein. Contrast enhancement is also used routinely for evaluation of intradural, and soft tissue extradural, neoplastic disease. For extradural neoplasia, acquisition of post-contrast scans using fat saturation markedly improves recognition of abnormal contrast enhancement. Contrast enhancement is not normally administered when the clinical question is that of vertebral metastatic disease. This pathology is well-delineated in most cases without intravenous contrast administration. In certain instances, contrast administration can improve the depiction of vertebral metastatic disease. However, when used for this indication, scans must be performed with fat saturation. Otherwise the low signal intensity of a metastatic lesion (on a T1-weighted scan) may enhance to near isointensity with the adjacent high signal intensity of fatty marrow, reducing conspicuity. Contrast enhancement is also routinely used for other disease processes that involve the spinal cord, in particular ischemia and demyelination. Infection, whether intradural or extradural, is an additional major indication for contrast administration on MR. Fig. 3.2 Pancoast (superior sulcus) tumor. An apical soft tissue lung mass is noted, seen both in the sagittal (part 1) and axial (part 2) planes, with extensive involvement of the chest wall, ribs, and adjacent spine (specifically the facets, transverse process, pedicle, and lateral vertebral body). There is no spinal canal compromise. The lesion demonstrates inhomogeneous contrast enhancement, with the suggestion of a central necrotic (nonenhancing) component. An apical lung mass with adjacent bone involvement should be considered to be bronchogenic carcinoma unless otherwise proven. Imaging of the spine in CT is typically performed without intravenous contrast administration. Depending on clinical symptoms and indications, intrathecal contrast administration may be performed prior to CT for improved depiction of the thecal sac, cord and nerve roots therein, and nerve root sleeves. It should be kept in mind that spine imaging by both CT and MR is acquired in non-load bearing and on occasion may not reflect the positioning and pathology that would be present if the patient were imaged in an erect position. Flexion and extension views, which are extensively used in plain radiography, could be obtained with both computed tomography (CT) and MR but only in a limited fashion, and are not part of routine clinical practice. It is also important to be familiar with the following terminology in spine imaging, which applies to lesion localization. An extradural (epidural) mass is one that is located outside the dura, and thus can cause, when of sufficient size, compression of the thecal sac. An intradural–extramedullary mass is one located within the thecal sac, but external to the cord. Such a lesion produces a filling defect in the CSF, outlined by a sharp meniscus above and below, with the ipsilateral subarachnoid space enlarged up to the level of the mass and the spinal cord deviated away from the mass. An intramedullary mass is one that involves (arises within) the spinal cord, and produces smooth cord enlargement with gradual effacement of the subarachnoid space. A block, which is an old term originating from myelography, is a level at which the subarachnoid space is obliterated. Depending on the location of the lesion causing the block, the thecal sac may be displaced (if extradural), and there can be cord compression (if the compression occurs at the level of, or above, the conus). Transitional vertebrae are a common anomaly at the lumbosacral junction, occurring in 5 to 10% of patients. There can be lumbarization of S1, or sacralization of L5 (Fig. 3.3). Key identifying features include a disk at L5–S1 that is small (the L5–S1 disk should be the largest of the lumbar disks), and the absence of a normal sacral curvature in the sagittal plane. Also helpful is the position of the conus. With modern MR scanners, a relatively high-resolution sagittal scout can be obtained of the entire spine, allowing a count from the dens (C2), which is readily recognizable, and providing the most reliable count of the vertebrae. On CT, articulation or fusion of an enlarged transverse process of the lowest lumbar segment to the sacrum can be seen (unilaterally or bilaterally). There will be decreased mobility at the affected level, with increased mobility and stress at the interspace immediately above. A not uncommon appearance on MR of the lumbar spine, well seen in the sagittal plane, is an apparent normal L5–S1 with degenerative findings at L4–5. This likely reflects, in some patients, the lack of normal mobility at L5–S1, and thus in a sense a transitional vertebra. In the literature, a transitional lumbosacral spine is reported as a known cause of back pain. Fig. 3.3 Transitional vertebra. Lateral and AP radiographs are presented in a patient with a sacralized L5. The count was established on the basis of the chest x-ray. There were 12 rib-bearing vertebral bodies, with the left rib at T12 small, and the right rib vestigial (and poorly visualized on the AP view). Nonsegmentation of L5 and the sacrum bilaterally is well seen on the AP radiograph. The lateral radiograph demonstrates the L5–S1 disk space to be small, and absence of the normal sacral curvature. Both findings can be key to recognition on MR of a transitional vertebra at the lumbosacral junction. Fig. 3.4 Congenital cervical vertebral body nonsegmentation (single level). Well seen on sagittal and coronal CT is nonsegmentation of C4–5. Note in this instance that there is nonsegmentation both anteriorly (vertebral body) and posteriorly (arrow, spinous process). The vertebral body segment is slightly narrowed in the AP dimension at the level of nonsegmentation, a characteristic finding. On CT there is little evidence of a residual disk space, which is typically more evident on MR. In a more broad sense, transitional vertebrae or variants therein can also be seen in the cervical and thoracic spine. Although much less common than variants at the lumbosacral junction, variants involving the dens and the thoracolumbar junction are well known. In a very small number of patients, a fusion anomaly of C2 and C3 can be seen, with its most apparent feature been the unusually long craniocaudal dimension of the dens. With regard to the thoracolumbar junction, the presence of either 11 or 13 thoracic vertebral bodies, as opposed to the normal 12, is known. Vestigial ribs at L1 are a common incidental finding on CT. A single level, congenital, cervical vertebral nonsegmentation can also be considered in a sense a normal variant (Fig. 3.4). Although a nonsegmentation will predispose the patient to accentuated degenerative disease at the levels above and below (due to slightly greater than usual motion at those levels), by itself the entity is asymptomatic and an observed variant in patients imaged by MR and CT for other reasons. On MR a small vestigial disk is commonly visualized. The shape of the nonsegmentation is distinct, as seen in the sagittal plane, with the two vertebrae not appearing as a rectangle, but rather with a smooth inward curvature toward the fusion level (seen with a narrow “waist” in the AP dimension). By shape, this entity can easily be distinguished from a postsurgical fusion, in which both vertebrae retain their normal rectangular shape. Craniovertebral junction anomalies include failure of segmentation between the skull base and C1, also referred to as atlantooccipital assimilation (or occipitalization of the atlas), which can be partial or complete (with assimilation of the lateral masses of C1 and the adjacent occipital condyles). Another distinct entity, a midline posterior cleft in the bony arch of the atlas, is quite common, in autopsy series seen in 4% (Fig. 3.5). An anterior arch cleft is much less common, and is typically seen