(1)
Neurology, Division of Pediatric and Developmental Neurology, Washington University School of Medicine and St. Louis Children’s Hospital, 660 South Euclid Ave, Campus Box 8111, St. Louis, MO 63110, USA
Keywords
Electrographic seizuresIctal–interictal continuumBrief rhythmic dischargesGamma-aminobutyric acid (GABA) N-methyl-D-aspartate (NMDA)Alpha-amino-3-hydroxy-5-methyl-4-isoxazolepropionic acid (AMPA)Introduction
Seizures are a common feature of neurologic illness in the neonatal period, the first 28 days of life. Neonatal seizures and status epilepticus may occur due to a range of etiologies, including structural injuries, genetic abnormalities, inborn errors of metabolism, and treatable systemic conditions, such as hypoglycemia, among others. In this chapter, we outline unique features of neonatal status epilepticus, including developmental differences in the gamma-aminobutyric acid (GABA) system, as well as the clinical and electrographic presentation, treatment, and outcomes.
Definition
Defining neonatal status epilepticus (SE) remains difficult. Many infants have prolonged seizures on presentation and frequently present with repetitive seizures. Determining whether there is return to baseline, a key component of the SE definition in children and adults, is complicated by differences in age-related responsiveness and by prematurity, critical illness, and encephalopathy.
Neonatal seizures have been defined as having a distinct beginning, evolution of at least 2 microvolts, and a duration of at least 10 s [1, 2]. Defining this beginning, evolution, and end is critical because many neonatal seizures are subclinical or have only subtle clinical features. Distinguishing these features from other types of short, nonevolving rhythmic discharges may prevent overtreatment [3]. The definition of neonatal SE has pitfalls. Typical definitions of SE rely on clinical factors, such as the duration of seizures, typically 30 min, or lack of return to baseline in between seizures [4, 5]. This definition is difficult to apply in neonates, as reliable electroencephalography (EEG) criteria are not readily available, and the majority of neonatal seizures are subclinical or subtle. Additionally, determining the neurologic baseline of an encephalopathic newborn often poses challenges.
Therefore, although neonatal seizures can be described with similar terminology as used for seizures in children and adults [4], neonatal SE may require an additional definition. Consequently, several studies refer to a measurement of an absolute seizure burden during a recording [2, 6, 7]. Seizure burden has been defined as the percentage of an epoch, typically an hour, during which there is electrographic seizure activity [6, 8]. This definition, however, does not correlate well with the assessment of seizures per hour (frequency) or of total seizure duration (summing the length of seizures over a particular epoch). To resolve this, calculating an “ictal fraction” by summing the overall duration of seizures in a period of time divided by the total recording time may provide a better picture of the individual seizure burden [9, 10]. The ictal fraction, however, will decrease with longer recording time, so neonatal SE should be defined as either an ongoing seizure for 30 min or total seizure time of 50% of an hour’s recording [11, 12].
Epidemiology
Given the challenges of defining neonatal SE, it is, by extension, also difficult to assess incidence and prevalence. It is estimated that neonatal seizures occur in 1–5 out of 1000 live births, and in up to 5% of low birth weight infants [13–16]. The most common etiologies include hypoxic-ischemic injury, stroke, infections, and inherited structural causes [3, 14, 15, 17]. Less common but important causes of seizures and SE are metabolic conditions, such as hypoglycemia or inborn errors of metabolism [18, 19]. Finally, the highest proportion of patients with SE is likely to be those with neonatal epileptic encephalopathies, the most well-recognized being early infantile epileptic encephalopathy (EIEE, or Ohtahara’s syndrome) and early myoclonic epilepsy (EME) [20].
Developmental Susceptibility
The relatively high incidence of seizures in the neonatal period may be related to an inherent predisposition to generating seizures. Normal neurobiologic processes of synaptogenesis and brain development that occur in the perinatal period require strong glutamatergic signaling. Additionally, there is also a propensity for gamma-aminobutyric acid (GABA)-mediated inhibitory circuitry to have a net excitatory effect on the developing brain in utero through at least the final trimester, thereby increasing the neonatal brain’s susceptibility to seizures [21, 22].
