One of the major advances in the treatment of thoracic spine tumors has been the development of high-dose conformal photon radiation therapy. 1 Improvements in imaging technology and computerized treatment planning have allowed the safe delivery of high-dose radiation (image-guided intensity-modulated radiation therapy, or spinal radiosurgery) to spinal thoracic tumors, even in close proximity to the spinal cord and other paraspinal dose sensitive organs. This stereotactic radiosurgery (SRS) treatment can be given in a single fraction of high-dose radiation that is able to limit the dose to the spinal cord and surrounding normal structures to safe dose levels. The greatest utility of SRS is the ability to treat tumors that are traditionally considered resistant to conventional external beam radiotherapy (cEBRT) with ablative doses. 2 SRS represents a major advance in the treatment of thoracic benign and malignant spine tumors in terms of both local tumor control and pain control. Open surgery can be avoided completely in some cases. Additional advantages include shorter treatment times and less soft tissue toxicity than seen with cEBRT. The primary indications for spine SRS include as a primary treatment for tumors, re-irradiation following failed prior cEBRT, as a postoperative adjuvant, or neoadjuvant therapy for some tumors. Most importantly, the safety of radiosurgery is well documented, even in the setting of prior spinal cord irradiation. 3, 4, 5, 6 While surgical extirpation is currently felt to be the best initial treatment option for most benign tumors of the thoracic, spine SRS has been demonstrated to have long-term clinical and radiographic benefit for the treatment of such lesions, including 10 year follow-up experience. 7, 8 In providing palliation for spinal tumors, especially radioresistant tumors, SRS is very effective either as initial therapy or after failure of cEBRT. Numerous studies have reported excellent local control (85 to 98%), rapid durable symptom relief (pain relief of 65 to 90% with a median time to pain relief of 2 weeks), and minimal toxicity. 9, 10, 11, 12, 13, 14, 15, 16 A substantial body of data supports a number of benefits of spine SRS over cEBRT, including several evidence-based reviews. 17 The current indications for spine SRS can be grouped into three general categories: (1) primary definitive therapy for previously unirradiated tumors, (2) salvage SRS for recurrent or progressive tumors having failed prior cEBRT, and (3) postoperative SRS after open surgical intervention with or without spinal stabilization (see ▶ text box). 18 Compared with primary cEBRT, the benefits of primary SRS include a shorter treatment time, which minimizes the potential for interruptions in systemic therapy; delivery of a high radiobiological dose, which may overcome the relative radioresistance that challenged cEBRT in certain histologies (e.g., melanoma, renal cell carcinoma); and improved tumor control and symptom relief. Tumor control rates as high as 100% have been published using SRS for previously unirradiated spine metastases. 19 Moreover, long-term radiographic tumor control has been demonstrated to be independent of primary histology, with 90% long-term tumor control. 11 Patient selection for primary SRS continues to evolve. A recursive partitioning analysis stratifying patients into a three-class system, based on time from primary diagnosis (> 30 months) and performance status (Karnofsky Performance Status > 70), has been developed and serves as a guide for selecting patients with longer overall survival more likely to benefit from the improved tumor and symptom control associated with a primary radiosurgical approach. 20 Safe dose escalation leads not only to higher rates of pain control than historical controls for cEBRT but also more durable pain control with a median duration of pain control of greater than 1 year. 21 Quality of life improves as a result of improved pain control. 9, 19 The more effective local treatment for thoracic spine metastases may translate into longer survival for select patients with oligometastases, similar to that seen for brain metastases. 22 Indications for Radiosurgery to Thoracic Tumors Primary definitive therapy Salvage therapy for recurrent or progressive tumors after prior radiotherapy Postoperative therapy after open surgical intervention For medically inoperable patients As an adjunct to surgery For lesions not amenable to open surgery All patients with thoracic spine tumors are treated in a supine position. At our institution, patients are immobilized with the BodyFix (total body bag, Medical Intelligence) when treatment sites are below T6; otherwise, a head and shoulder mask with S-board (CIVCO, Kalona, Iowa) is used ( ▶ Fig. 40.1). Perioperative steroids may be administered for cases in which there is a concern that post-SRS tumor swelling might cause symptomatic compression of neural elements. For lower thoracic spine SRS cases, antiemetics are routinely given the day of treatment to avoid