in association with a cleft posteriorly (split atlas). An os odontoideum is a well-corticated ovoid ossicle, located superior to a hypoplastic odontoid. Its etiology is controversial, and has been ascribed to both congenital and acquired causes. Current thinking is that an os odontoideum results from an undiagnosed fracture through the C2-odontoid growth plate before the age of 5 or 6. A common secondary finding is a hypertrophic appearance of the anterior arch of C1, as seen in the mid-sagittal plane, appearing rounded as opposed to the typical crescent shape (Fig. 3.6) Differentiation from an acute high type II odontoid fracture is important, which can be made on MR on the basis of the absence of associated soft tissue edema and on CT by the absence of a well-defined fracture line (extending through the cortex) and the absence of a hypertrophic anterior C1 arch. Fig. 3.5 Posterior fusion defect of C1. There is incomplete ossification of the arch of C1 posteriorly along the midline (arrow), seen on sagittal and axial CT. The margins of the defect are well corticated. This common congenital variant should not be mistaken for a fracture. A limbus vertebra is a small, triangular corticated bone fragment, corresponding to the anterosuperior (most common) or anteroinferior corner of a vertebral body, caused by herniation of nucleus pulposus beneath the apophysis. The most common location is midlumbar, with no treatment indicated (Fig. 3.7). The filum terminale extends from the tip of the conus to the end of the thecal sac, where it inserts on the first coccygeal segment. A diameter < 2 mm is normal, and the average filum measures about 1 mm in thickness. It is easily seen on high-resolution axial MR images. In < 5% of patients, a small amount of fat can be seen blending in with and extending along the filum terminale, without focal enlargement. This entity, a fatty filum terminale, is well seen on MR on T1-weighted scans, and is a normal variant. On occasion, a small focal collection of fat, incidental to any clinical symptomatology, can be observed adjacent to the conus (Fig. 3.8). Fig. 3.6 Os odontoideum. This anatomic variant is illustrated on both sagittal CT and MR images. The well-corticated os (arrow) lies above a hypoplastic odontoid process. Note the corticated margins on CT, the lack of edema on MR, and the hypertrophic anterior arch of C1, all characteristic for this variant, and distinguishing it from an acute type II odontoid fracture. Fig. 3.7 Congenital lumbar spinal stenosis. Despite evidence of mild degenerative changes, including disk dehydration and loss of disk space height, there is no compromise of the canal due to disk disease. The spinal canal is, nevertheless, small in AP dimension. Congenitally shortened pedicles—the etiology of this finding—are evident in the axial image. For the clinician recognition of this entity is important, as a congenitally narrowed canal will amplify the severity of any degenerative findings or trauma. Note the incidental limbus vertebra (arrow), involving L3 anteriorly and superiorly. Lumbosacral nerve root anomalies are seen in 1 to 3% of the population. The most common anomaly involves the roots of L5 and S1 unilaterally, with two roots arising from a single (conjoined) root sleeve, but then exiting separately in the appropriate foramina (Fig. 3.9). This entity is asymptomatic, but noteworthy for two reasons. On MR, when a disk herniation is identified at the level of a conjoined nerve root, description of this is important for operative planning. Alternatively, on CT due to poor soft tissue contrast, a conjoined nerve root can be mistaken for a herniated disk, if the film reader is not familiar with this normal variant. Fig. 3.9 Conjoined nerve root. This common entity has many variants, with the most frequently seen being that in which two nerves arise within a single root sleeve, which subsequently divides, with the respective nerves then exiting separately in the appropriate foramina. Illustrated is a conjoined L5–S1 nerve root sleeve on the left. The sections chosen show the two nerves (L5 and S1, labeled) on the left just after separation (arrow), on the slice just caudal to this (middle column), and then on a final more caudal axial slice (right column) where relative symmetry of right and left has been reestablished. The Nabors classification differentiates three types of spinal meningeal cysts. Types I and II are both extradural, with type I not containing and type II containing nerve roots. Type I includes IA, extradural arachnoid cysts, and IB, sacral meningoceles. Type II includes the commonly visualized Tarlov cysts, so prevalent in the sacrum, and the common very small foraminal cysts seen incidentally in the cervical and thoracic spine on MR (Fig. 3.10). Tarlov cysts are frequently large, multiple, and bilateral. When large, there will be scalloping of the surrounding bone (Fig. 3.11). Type III spinal meningeal cysts are intradural-extramedullary in location (and include arachnoid cysts, which can present with cord compression) (Fig. 3.12), and are most often seen in the thoracic spine dorsal to the cord. Spinal meningeal cysts (other than type IA) communicate with the subarachnoid space and contain CSF, and on MR will thus demonstrate CSF signal intensity on all pulse sequences. Clinically, spinal meningeal cysts, with the exception of type III, are usually asymptomatic. Lateral meningoceles are included in the discussion of incidental lesions, since most are asymptomatic. Eighty-five percent are seen in neurofibromatosis. With a lateral meningocele, there is protrusion laterally of the dura and arachnoid through an enlarged neural foramen, with scalloping of the pedicles, lamina, and dorsal surface of the vertebral body (Fig. 3.13). The lesions are usually solitary. A lateral meningocele will have CSF signal intensity on all MR pulse sequences. No measurements are absolute; however, AP dimensions of the spinal canal less than 10 mm in the cervical region (Fig. 3.14) and 12 mm in the lumbar region (see Fig. 3.7) are considered to be stenotic. To some extent canal dimensions are more critical in the cervical spine, due to the presence of the cord, and in this region AP diameters of 10 to 13 mm are considered to represent relative spinal stenosis. It should be noted that the normal dimensions of the cervical spinal canal vary according to anatomic level, sex, age, and height. One easily recognized imaging finding in congenital spinal stenosis of the lumbar region is tapering of the canal dimension from the upper to the lower lumbar levels, with this region normally equal in size or greater in dimensions when compared to the thoracic spine. Patients with congenital cervical spinal stenosis are predisposed to traumatic spinal cord injury. Clinical presentation with congenital cervical spinal stenosis is typically due to myelopathic symptoms. However, radicular symptoms may be present, in either cervical or lumbar congenital stenosis, due to nerve root impingement with narrowing of the lateral recesses or neural foramina. A major element of congenital spinal stenosis is short pedicles. Achondroplasia is well known for symptomatic lumbar stenosis, with the entire spine stenotic in some patients. Down syndrome is known for congenital stenosis of both the cervical and lumbar spine. Fig. 3.10 Type II spinal meningeal cyst (Tarlov perineural cyst, thoracic). Small cysts such as that illustrated are commonly noted on cervical and thoracic MR exams, as seen on the right at a lower thoracic level, and are incidental findings. Fig. 3.11 Type II spinal meningeal cysts (Tarlov perineural cysts). Tarlov cysts are the most frequently encountered spinal meningeal cysts in clinical practice. Such lesions typically involve the sacral nerve roots, and demonstrate CSF SI on all pulse sequences. In the example presented, Tarlov cysts involve the