In a fully developed brain, neuronal stress or injury leads to rapid depolarization of the presynaptic cell membrane, an influx of calcium ions, and release of the excitatory neurotransmitter glutamate. Glutamate then crosses the synapse to act on its receptors, specifically the N-methyl-D-aspartate (NMDA) and alpha-amino-3-hydroxy-5-methyl-4-isoxazolepropionic acid (AMPA) receptors. In the neonatal period, there is a greater number of these receptors due to the importance of glutamate in normal neuronal maturation [23].
Both the NMDA and AMPA receptors bear developmental signatures that lead to an imbalance of excitation and inhibition. Neonatal NMDA receptors are even more excitable than in the older brain, which is crucial for the development of the hippocampus and neocortex. In the neonate, NMDA receptors have increased glutamate binding and glycine activation—necessary for channel activation by glutamate. Additionally, there are changes in the inhibitory modulation of the NMDA receptor that leave it with a higher propensity for activation [12, 24, 25]. There are also changes in subunit expression, specifically over-expression of the NR2B subunit of the NMDA receptor [26–28]. The NR2B-containing neurons are uniquely positioned to cause cell injury and subsequent excitation based on their association with prolonged calcium influx [29–31]. Similarly, changes in the AMPA receptor lead to increased excitation [32]. In mature neurons, the GluR2 subunit decreases calcium permeability, thereby decreasing excitotoxicity. This subunit is decreased in the immature brain, and is further decreased by hypoxic-ischemic injury [33–35].
In addition to differences in the physiology of excitatory synapses in the immature brain, there is lower GABA-mediated inhibitory tone through the third trimester, further increasing the propensity toward excitation. The expression of GABA receptors is decreased in the developing brain [36, 37], and its inherent properties, including receptor subunit expression and the direction of the chloride ion (Cl−) gradient, are different from that in mature neurons [38]. In the immature brain there is a relative over-expression of the α4 and α2 GABA subunits compared that of the α1 subunit [36, 37]. This pattern of subunit expression leads to resistance to benzodiazepines [36, 37] and has been shown to occur independently in SE [23, 36, 39]. Changes in subunit expression that predispose to seizures occur independently in both the immature brain and in SE and may thus be additive in the neonatal brain.
Furthermore, there are physiologic differences in the movement of Cl− across the postsynaptic cell membrane. In mature neurons, GABA and its agonists bind to the postsynaptic receptors and promote Cl− entry into the cell, thereby causing hyperpolarization and decreased cell firing. The opposite occurs in immature neurons, due to changes in the proteins that assist with ion transport across membranes. There is an over-expression in the sodium–potassium–chloride cotransporter 1 (NKCC1) and an under-expression of the potassium–chloride2 (KCC2) channel. NKCC1 pumps chloride ions into the cell, while KCC2 ion channels allow chloride to exit the neuron. Therefore, binding by GABA or other agonists to the GABA-A receptor on the postsynaptic cell prompts chloride ions to travel out of the cell, leading to membrane depolarization and increased excitation [40–42]. This difference between the developing and mature brain is currently under further investigation in clinical trials with bumetanide, an NKCC1 transporter inhibitor. Bumetanide blocks NKCC1, driving up the extracellular chloride ion concentration. Therefore, as GABA or a GABA agonist binds to the GABA-A receptor, chloride travels into and depolarizes the cell, and blocks epileptiform activity in hippocampal slices and seizures in neonatal rats [40].
Finally, this chloride gradient in GABA-A containing neurons matures in a caudal to rostral direction [43]. This maturation pattern may provide a neurophysiologic mechanism whereby electroclinical dissociation occurs, in which the clinical manifestation of seizures subsides with GABA agonist treatment, but the electrographic seizure persists. Subcortical GABA neurons may remain inhibitory during this period, leading to inhibition of subcortical motor innervation, while causing paradoxical excitation of cortical circuits [40].
Etiologies
The most common causes of status in a newborn are acquired neonatal brain injuries, including hypoxic-ischemic encephalopathy (HIE), infarction, and hemorrhage (Table 26.1) [14, 44]. Hypoxic-ischemic injury, typically caused by perinatal asphyxia, is the cause of seizures in 60–80% of infants [14, 38, 45]. When seizures occur in the setting of HIE, they often present within the first 12–24 h of life; most will be subtle seizures in the first 12 h, including SE [14]. Decreased oxygen delivery or blood flow, or both, lead to cell injury, placing these regions at risk for seizures. Additionally, the sodium–potassium–chloride cotransporter 1 (NKCC1), discussed above, is up-regulated in hypoxic-ischemic injury, providing another mechanism for seizure propensity in this population [46].