symptoms of postoperative nausea that may result from radiation falloff to the stomach. Fig. 40.1 Patients are positioned on the treatment couch in a supine position and immobilized. A molded facemask is used in cases of upper thoracic targets, and a body bag is used for lower thoracic targets. The spine SRS procedure involves accurate identification of the target and surrounding normal tissues, radiosurgery treatment planning, reliable immobilization, image-guided verification, and targeted delivery with submillimeter accuracy. There are important similarities to open surgery. Careful target delineation and tumor contouring are essential for successful SRS treatment. SRS requires precise identification of the tumor as well as normal structures, particularly the spinal cord ( ▶ Fig. 40.2). Failure to contour any part of the tumor or spinal cord may result in tumor progression or spinal cord injury, respectively. Fig. 40.2 Case example of a T11–12 breast cancer metastasis. Sagittal (a) and axial (b) magnetic resonance imaging is used to better delineate the normal and abnormal anatomy, especially the spinal cord. This image is fused with a computed tomographic image for target contouring and treatment planning. Tumors are optimally visualized with magnetic resonance imaging (MRI), but treatment simulation is currently based on computed tomography (CT). Modern commercial radiosurgery systems use CT-based imaging for planning and delivery. A number of systems have MRI and CT fusion algorithms to better identify the targets and critical structures. Most systems today also permit image fusion between MRI and CT images. For some cases, 10 F-fluorodeoxyglucose positron emission tomography may be used to more precisely identify the tumor target volume ( ▶ Fig. 40.3). Such image fusion may improve the target definition for spinal tumors, especially when such neoplasms exhibit heterogeneous contrast enhancement. For cases in which spinal instrumentation has been implanted, postmyelography CT is useful to better delineate the spinal cord. Fig. 40.3 Positron emission tomography and computed tomographic imaging may also be used for better delineation of the tumor within the vertebral body. In the spine, MRI-CT image fusion is usually more challenging than that required for intracranial radiosurgery because it is more dependent on the technical aspects of image acquisition and patient positioning during image acquisition because of flexibility of the spine. The quality of MRI spinal image fusion often requires that the patient’s imaging position closely match the intended treatment position. Issues of spatial distortion need to be considered if MRI is used directly for planning. Because signal intensities of MRIs do not reflect a direct relationship with electron densities unless attenuation coefficients are manually assigned to the region of interest, spatial distortion limits the accuracy of using MRI directly in radiosurgery planning. The ability to identify tumors on CT, together with the probability of generating an adequate image fusion with MRI, is the key to defining the radiosurgery target. The International Commission on Radiation Units and Measurements has formalized the contouring process by defining three different volumes: the gross tumor volume (GTV) represents the unambiguous radiologic confines of the target neoplasm, the clinical treatment volume (CTV) includes nearby anatomic regions such as the vertebral body where microscopic tumor extension is anticipated, and the planned treatment volume (PTV) is an augmented form of the CTV that corrects for movement and accuracy of treatment delivery. For tumor delineation, the GTV is contoured to precisely identify the tumor that is visualized on MRI and CT imaging ( ▶ Fig. 40.4). The best images to identify bone tumors are the T1-weighted and T2-short tau inversion recovery. Tumor has variable intensity on T2-weighted images, which is not as useful for tumor delineation; however, T2-weighted axial images provide the best assessment of the spinal cord to assess spinal canal impingement or spinal cord compression and to keep track of dose to the spinal cord. From the GTV contour, a CTV is drawn to account for microscopic disease outside the defined GTV. As opposed to brain metastases, vertebral body tumors are thought to have an infiltrative penumbra throughout the marrow space of the entire vertebrae. If the GTV involves a small portion of the vertebral body, the CTV will be defined as the entire vertebral body. Contouring the entire vertebral body may reduce the risk of recurrence and improve symptom relief/recurrence over partial vertebral body coverage. Fig. 40.4 Axial (a), sagittal (b), and coronal (c) images of the contours of the target gross tumor volume (red line) and 2-mm expansion planned tumor volume (green line). The PET avid portion is receiving a large boost of radiation. Notice the rapid falloff of radiation dose (yellow line) to the surrounding normal structures such as the lungs, aorta, and spinal cord.