S2 and S3 nerve root sleeves on the right. Foraminal enlargement and posterior scalloping of the vertebral bodies are common. Scoliosis is simply lateral curvature of the spine. Ninety percent of cases are idiopathic with no underlying cause. The typical idiopathic scoliosis is an S-shape curve, with the thoracic curvature convex to the right. The remaining 10% are congenital (Fig. 3.15), neuromuscular, and post-traumatic in etiology. In congenital scoliosis, vertebral anomalies can be seen (e.g., a hemivertebra) (Fig. 3.16) and in other instances the scoliosis is associated with a congenital abnormality such as diastematomyelia or a Chiari I malformation (with hydromyelia). Neuromuscular causes include cerebral palsy, with an incidence as high as 50% in patients with severe disability as assessed by the gross motor function classification system. Posttraumatic etiologies include prior fracture, chronic osteomyelitis, prior surgery, and radiation therapy. Plain films are utilized for quantitation of the curvature and monitoring for possible progression. MR is the imaging modality of choice for evaluation of atypical or progressive scoliosis, specifically to exclude an underlying abnormality, with coronal imaging important (in addition to the more routinely acquired sagittal and axial scans). Fig. 3.13 Lateral meningoceles, neurofibromatosis type 1, CT. Sagittal and coronal reformatted CT scans (part 1) depict prominent scalloping of the L4 and L5 vertebral bodies, which can be identified as chronic in nature due to the thin sclerotic bony margin. The density therein was fluid (CSF) by attenuation measurement. In this patient, the meningoceles extended far beyond the spine into the adjacent soft tissues, with only the medial margin (white arrows) of their soft tissue extent identified on this cropped image. Volume rendering of the CT dataset (part 2) demonstrates both the vertebral body scalloping as well as thinning and deformity of the pedicles. This congenital anomaly is defined by a low position of the conus, with the conus being tethered (held in that position) due to an additional abnormality. Causes include a tight (often slightly thickened) filum terminale, lipomyelomeningocele (and variants thereof), diastematomyelia, and retethering following meningomyelocele repair (Fig. 3.17). The clinical presentation is typically that of a young child with progressive neurologic dysfunction, specifically gait disturbance, motor and sensory loss in the lower extremities, and bladder dysfunction (all presumably due to cord traction and ischemia). In a tight filum terminale there will be an identifiable conus. The most common appearance of a tethered cord, however, is that of the cord extending without change in caliber to the lumbosacral region, tethering posteriorly with an associated lipoma and dysraphic posterior spinal elements. There may be associated hydromyelia. Treatment is surgical with release of the tether, for prevention of symptom progression. Following release, the level of the conus/distal cord typically will not change. Fig. 3.14 Congenital cervical spinal stenosis. Sagittal images identify a reduced AP dimension to the cervical spine, with little CSF either anterior or posterior to the cord. Axial images are presented at the C4–5 and mid C5 levels. At C4–5 a broad-based disk osteophyte complex leads to moderate spinal canal stenosis with moderate cord flattening, which otherwise in a patient with a normal diameter canal would have had little impact. Note also that even at the mid C5 level there is markedly reduced CSF anterior and posterior to the cord, on axial imaging, with mild to moderate cord flattening. Hydromyelia is strictly defined as dilatation of the central canal of the spinal cord, lined by ependyma. Syringomyelia is defined strictly as the presence of a fluid-filled cavity within the spinal cord, lined by gliotic parenchyma, specifically not representing dilatation of the central canal. Unfortunately, these terms are commonly confused, and used interchangeably, by physicians. Thus the term syringohydromyelia has emerged, being less specific and including both hydromyelia and syringomyelia. Syringobulbia refers to the extension of a fluid collection into the brainstem, often accompanied by cranial nerve findings (due to compression). These entities are all difficult to visualize on CT. MR well depicts the cord and any pathology therein, and specifically abnormal fluid collections. The sagittal plane is commonly used to define the extent of syringohydromyelia, with the axial plane providing localization relative to the cross-section of the cord. Axial images also better identify very small cavities, and can determine with greater certainty the true extension of hydromyelia (with minimal dilatation of the central canal difficult to assess on sagittal images due to partial volume effects). Neoplastic disease and trauma are common etiologies for syringomyelia, whereas the Chiari I and II malformations account for the majority of cases of hydromyelia (Figs. 3.18 and 3.19). Intravenous contrast administration is strongly recommended in patients with syringomyelia, improving the sensitivity for detection of neoplastic disease. In the patient population at large, hydromyelia is much more commonly encountered, congenital in etiology, than syringomyelia due to either neoplasia or trauma. Both syringomyelia and hydromyelia can be treated by direct shunting of the cavity, amongst other options. An enlarging syrinx in a post-traumatic patient, for example following cervical cord injury, can cause neurologic deterioration unless so treated. With MR imaging at 3T now commonly available clinically, the normal central canal of the spinal cord is routinely visualized on high-resolution images, with the canal slightly smaller or larger (specifically with a range of normal diameters) depending on the individual (Fig. 3.20). In a meningomyelocele and a meningocele, there is incomplete closure of the posterior bony elements, with the contents of the spinal canal extending through the defect (open spinal dysraphism) (Fig. 3.21). A meningocele, by definition, contains only dura and arachnoid, with neurologic deficits uncommon. A meningomyelocele, by definition, contains neural tissue within the expanded posterior subarachnoid space, with cord tethering. Imaging studies are rarely acquired at presentation in the newborn, with the exposed neural placode readily evident (in a meningomyelocele) and surgery typically performed within 48 hours (Fig. 3.22). MR and CT are often obtained many years following surgery, and demonstrate a wide dysraphic defect with an accompanying CSF-filled sac covered by skin. Retethering is a common long-term complication. It is important to note that Chiari II malformations are virtually always associated with a meningomyelocele (Fig. 3.23). Fig. 3.15 Congenital scoliosis. Two curves are present, the first convex to the left in the cervical spine, and the second convex to the right in the thoracic spine. However, this is different from the more common idiopathic S-shaped scoliotic curvature, which is thoracolumbar in location, with the thoracic component convex to the right. There are nonsegmentation anomalies involving the skull base and upper cervical spine, together with an anomalous upper thoracic vertebral body (representing nonsegmentation of a normal vertebra and a hemivertebra) at the apex of the lower curvature. Fig. 3.16 Butterfly vertebral body. Coronal and axial images well depict a not uncommon vertebral body anomaly, a “butterfly” vertebral body. In this entity, there is failure of fusion of the two halves of the vertebral body due to persistent notochordal tissue centrally. Fig. 3.17 Tethered cord with a lipoma (retethering). This 12-year-old child had surgery at 2 years of age, with release of a tethered spinal cord and excision of a lipomeningocele. On