Table 26.1
Common etiologies of neonatal seizures, their relative frequency, and the prognosis for normal development in these conditions when they occur with seizures
Etiology | Timing of seizure (hours of life) | Frequency (%) | Normal development (%) |
---|---|---|---|
Hypoxic-ischemic encephalopathy (HIE) | 0–24 | 60 | 50 |
Cerebral infarction | 12–72 | 15 | a |
Intracranial hemorrhage – Term – Preterm | 24–48 >72 | 15 45 | 10 |
Infection | >72 | 5–10 | 50 |
Genetic/structural | Variable | 15 | 0 |
Hypoglycemia | 0–48 | 3 | 50 |
Hypocalcemia | 24–48 | Rare | 50–100 |
Focal cerebral infarction, or stroke, accounts for about 15% of seizures in neonates. In these patients, seizures often occur in the first 12–72 h of life [47, 48]. In contrast to encephalopathic infants with HIE, patients with focal stroke are otherwise well-appearing, without signs of encephalopathy.
Intracranial hemorrhages, including subarachnoid (SAH) and subdural hemorrhage (SDH), are less common causes of seizures and SE, but intraventricular hemorrhage (IVH) can be seen in up to 45% of preterm infants with seizures [47]. SAH is extremely common in term newborns and rarely causes seizures, but when it occurs it is usually at 24–48 h of life [49]. SDH is also a rare cause of seizures due to trauma. These are typically focal seizures occurring in the first 48 h of life [50].
Intracranial infection accounts for 5–10% of cases of neonatal seizures [14]. Common bacterial pathogens include Group B streptococci and E. Coli, with seizures usually occurring after day 5 of life [14, 51]. The most common nonbacterial pathogens include TORCH infections: Toxoplasmosis, Rubella, Cytomegalovirus (CMV), and Herpes simplex virus (HSV).
Another important cause of neonatal seizures and potentially, SE, is transient metabolic abnormalities. They may occur in isolation or more frequently, accompany other diseases. The three leading causes of these metabolic derangements are hypoglycemia, hypocalcemia, and hypomagnesemia. Hypoglycemia is the most common of these metabolic derangements, typically occurring in infants of mothers with gestational diabetes. These infants are small-for-age and, depending on the duration of in utero hypoglycemia, may have a variety of neurologic symptoms, including decreased mental status, apnea, and jitteriness, with seizures occurring in approximately 50% of patients, often around the second day of life [14, 52]. As with many of these conditions, hypo- or hyperglycemia may contribute to poor outcomes in the setting of underlying brain pathology, such as hypoxic-ischemic injury [53].
Hypocalcemia occurs more frequently in low birth weight infants in the first 2–3 days of life, but it may also be seen in normal-weight infants in the first 24 h. Calcium ions play a critical role in neuronal firing, in both pre- and postsynaptic cells. Most commonly, hypocalcemia occurs due to other conditions, including hypoglycemia or HIE [54, 55]. Similarly, hypomagnesemia occurs frequently in the setting of hypocalcemia but may occur independently. Hypomagnesemia produces a characteristic syndrome of hyperreflexia, jitteriness, and seizures [54, 56].
Less common causes of seizures in the newborn period include anesthetic intoxication due to local anesthetic administration during labor, and hyper- or hyponatremia related to a structural etiology, at times presenting with the syndrome of inappropriate antidiuretic hormone (SIADH), or due to iatrogenic hypernatremia [14].
Beyond transient metabolic abnormalities, inborn errors of metabolism and genetic and structural abnormalities may also lead to neonatal SE. These are frequently accompanied by neonatal epileptic encephalopathies. The two most well-recognized phenotypes are early myoclonic encephalopathy (EME) and early infantile epileptic encephalopathy (EIEE) or Ohtahara syndrome. These two syndromes, often caused by underlying genetic or metabolic conditions, are characterized by frequent clonic, myoclonic, and tonic seizures. The EEG is characterized by a “suppression–burst” pattern, where the suppression is more prominent than the bursts (Fig. 26.1). This pattern worsens during sleep in EME but is unchanged in EIEE. The suppression–burst pattern often persists in EME but typically transitions to hypsarrhythmia in EIEE [57, 58]. The phenotypes of these two syndromes are relatively similar. Traditionally, the etiologies have been considered diverse, with metabolic causes leading to EME and structural causes to EIEE. As more metabolic and genetic abnormalities become recognized, however, the phenotype–genotype correlation is becoming less clear, and these two syndromes may exist on a spectrum, rather than as isolated diseases.