40.2 Patient Selection
40.3 Preoperative Preparation
40.4 Operative Procedure
40.4.1 Target Delineation
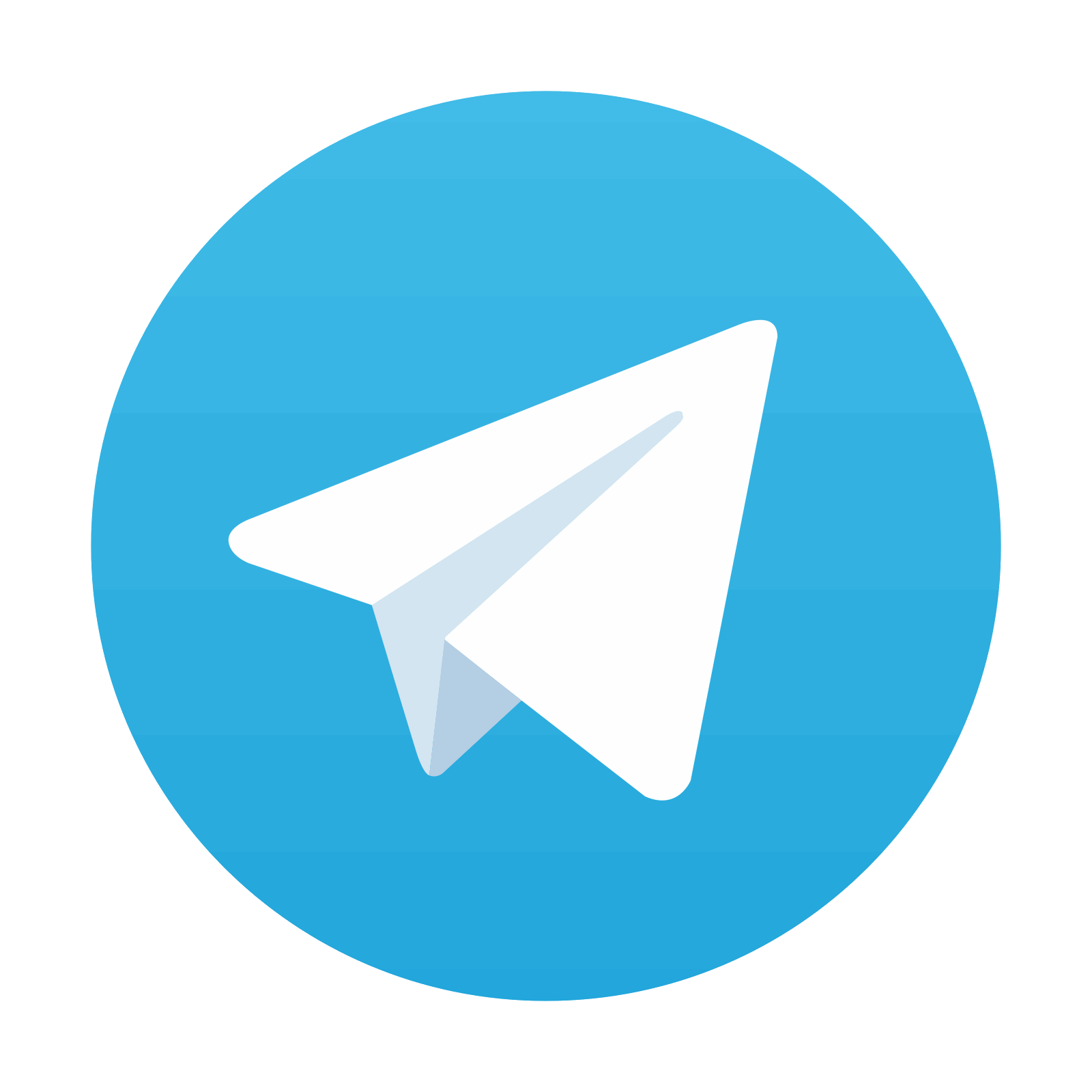
Stay updated, free articles. Join our Telegram channel

Full access? Get Clinical Tree
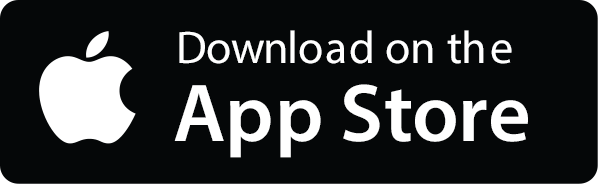
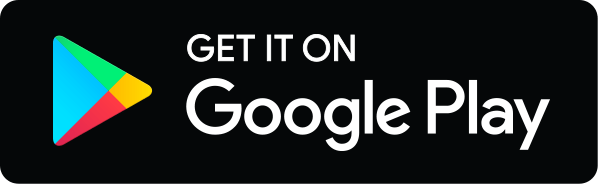