the current exam, there is retethering, with the cord taunt and extending to a residual lipoma in the low sacral region. There is evidence of bony spinal dysraphism posteriorly. Fig. 3.19 Chiari I with extensive, marked hydromyelia. Wedge-shaped cerebellar tonsils are noted extending well below the C1 level. There is little if any CSF surrounding the brainstem at the level of the foramen magnum. There is marked dilatation of the central canal of the spinal cord, with a characteristic haustral-like appearance and the cord substance itself compressed peripherally. Complete evaluation of the cord from the foramen magnum to the conus is important in such cases, to establish the caudal extent of the central canal dilation, which may extend to the conus. Lipomyelomeningoceles (and lipomyeloceles) differ from the two entities just described by the presence of a lipoma attached to the dorsal surface of the cord termination and intact skin overlying the defect (closed spinal dysraphism) (Fig. 3.24). The lipoma extends through the dysraphic spinal canal merging with and becoming indistinguishable from, subcutaneous fat. The distal cord is tethered by the lipoma. When a mass is present posteriorly, it presents clinically under the age of 6 months (Fig. 3.25). If the mass is subtle, presentation may not be until 5 to 10 years of age when neurologic or urologic deficits are noticed. Occasionally this entity goes undetected until adulthood, since the lesion is skin-covered. In diastematomyelia the spinal cord is split into two hemicords, each invested by pia and each with a central canal, and dorsal and ventral horns. The two hemicords may lie within the same dural sac, or in separate sacs. A fibrous band or osteocartilaginous spur is often present at the most caudal aspect of the split (Fig. 3.26). Most cases are lower thoracic or lumbar in location. Vertebral segmentation anomalies are common, and the presence of multiple nonsegmented vertebrae in the thoracic region is a clue to the possible presence of diastematomyelia. Clinical presentation is often nonspecific. There may be signs related to cord tethering, and cutaneous stigmata may be present along the back. As with all congenital anomalies involving neural structures, MR is the modality of choice, with imaging in all three orthogonal planes mandated. Fig. 3.20 Normal central canal of the spinal cord. The central canal can be visualized on high-resolution MR images in some individuals, as seen on sagittal and axial images, appearing slightly prominent size wise but within the spectrum of normal. Fig. 3.22 Meningomyelocele, at birth. The midline sagittal T2-weighted image from the MR exam of this newborn, on the first day of life, is presented. A large spinal defect is noted posteriorly in the low lumbar region, with a CSF-filled sac protruding dorsally. It is very rare to ever see such a case on MR, given that surgery (repair with closure) is performed almost immediately. By definition, this defect lacks a skin covering, with neural tissue (and specifically the CSF sac) exposed to air. Fig. 3.23 Tethered cord in a patient with a Chiari II malformation. The typical tethered cord patient presents with neurologic dysfunction early in life, with many cases repaired at birth. Retethering may occur, as illustrated. The cord gradually tapers until reaching the end of the thecal sac, with no distinct conus—a typical appearance for either simple tethering or retethering. Nearly all Chiari II patients present at birth with a meningomyelocele, as was the case with this child. Fig. 3.24 Lipomyelocele. A single sagittal midline T2-weighted image demonstrates the cord extending to and tethered in the sacral region, without a change in caliber. There is an extensive posterior bony sacral defect, through which the cord continued terminating in a neural placode (visualized on adjacent off midline images, not presented) within the large dorsal lipoma. In caudal regression (sacral agenesis), there is absence of sacrococcygeal vertebrae, which in more severe cases extends to include a portion of the lumbar spine. The agenesis is limited to the sacrum in about half of cases. As might be expected, associated anomalies of the gastrointestinal and genitourinary systems are common, in particular anal atresia. Clinical findings range from mild deformities of the feet or distal muscle weakness to lower extremity paralysis, depending on the extent of vertebral involvement. Associated cord tethering is common. MR well depicts the level of regression together with the vertebral anomalies. In about half of patients (and specifically in patients without tethering) there is a characteristic, hatchetlike appearance (blunting, with the terminus slightly shorter along its ventral aspect) to the cord termination (Fig. 3.27). In this instance the cord terminus is usually above L1. Fig. 3.26 Diastematomyelia. Sagittal, coronal, and axial MR images depict multiple nonsegmented midthoracic vertebral bodies, two hemicords, and on the final axial image a bony spur separating the two cords. Vertebral segmentation anomalies are common with diastematomyelia, in particular block vertebrae, and thus the presence of the latter finding (on plain film or CT) should raise the question of diastematomyelia. An anterior sacral meningocele is a protrusion of the dura and leptomeninges, of varying size, anteriorly through a defect in the sacrum. The neck of the lesion is typically narrow. On sagittal CT or MR (or lateral plain film), the residual sacrum will be scalloped anteriorly and beneath the defect (in a semicircular manner), leading to the appearance of a scimitar or sickle (Fig. 3.28). On MR, the contents will be isointense with CSF on all pulse sequences. It is important to delineate on imaging whether nerve roots traverse the sacral defect to lie within the meningeal sac, which negates simple ligation of the neck of the meningocele. A dorsal dermal sinus is a midline epithelial-lined tract that extends from the skin inward to a variable distance. The tract extends to the spinal canal in about half of cases (a dorsal dermal sinus may terminate in soft tissue, at the dura, or within the thecal sac) (Fig. 3.29). Most are either lumbosacral (the largest percentage) or occipital in location. A dimple or small ostium may be seen on the skin, frequently associated with a hairy nevus, hyperpigmented patch, or capillary angioma. Symptoms occur due to infection or neural compression, the latter with an associated dermoid or epidermoid cyst (about half of cases have a dermoid or epidermoid cyst at the tract termination). Cord tethering is common in lumbosacral lesions. When infected, intravenous contrast enhancement on MR is recommended, and improves delineation of the sinus tract and visualization of associated inflammation. Fig. 3.28 Anterior sacral meningocele. Sagittal, coronal, and axial T2-weighted scans demonstrate a small presacral CSF collection contiguous with the thecal sac. There is also mild hydromyelia involving the distal cord, seen on the sagittal image. Characteristic accompanying findings include hypoplasia of the sacrum and, in the coronal plane, a curved “scimitar” shape to the sacrum. The Currarino syndrome (triad), present in this patient, consists of an anorectal malformation (typically with constipation), bony sacral defect, and presacral mass (anterior meningocele or benign teratoma). Note the dilated stool-filled rectum, reflecting the third part of the triad in this patient. Most patients present as adolescents or young adults with pain, and, on imaging, marked compression of the cord. The cyst itself can have variable contents, and thus a spectrum of signal intensity on MR (including that of CSF). The cyst is usually single, smooth, unilocular, intraduralextramedullary in location, and ventral or ventrolateral to the cord, occurring either in the cervical or thoracic