Fig. 26.1
Suppression–burst pattern of a neonatal epileptic encephalopathy. This patient had a clinical presentation and EEG on the spectrum between early myoclonic epilepsy (EME) and early infantile epileptic encephalopathy (EIEE) and was found to have a pyridox(am)ine oxidase (PNPO) mutation
One of the best characterized causes of neonatal epileptic encephalopathy is non-ketotic hyperglycinemia or glycine encephalopathy. This condition is due to an underlying defect of the glycine cleavage system, an enzyme complex consisting of four separate proteins. Defects in one of these proteins, most commonly the P- or T-proteins, lead to accumulation of glycine in the central nervous system (CNS). Excess glycine acts as both an excitatory and inhibitory transmitter in the CNS. It acts as an excitatory transmitter via NMDA receptors, leading to excess calcium entry into the cell and subsequent neuronal injury. The inhibitory glycine receptors are located in the brainstem and spinal cord and may contribute to the constellation of apnea, hypotonia, and in utero hiccupping that occurs in this condition. When glycine encephalopathy presents in the newborn period it is severe, with refractory myoclonic seizures, hypotonia, apnea, and encephalopathy [59]. Outcomes are poor, with a high mortality rate in the newborn period and profound neurodevelopmental delays in survivors. Treatments with sodium benzoate, which binds excess glycine, and with dextromethorphan to block NMDA receptors have been tried as treatments, with some improvement in outcomes [60].
Another inborn error of metabolism that is important to recognize given its distinct treatment is the spectrum of pyridoxine and pyridoxal-5-phosphate (P5P or PLP)-dependent epilepsy. Pyridoxine (or vitamin B6)-dependent epilepsy may cause SE in the newborn period. There are two known causes of pyridoxine-related epilepsy. The first, more common, type is related to a defect in antiquitin, an enzyme in the lysine degradation pathway that leads to build up of a toxic metabolite, alpha-aminoadipic-semialdehyde (AASA). AASA sequesters P5P, pyridoxine’s metabolically active form, thereby limiting its availability as a cofactor in numerous reactions, including the synthesis of the inhibitory neurotransmitter GABA [61, 62].
The second, more recently discovered, pyridoxine-related epilepsy is due to a deficit in the enzyme pyridox(am)ine oxidase (PNPO) that converts pyridoxine to the metabolically active P5P. Defects in the quantity and functional configuration of PNPO have been identified in patients with early epileptic encephalopathies [63–65].
Another overlapping, rare but treatable cause of neonatal seizures is folinic acid-responsive epilepsy. This condition may present within the first several hours of life and responds to oral treatment with folinic acid [66]. Folinic-responsive seizures may be identical to pyridoxine-dependent seizures, given similar metabolites found on high pressure liquid chromatography [67]. Of note, though, case reports of other conditions, including cerebral folate deficiency and STXBP1 mutation, are also responsive to folinic acid, implying that the field is still evolving [68, 69].
Disorders of glucose transport 1 (Glut1), encoded by the gene SLC2A1 (Solute Carrier Family 2 Member 1) are also becoming recognized as causes of seizures at all ages of childhood. Nearly 70% of patients present before 6 months of age and a quarter of these in the first 2 months of life. This condition is characterized by a low CSF glucose concentration compared with serum levels [70, 71]. The most effective treatment is the ketogenic diet [72]. See Table 26.2 [18, 73] for a summary of inborn errors of metabolism.
