Cranial arteriovenous malformations (AVM) and cranial dural arteriovenous fistulas (AVF) carry a significant risk of morbidity and mortality when they hemorrhage. Current treatment options include surgery, embolization, radiosurgery, or a combination of these treatments. Radiosurgery is thought to reduce the risk hemorrhage in AVMs and AVFs by obliterating of the nidus of abnormal vasculature over the course of 2 to 3 years. Success in treating AVMs is variable depending on the volume of the lesion, the radiation dose, and the pattern of vascular supply and drainage. This article discusses the considerations for selecting radiosurgery as a treatment modality in patients who present with AVMs and AVFs.
Cranial arteriovenous malformations (AVM) are collections and anastomoses of arterial and venous vasculature. These are nonneoplastic and are distinguished from hemangiomas, which have increased endothelial cell turnover. In some cases, they may demonstrate slow growth, but this is caused by hypertrophy and not increased cell cycle. Left untreated, 3% to 5% of AVMs bleed each year, with higher rates of bleeding in AVMs that have previously ruptured or contain intranidal aneurysms. High flow rate and venous outflow stenosis have also been proposed to increase the risk of hemorrhage, but these variables have not been reported because they are difficult to measure. In AVMs with no history of bleeding, the annual risk is slightly lower at 2%, but after the first bleed, the annual risk of bleed is nearly 18%. A total of 1% to 1.5% of AVM patients die each year, most commonly because of hemorrhage. Although hemorrhage contributes to significant morbidity and mortality in AVM patients, about 1% also develop new seizures each year. Management of AVMs is a delicate balance of the morbidity and mortality of treatment versus the symptoms of the patient, risk of recurrent hemorrhages, seizure risk, and quality of life.
Dural arteriovenous fistulas (AVFs) are abnormal connections between meningeal arteries and a dural venous sinus, or subarachnoid veins. AVFs have a variable natural history, and not all cases may require intervention. The natural history of AVFs is predicted by the Borden classification scale, which describes AVFs based primarily on the draining veins and the direction of flow ( Table 1 ). Risks for hemorrhage can vary from 2% (Borden Grade I) to 19.2% (Borden Grade II or III). Furthermore, higher-grade AVFs have increased morbidity and mortality. For example, Borden Grade II and III AVFs have a 10.9% annual neurologic deficit incidence, and a 19.3% annual mortality rate. In addition, AVFs that present with cortical venous drainage are thought to have an increased chance of hemorrhage, and are typically treated regardless of clinical presentation, but this opinion continues to evolve. In a similar manner to AVMs, the risks of treatment and the natural history of AVFs must be considered in developing a treatment plan.
Type | Arterial Supply | Venous Drainage | Direction of Flow |
---|---|---|---|
Type I | Meningeal artery | Dural venous sinus or meningeal vein | Anterograde |
Type II | Meningeal artery | Venous sinus | Retrograde flow into subarachnoid veins |
Type III | Meningeal artery | Subarachnoid veins or isolated segment of dural venous sinus draining into subarachnoid veins | Retrograde flow into subarachnoid veins |
Stereotactic radiosurgery
Stereotactic radiosurgery (SRS) is a technique in radiosurgery that delivers a conformal dose of intense radiation on a target, such as an AVM. A number of different technologies have been developed to achieve this result: Gamma Knife; linear accelerators (LINACs; e.g., CyberKnife, Synergy S, and Trilogy); and proton-beam. Gamma Knife uses a radioactive source (cobalt) and aims radiation through a circular array of ports to focus the beams at a single point. LINACs, which are made by a number of different companies with different designs and implementations, do not have a radioactive source but rather accelerate electrons, which either directly interact with the target tissue or produce an X-ray beam directed at the tissue. Proton-beam therapy uses a synchrotron to accelerate protons to interact with the target tissue. Proton-beam therapy is often distinguished from the Gamma Knife and LINAC by the use of protons, which have more mass. As a result, the steep radiation dose fall-off allows for treatment of larger lesions and use of higher doses to minimize damage to neighboring tissue.
By definition, in addition to the radiation source, SRS involves a navigation system that allows the radiation to be delivered accurately and precisely. The original solution developed for the Gamma Knife was a stereotactic frame that was fixed to the skull, providing a set of reference coordinate axes. Other SRS systems, such as the CyberKnife, use frameless guidance involving a thermoplastic mask to minimize movement; built-in imaging systems update the position of the patient in real-time to improve the accuracy.