region. There are associated vertebral anomalies in about half of patients (Fig. 3.30). The bony spinal canal may be focally expanded (due to local pressure effects). There will be no abnormal contrast enhancement, differentiating this lesion from neoplasia. An important additional differential diagnostic consideration is that of an arachnoid cyst (type III spinal meningeal cyst), which will have CSF signal intensity on all pulse sequences and is not associated with vertebral anomalies. Fig. 3.29 Dorsal dermal sinus. Sagittal midline images are presented in this infant, the first being at 2 months of age (left most image). Additional images (T1- and T2-weighted, and DWI – insert) are provided from a second exam at 9 months of age. On the initial exam a sinus tract is noted extending from the skin, posteriorly, to the thoracic cord. There is a small associated intradural lipoma. On the follow-up exam, the sinus tract is again noted, but now well seen is an intramedullary lesion, with low and high SI respectively on T1-and T2-weighted images, and restricted diffusion (high SI on DWI). This abnormality corresponds to a growing epidermoid (with an epidermoid or dermoid found, in association, in 50% of dermal sinus cases), which was resected a few weeks following the second exam. Idiopathic spinal cord herniation is a rare, known entity in the thoracic spine, and a cause of thoracic myelopathy. On sagittal MR scans there will be an abrupt ventral deviation of the cord, due to prolapse through an anterior or lateral dural tear (Fig. 3.31). An important diagnosis to exclude in this instance is a type III spinal meningeal arachnoid cyst, with both CT myelography and MR CSF flow studies permitting differentiation. Although of unknown etiology, a congenital origin has been postulated. These are not true neoplasms, and can be congenital (e.g., associated with a dorsal dermal sinus) or acquired (after surgery, lumbar puncture, or trauma) in origin. These cystic lesions are lined by squamous epithelium, with the presence of hair and skin elements (dermal appendages) defining a dermoid (as opposed to an epidermoid). Cyst rupture can occur, and can be asymptomatic or cause chemical meningitis. They are most commonly intraduralextramedullary, or intramedullary in location. Most spinal dermoid and epidermoid cysts arise in the lumbosacral region (Fig. 3.32). They are typically well-circumscribed round lesions. On both CT and MR the fat component of a dermoid can be readily recognized, although the proportion of fat varies markedly. Unless infected, these lesions do not enhance on MR (other than subtle enhancement of the capsule). On CT and MR, epidermoid cysts (which do not contain fat) have an appearance similar to CSF (and thus an arachnoid cyst), with diffusion-weighted imaging (DWI) (an epidermoid has marked hyperintensity on DWI) critical for differential diagnosis, and often for detection as well. An epidermoid cyst can also be differentiated from CSF on FLAIR, although the difference in signal intensity can be subtle (and, FLAIR scans are typically utilized only in the brain). In large dermoid and epidermoid cysts, there may be remodeling of the bony spinal canal. Spine manifestations in neurofibromatosis type 1 include posterior scalloping of the vertebral bodies (Fig. 3.33), lateral meningoceles, and neurofibromas of exiting nerve roots (with enlargement therein of the neural foramina) (Fig. 3.34). Multiple cutaneous neurofibromas are the hallmark of this disease. Plexiform neurofibromas of the paraspinal and sacral regions are also common. Neurofibromatosis type 1 occurs in about 1 in 3,000 births. On MR, neurofibromas characteristically are markedly hyperintense on T2-weighted scans. As with other neural origin tumors, enhancement is also seen following gadolinium chelate injection (Fig. 3.35). The incidence of neurofibromatosis type 2 (NF2) is approximately 5% that of type 1. Bilateral vestibular schwannomas are pathognomonic for this disease. Tumors involving the spine, and specifically intradural lesions, are very common in NF2. Extramedullary lesions include schwannomas and meningiomas, with neurofibromas less common. Intramedullary lesions include astrocytomas and ependymomas, although these are less common (in comparison with extramedullary lesions) (Fig. 3.36). Today Klippel-Feil is broadly defined to include any patient with failure of segmentation of two or more cervical vertebrae (most commonly C2–3 or C5–6) (Fig. 3.37). Most patients do not exhibit the classic clinical triad of short neck, low posterior hairline, and limited neck motion. Commonly associated anomalies include deafness, congenital heart disease, Sprengel deformity (elevation and rotation of the scapula), and urologic abnormalities. Nonsegmentation at one or two cervical levels is most common, and there may be associated occipitoatlantal nonsegmentation. Less common are patients with extensive cervical and thoracic nonsegmentation anomalies (Fig. 3.38). Patients are often asymptomatic. There can be cord and nerve root compression. Patients with more extensive abnormalities are predisposed to spinal cord injury following minor trauma. Diastematomyelia is present in a small percentage of patients with Klippel-Feil. Fig. 3.31 Idiopathic spinal cord herniation. The sagittal T2-weighted scan demonstrates focal anterior displacement (kinking) of the cord in the midthoracic region. On the axial scan, the cord is noted to have prolapsed through an anterolateral dural defect. The patient presented with spastic monoparesis of the right leg. Findings were confirmed at surgery, with reduction of the herniation. Achondroplasia, an autosomal dominant disorder, is the most common cause of dwarfism. In the lumbar spine, the interpedicular distance characteristically decreases from L1 to L5 and the pedicles will be short, leading to spinal stenosis. In the cervical spine there can be generalized spinal canal stenosis, with stenosis at the foramen magnum also known to be associated (potentially resulting in cervicomedullary compression). Fig. 3.33 Vertebral body scalloping, neurofibromatosis type 1, MR. There is posterior scalloping of L4, and to a lesser degree L5 (without any associated mass), seen on axial and sagittal images. Spine manifestations of NF1 include scoliosis, bony dysplasia, dural ectasia (with vertebral scalloping), and lateral meningoceles. Fig. 3.34 Neurofibromatosis type 1, CT. Sagittal and coronal reformatted CT sections demonstrate the neural foramina of the cervical spine to be diffusely enlarged, due to the presence of plexiform neurofibromas. In regard to the mechanisms of spine injury, and in particular referencing the cervical spine, flexion injuries lead to anteriorly wedged vertebral body fractures (Fig. 3.39). In a severe flexion injury there can be disruption of the posterior longitudinal ligament and the interspinous ligaments (Fig. 3.40), facet distraction, and anteroposterior subluxation (posterior ligament complex disruption). In the most severe cases, bilateral facet dislocation can occur. Extension injuries lead to posterior element fractures, and in a severe extension injury there can be disruption of the anterior longitudinal ligament and subluxation. In axial load injuries (e.g., from diving into shallow water), vertebral body compression (burst) fractures and lateral element fractures occur (Fig. 3.41). Fig. 3.35 Neurofibromatosis type 1, MR. There is diffuse enlargement of lumbar nerve roots within the neural foramina (which are also slightly enlarged), consistent with a diagnosis of NF1. The lesions are hyperintense on the T2-weighted scan, and demonstrate moderate contrast enhancement. This adolescent male patient has extensive plexiform neurofibromas, meeting the clinical criteria for diagnosis on this basis together with the presence of multiple café-au-lait spots. In high velocity auto accident