Inherited metabolic epilepsies | Lab tests | Gene(s) involved | Treatment |
---|---|---|---|
Amino acidurias | Amino acids (serum) | Varied | Amino acid dependent |
Organic acidurias | Organic acids (urine) | Varied | Organic acid dependent |
Fatty acid oxidation defects | Lactate, pyruvate | Varied | Symptomatic |
Mitochondrial disorders | Lactate, pyruvate, serum amino acids, urine organic acids, acylcarnitines | Varied | Cofactors and supplements |
Urea cycle defects | Ammonia, acylcarnitines | Varied | Ammonia scavengers |
Biotinidase deficiency | Ammonia, biotinidase activity | BTD | Biotin |
Cerebral folate deficiency | CSF methyltetrahydrofolate | Folate receptor protein 1 (FR1) | Folinic acid |
Disorders of creatine synthesis | Urine guanidinoacetate and MRS | GAMT, GATM, and SLC6A8 | Disorder dependent |
Glucose transporter deficiency (Glut1) | CSF: serum glucose ratio | SLC2A1 | Ketogenic diet |
Glycine encephalopathy | Glycine (serum and CSF) | Glycine cleavage proteins | Sodium benzoate, dextromethorphan |
Menkes disease | Serum copper and ceruloplasmin | ATP7A | Copper injections |
Molybdenum cofactor deficiency/sulfite oxidase deficiency | Urine sulfites and S-sulfocysteine | MOCS1 or 2, SUOX | Symptomatic |
Pyridoxine-dependent epilepsy | AASA, pipecolic acid (serum) | ALDH7A1 | Pyridoxine |
PLP-dependent epilepsy | CSF PLP | PNPO | PLP |
Pyruvate dehydrogenase deficiency | Lactate, pyruvate | PDH | Ketogenic diet |
Serine deficiency (PHGDH deficiency) | CSF amino acids | PHGDH | L-serine |
Succinic semialdehyde dehydrogenase deficiency (SSADH) | 4-hydroxybutyric acid (urine, serum, CSF) | ALDH5A1 | Symptomatic |
Zellweger syndrome | Peroxisomal enzyme or very long chain fatty acid panel | PEX genes | Symptomatic |
Finally, inherited structural etiologies may be the cause of neonatal seizures in 3–17% of patients [74]. These seizures and SE episodes may be refractory and include etiologies such as hemimegalencephaly, focal cortical dysplasia, pachygyria, polymicrogyria, and lissencephaly. Many of these structural causes of epileptic encephalopathies have been linked to genetic mutations [75]. Of particular interest, a link to the mammalian target of rapamycin (mTOR) pathway, which is involved in cell size and proliferation, has received increasing interest as a target of therapeutic interventions [76–78].
Clinical Semiology
Neonatal seizures tend to be focal, as opposed to generalized, and often have very subtle clinical findings [14, 79]. Classically, neonatal seizures have been identified based on clinical characteristics and criteria. Mizrahi and Kellaway, however, highlighted the notion that many of these movements and behaviors labeled as seizures did not have an EEG correlate [80]. Their study classified 425 clinical seizures in 71 infants and found that focal clonic, multifocal clonic, hemiclonic, and particular myoclonic and tonic seizures had a one-to-one correlation with epileptiform discharges on EEG, while more subtle oral-buccal movements, tremor, generalized tonic seizures, and other particular myoclonic seizures did not have a consistent EEG correlate [80].
Neonatal seizures and SE also have a high prevalence of subtle and electrographic-only seizures [47, 79, 81, 82]. Electrographic seizures occur in up to 90% of neonates who have seizures identified on clinically ordered video-EEG monitoring. Of those 90% with seizures, 12–79% have electrographic-only, or subclinical, seizures [1, 47, 83]. This high prevalence of electrographic-only seizures in neonates indicates that a large proportion of seizures may present without clinically recognizable signs.
Neonatal seizures can therefore be classified as subtle, clonic, tonic, or myoclonic [14], plus subclinical (or electrographic-only) seizures. Subtle seizures consist of a number of behaviors, movements, or phenomena that are not deemed clearly clonic, tonic, or myoclonic. For example, oral automatisms, eye deviation or fixation, and autonomic changes or apnea are all considered subtle seizures [14]. These subtle seizures may account for up to 68% of all preterm and up to 75% of term neonatal seizures [47]. Apnea is rarely a seizure on its own but may occur in conjunction with other subtle clinical phenomena [84, 85].