injuries, axial load injury may result in compression of multiple contiguous vertebral bodies, in particular involving the thoracic spine (Fig. 3.42). Rotational injuries rarely occur in isolation, rather typically with flexion, and result in lateral mass fractures and unilateral facet subluxations or dislocations. Fig. 3.36 Neurofibromatosis type 2. Three small intramedullary lesions, with high signal intensity on the T2-weighted scan and abnormal enhancement post-contrast, are noted within the cervical cord, consistent with either small astrocytomas or ependymomas, both which occur in NF2. Note also the small enhancing meningioma, with a broad dural base adjacent to the posterior arch of C1. Fig. 3.37 Klippel-Feil syndrome. On the sagittal image, the C2–C4 vertebral bodies are hypoplastic and nonsegmented, with absence of normal intervening disk spaces. Diastematomyelia is identified on the axial scan at C1–2, with the two hemicords tethered by a fibrous band and enveloped in a single arachnoid-dural sheath. Fig. 3.38 Multiple cervical vertebral anomalies. Seen on sagittal and coronal MR these include hemivertebrae (C5, C6), nonsegmentation of the posterior elements (C1–2), and a wedge-shaped vertebral body (C7). A large osteophyte at C2–3 reflects accentuated movement at this level, with mobility of the cervical spine otherwise evidently restricted. Fig. 3.40 Interspinous ligament injury. On CT, this injury is visualized only indirectly by widening of the interspinous distance (*). On MR, edema within the ligament can be directly visualized (arrow), with the extensive posterior paraspinous edema/hemorrhage an additional indication of the severity of the injury in this patient. Fractures can be classified as stable or unstable on the basis of the three-column concept. The anterior column is defined as the anterior two-thirds of the vertebral body, the middle column the posterior one-third, and the posterior column extending from the posterior vertebral body margin to the tip of the spinous process. Injury of two of the three columns, or the middle column, is considered an unstable fracture. CT with multiplanar reformatted images is critical for the evaluation of osseous injury (including the assessment of bony canal compromise and the presence of bone fragments therein), with MR extremely valuable for evaluation of the spinal cord and injuries involving, or in which the important element is, soft tissue (e.g., an epidural hematoma, or acute disk herniation). Acquisition of T2-weighted scans with fat suppression (or alternatively the use of STIR) is important for the demonstration of marrow edema (and improved detection, as compared to CT, of vertebral body microfractures) and soft tissue injury (e.g., involving the paraspinal musculature). Although marrow edema is commonly seen on MR in acute fractures, it is not always present. In the cervical spine on CT, review of high-resolution reformatted sagittal and coronal images is mandatory, in addition to thin section (source) axial images. Fig. 3.41 Traumatic C3 burst fracture. An axial load injury has resulted in a burst fracture of C3, with mild loss of height and edema seen throughout the vertebral body. A vertically oriented, fracture plane is seen on the sagittal T2-weighted image extending through the C3 vertebral body. Retropulsion of the posterior portion of the C3 vertebral body into the spinal canal results in mild spinal canal compromise with mild cord flattening. There is no evidence of cord edema in this region. Edema in the posterosuperior portion of the C4 vertebral body is consistent with an additional acute fracture. Prevertebral soft tissue edema (only partially depicted) extended from the skull base to the C4 level. Fig. 3.43 C1 fracture. Because C1 is a ring, when fractured there will typically be at least two fracture lines (as in this instance, with fractures of both the anterior [black arrow] and posterior [white arrow] arches). The Jefferson fracture, of which this is an example, is a burst fracture due to axial loading. Specific osseous injuries in the cervical spine are subsequently discussed. Atlantooccipital dislocation (dissociation) occurs due to disruption of the ligaments between the occiput and C1. Increased distance is seen on coronal and sagittal reformatted CT images between the occipital condyles and the lateral masses of C1. MR visualizes both this finding and edema in the region of the disrupted stabilizing ligaments, reflecting the ligamentous injury itself. Atlantooccipital dislocation is often fatal. A Jefferson fracture is a burst fracture involving both the anterior and posterior arches of C1 (the atlas) (Fig. 3.43). Unless the transverse atlantal ligament is disrupted, the patient is usually neurologically intact. Odontoid fractures occur with both flexion and extension injuries, and are primarily transverse in orientation (and thus can be difficult to detect on axial images). They are classified according to the location of the fracture line. Type I involve the upper portion of the odontoid (Fig. 3.44), type II involve the junction of the odontoid and the body of C2 (these are the most common, and have the highest rate of nonunion), and type III extend into the body of C2 (Fig. 3.45). A Hangman fracture is a bilateral fracture of the C2 ring, which has many variants (Fig. 3.46). The pedicles and even the vertebral body may be involved. Extension of the fracture into the transverse foramen, as with all such fractures, raises the question of damage to the vertebral artery. The C2 vertebral body will be displaced anteriorly relative to C3—sometimes this is minimal—(but with the laminae still aligned) on the lateral plain film, and on sagittal CT or MR. As opposed to other fractures of the cervical spine that often compromise the spinal canal, these fractures often widen the canal and neurologic symptoms may be absent or minimal (autodecompression). A Clay-shoveler fracture is an avulsion fracture of a spinous process, involving a lower cervical or upper thoracic vertebra, classically C6 or C7 (Fig. 3.47). Fig. 3.44 Type I odontoid fracture. There is a mildly distracted fracture involving the tip of the odontoid, seen on sagittal and coronal reformatted images. A type I fracture involves only the superior portion of the dens, as distinguished from a type II fracture that involves the base of the dens (not illustrated). In this patient, there is likely an additional atlantoaxial dissociation injury, with increased distance between the articular facets of C1 and C2 on the right. Fig. 3.46 Traumatic spondylolisthesis of C2 (hangman fracture). Sagittal images reveal coronally oriented fractures (arrows) bilaterally of the pars interarticularis of the axis (C2). In this instance there is little displacement or angulation. A teardrop fracture occurs due to flexion in combination with axial compression, resulting in a fracture involving the anteroinferior aspect of a cervical vertebral body (Fig. 3.48). Bilateral facet fractures or dislocation occur due to flexion. A unilateral facet fracture involves both flexion and rotation. Vertebral body compression fractures can occur due to flexion (Fig. 3.49). Hyperflexion typically involves injury to the posterior musculature and posterior ligamentous complex (detected in part on MR due to the accompanying edema), together with facet fracture/subluxation/dislocation (Figs. 3.50 and 3.51). If the injury to the posterior paraspinal musculature is unilateral, the injury involved flexion with rotation. Fig. 3.47 Clay-shoveler fracture. Sagittal and axial CT images (part 1) depict a slightly displaced acute fracture of the C7 spinous process. Note the very sharp discontinuity of cortical bone, best seen on the axial section, defining this fracture as acute. On sagittal MR (part 2), the fracture of the spinous process can be identified (white arrow), but is more difficult to