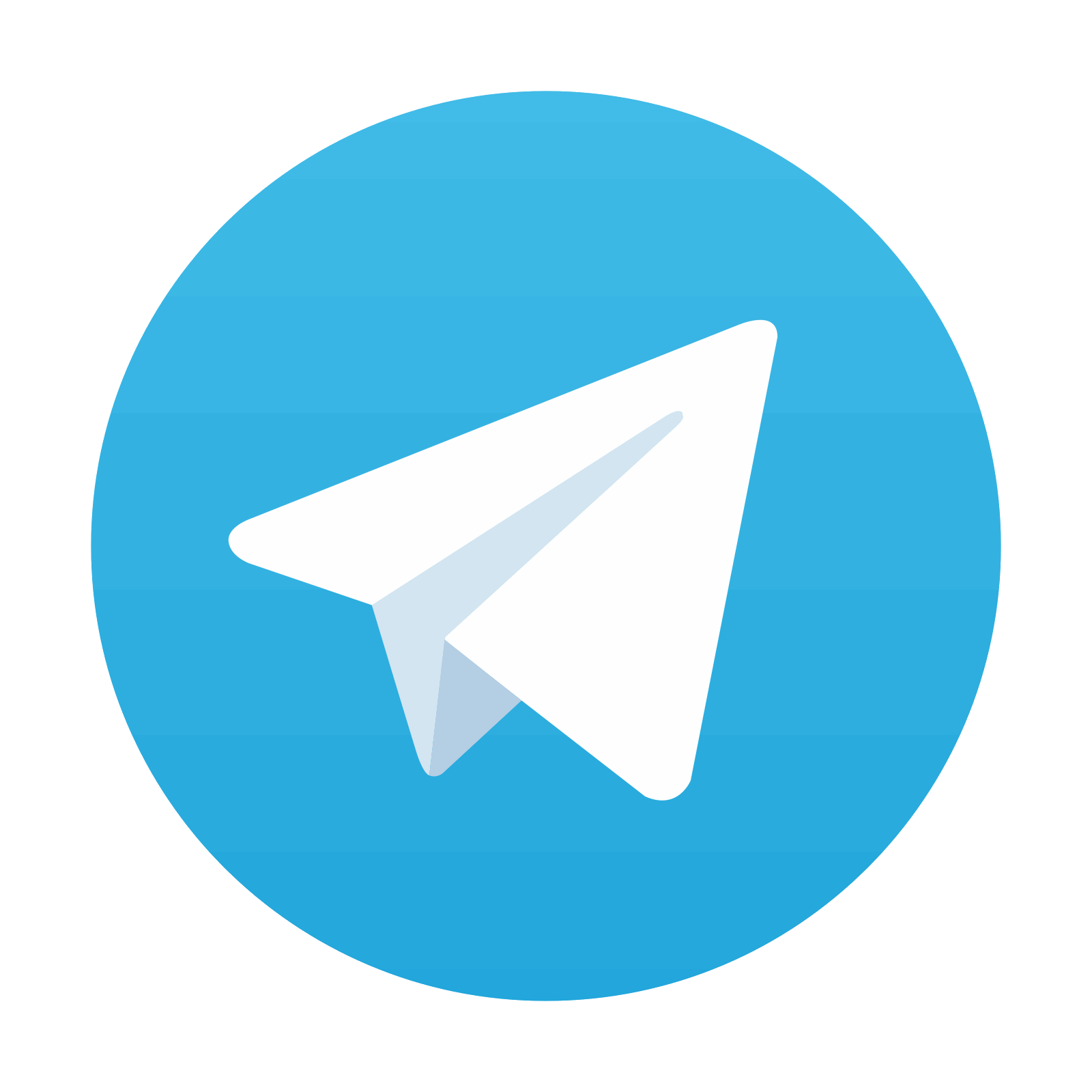
Stay updated, free articles. Join our Telegram channel

Full access? Get Clinical Tree
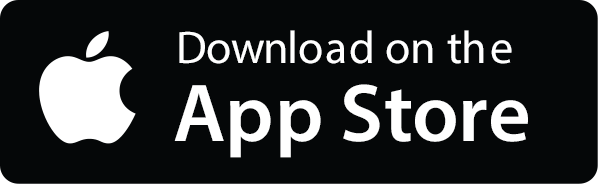
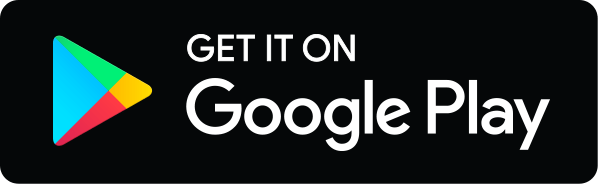