detect than on CT. Note the relative absence of edema within the bone adjacent to the fracture line, a common but nonintuitive finding in acute trauma. What CT does not depict however is the extensive nature and severity of the injury, reflected by the diffuse edema in the posterior paraspinal tissues (*) and the prevertebral edema/fluid (black arrow). Fig. 3.49 Compression fractures, acute, cervical spine. In trauma, a vertebral body may wedge anteriorly due to flexion. Or, with excess axial load, a compression (burst) fracture may result—this being the most common traumatic injury in the thoracic spine. The latter may manifest as a loss of vertebral body height, as seen in the T3 vertebral body (lower white arrow). However, body height may be maintained, rendering visualization impossible on plain film or CT. With MR these microfractures are easily identified due to marrow edema, in this instance with low signal intensity (upper arrow) on the T1-weighted scan within C7. Fig. 3.50 Severe hyperflexion injury, CT. Midline and right lateral sagittal reformatted CT images are presented (part 1), along with a single axial image at the C4 level (part 2). There is a fracture involving the left C4 lamina extending into the articular pillar and transverse foramen. The C4–C5 facet on the right is perched. Splaying of the C4–C5 spinous processes, consistent with interspinous ligament injury and instability, is also noted. Together these result in 4 mm of anterolisthesis of C4 on C5 with a mild acute kyphotic angulation at this level. This anterolisthesis and a broad-based disk bulge at the C4–5 level (not well seen on the images presented) result in mild AP dimension spinal canal narrowing at this level. Given the extent of injury, likely the entire posterior ligamentous complex is disrupted at C4–5. A burst fracture occurs secondary to axial loading, with vertical compression. Typically a single vertebral body is involved, with radial displacement of fragments. Burst fractures are most common in the lower thoracic and lumbar regions. Neurologic deficits result due to retropulsion of bone fragments into the spinal canal (Fig. 3.52). CT is the study of choice for initial evaluation, with MR providing potentially important supplemental information regarding the cord (and specifically damage therein, including edema and hemorrhage) and ligamentous injury (see Fig. 3.41). Fig. 3.51 Severe hyperflexion injury, MR. On the midline sagittal MR image, there is a traumatic anterolisthesis of C4 on C5, with an acute disk herniation, leading to mild canal narrowing and cord compression. There is splaying of the C4–5 spinous processes, and edema between, consistent with disruption of the interspinous ligament. On the off-midline image, a perched facet is also noted (arrow), implying at least an additional tear of the interfacetal ligaments. Flexion injuries of the spine, other than those involving the cervical spine, occur most commonly from T12 to L2. The injury is termed a Chance fracture, and consists of an anterior vertebral body compression fracture in combination with an injury involving the posterior elements (with a spectrum from ligamentous disruption to transverse fracture). There will be anterior wedging of the involved vertebral body. In the past this occurred due to the use of seat belts (prior to the introduction of shoulder belts), due to passenger restraint with sudden forward flexion in a head on collision. This injury is also seen in unrestrained occupants in a major motor vehicle accident. Osteoporotic compression fractures occur in the elderly, and are more common in postmenopausal women, due to bony insufficiency (Figs. 3.53 and 3.54). Although loss of height of a vertebral body is well identified on CT, discrete fractures (through cortical bone) are often not visualized, making CT poor for differentiation of acute from chronic changes (Fig. 3.55). MR readily identifies both the loss of height and edema therein, in acute benign vertebral body compression fractures. STIR is the favored imaging technique, with images acquired in the sagittal plane, for the detection of edema in an acute benign compression fracture (Fig. 3.56). Fig. 3.52 Burst fracture, acute, thoracic spine, CT. Sagittal, coronal (part 1), and axial (part 2) reformatted CT images are presented of the midthoracic spine, in the acute time frame following trauma. There is moderate loss of height of T7, with mild anterior wedging. Portions of the vertebral body are displaced (on the sagittal image) both anteriorly and posteriorly, and (on the coronal image) both to the left and right. Note the centrifugally located vertebral body fragments, best appreciated on the axial image. The edema is also well seen on T1-weighted and fat suppressed T2-weighted scans, but is more striking on STIR images, likely due to the combined T1- and T2-weighting of this sequence, with edema producing an increase in signal intensity by the change in both T1 and T2. The edema within the vertebral body also demonstrates abnormal contrast enhancement on scans acquired with fat suppression (Fig. 3.57). In an acute vertebral body compression fracture, the entire vertebral body may be involved, or simply a portion of the body immediately adjacent to, and parallel with, the intervertebral disk (e.g., involvement of the upper or lower one-third of a body). Surprisingly, in cases that involve the entirety of the vertebral body, identification of a discrete fracture line on MR (as a low signal intensity irregular line extending through the vertebral body) can be made on occasion, improving further diagnostic confidence. Fig. 3.53 Compression fracture, acute, L1 (CT). On the sagittal reformatted image, a mild anterior wedge compression deformity is noted, with an acute fracture suggested only indirectly (in this plane) by the sclerotic line (black arrow). This corresponds to compressed trabecular bone. CT is excellent for demonstration of acute fracture lines, in particular where they intersect cortical bone (small white arrow, axial plane), but insensitive to marrow edema and microfractures, which are well depicted by MR. Sacral insufficiency fractures can occur unilaterally or bilaterally, with marrow edema seen in the sacral alae. Although fracture lines and sclerosis may be seen on CT, MR is the imaging modality of choice for diagnosis, with substantially improved detection. A moderate in size, patchy area of edema will be visualized, and is typically well seen on T1-weighted, T2-weighted (with fat saturation) and STIR images (Fig. 3.58). Fig. 3.54 Compression fracture, acute and chronic, lumbar spine (CT). There is generalized osteopenia. There is an acute compression fracture of L3 with discrete fracture lines identified (arrows). There is approximately 50% loss of vertebral body height. There is moderate retropulsion of the posterior superior portion of the L3 vertebral body into the spinal canal. This leads to approximately one-third to one-half loss of bony central spinal canal dimensions in the AP direction. However, the level of canal compromise should be well below the termination of the conus, which could not be determined on this CT. Also demonstrated is a chronic anterior wedge compression deformity of L4, with the time frame of this deformity established by its presence on a prior exam. There is mild retropulsion of the superior posterior portion of this vertebral body into the spinal canal. Fig. 3.55 Compression fractures, osteoporotic (CT). There is mild loss of vertebral body height involving T6, with a prominent wedge compression deformity of T8. The accentuated vertical trabeculae and the demineralization of the spine reflect generalized osteoporosis. Note the accentuated kyphotic deformity of the thoracic spine. However, without demonstration of a discrete fracture line, neither compression deformity can be classified in regard to time frame.
Normal Anatomy, Imaging Technique, and Common Variants
Anatomy of the Normal Spine
Imaging Technique
Common Normal Variants, and Incidental Findings
Incidental Cystic Lesions
Congenital Disease
Congenital Spinal Stenosis
Scoliosis
Tethered Cord
Syringohydromyelia
Meningomyeloceles and Lipomyelomeningoceles
Diastematomyelia
Caudal Regression
Anterior Sacral Meningocele
Dorsal Dermal Sinus
Intraspinal Enteric Cyst (Neurenteric Cyst)
Spinal Cord Herniation
Dermoid and Epidermoid Cysts
Neurofibromatosis
Klippel-Feil
Achondroplasia
Trauma
Cervical Spine Trauma
Burst Fracture
Flexion Injury
Benign Osteoporotic Fractures
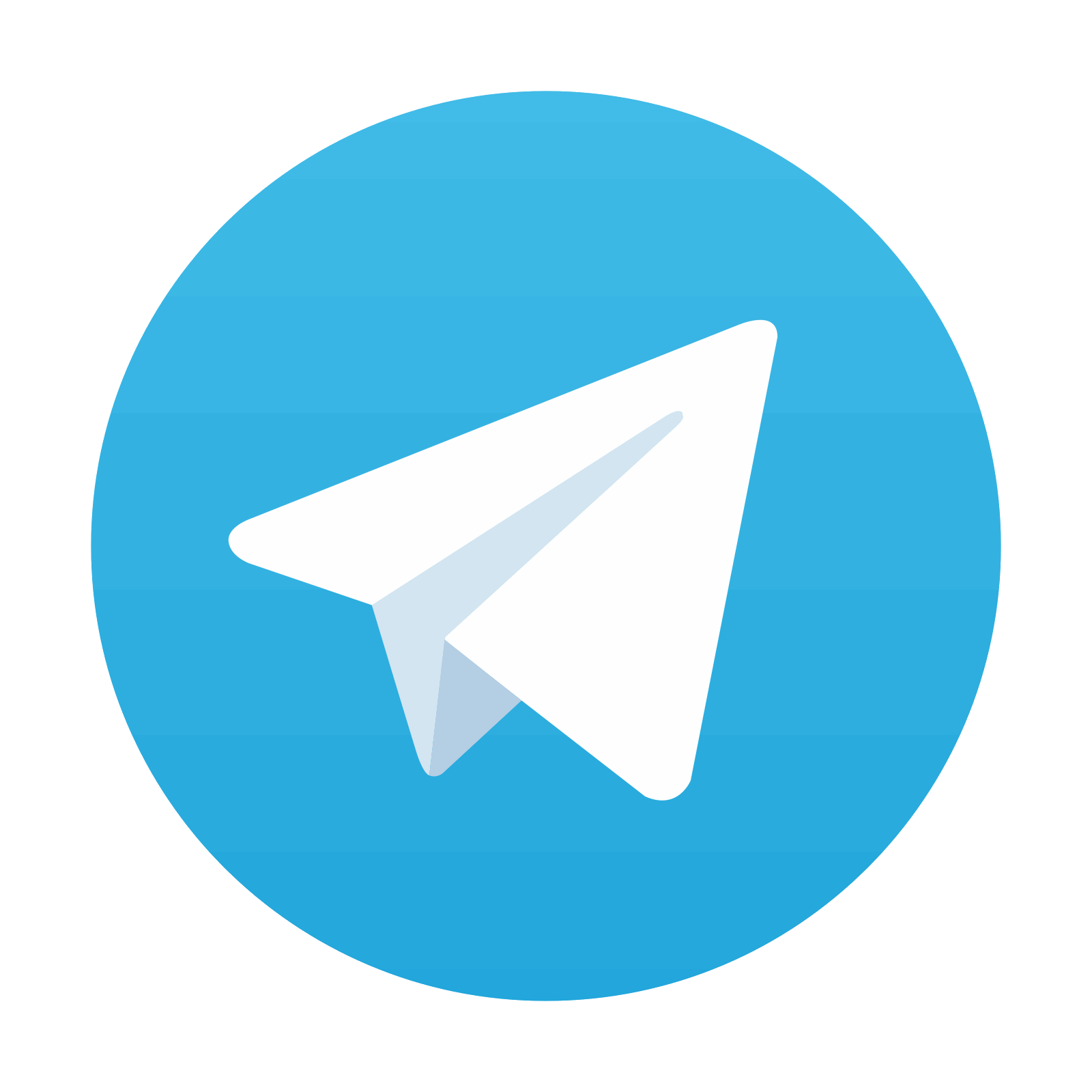