Mechanism and techniques of radiosurgery for AVM s and AVF s
Radiosurgery works to obliterate the AVM through two stages. Initially, radiosurgery damages the endothelial cells. The intimal layer thickens because of smooth muscle proliferation and deposits of hyaline, calcium, and collagen in the extracellular matrix. Ultimately, radiosurgery effects thrombosis and necrosis of AVM vessels resulting in a durable response that lasts at least a decade after radiosurgery. It is important to note that this process can take years to work. The exact mechanism of action for obliteration of AVFs is unknown; however, given the observed time course of obliteration, we believe that the mechanism of endothelial cell damage and thrombosis are likely applicable in AVFs.
Radiosurgery requires a median of 20 months to achieve subtotal (>95%) obliteration in AVMs. As a result, radiosurgery is not typically used in AVMs that present with hemorrhage. There is a significantly increased incidence of repeat hemorrhage (18% per 12 months) in these patients, so the immediate effect of microsurgery is a significant benefit compared with radiosurgery.
The important technical point for treating AVMs and AVFs is localization. In appropriately selected patients, radiosurgery obliteration depends on accurate targeting of the nidus or the fistula. Although digital subtraction angiography (DSA) serves as the gold standard for detection of AVM and AVF before and after radiosurgery, the two-dimensional study alone cannot be used for treatment planning. Therefore, thin-slice magnetic resonance imaging (MRI) and computed tomography (CT) are used to determine nidus geometry and neighboring at-risk structures for generating radiosurgery treatment plans. However, even MRI and CT have an average of 1-mm difference in stereotactic coordinates.
Technologic advancements in MRI and CT may eventually obviate the need for confirmatory DSA, but they are still insufficiently sensitive. As an example, patients can be followed with MRI or CT while an AVM nidus is visible, but a negative MRI or CT is followed by DSA to confirm AVM obliteration. Concurrently, advancements in DSA techniques offer the possibility of three-dimensional information needed for radiosurgery at the high resolution and sensitivity of DSA.
In terms of the actual machines, studies have suggested that the various radiosurgical systems are likely to be equivalent. One comparison of LINAC at 16 Gy the 70% isodose line with Gamma Knife at 20 Gy at the 50% isodose line found angiography-confirmed obliteration rates of 60% and 72%, respectively. Toxicity was observed in 8% of patients in both groups. The subsequent studies for the Gamma Knife and LINAC have also suggested no difference in efficacty. However, these studies are small and further comparative studies are necessary.
Mechanism and techniques of radiosurgery for AVM s and AVF s
Radiosurgery works to obliterate the AVM through two stages. Initially, radiosurgery damages the endothelial cells. The intimal layer thickens because of smooth muscle proliferation and deposits of hyaline, calcium, and collagen in the extracellular matrix. Ultimately, radiosurgery effects thrombosis and necrosis of AVM vessels resulting in a durable response that lasts at least a decade after radiosurgery. It is important to note that this process can take years to work. The exact mechanism of action for obliteration of AVFs is unknown; however, given the observed time course of obliteration, we believe that the mechanism of endothelial cell damage and thrombosis are likely applicable in AVFs.
Radiosurgery requires a median of 20 months to achieve subtotal (>95%) obliteration in AVMs. As a result, radiosurgery is not typically used in AVMs that present with hemorrhage. There is a significantly increased incidence of repeat hemorrhage (18% per 12 months) in these patients, so the immediate effect of microsurgery is a significant benefit compared with radiosurgery.
The important technical point for treating AVMs and AVFs is localization. In appropriately selected patients, radiosurgery obliteration depends on accurate targeting of the nidus or the fistula. Although digital subtraction angiography (DSA) serves as the gold standard for detection of AVM and AVF before and after radiosurgery, the two-dimensional study alone cannot be used for treatment planning. Therefore, thin-slice magnetic resonance imaging (MRI) and computed tomography (CT) are used to determine nidus geometry and neighboring at-risk structures for generating radiosurgery treatment plans. However, even MRI and CT have an average of 1-mm difference in stereotactic coordinates.
Technologic advancements in MRI and CT may eventually obviate the need for confirmatory DSA, but they are still insufficiently sensitive. As an example, patients can be followed with MRI or CT while an AVM nidus is visible, but a negative MRI or CT is followed by DSA to confirm AVM obliteration. Concurrently, advancements in DSA techniques offer the possibility of three-dimensional information needed for radiosurgery at the high resolution and sensitivity of DSA.
In terms of the actual machines, studies have suggested that the various radiosurgical systems are likely to be equivalent. One comparison of LINAC at 16 Gy the 70% isodose line with Gamma Knife at 20 Gy at the 50% isodose line found angiography-confirmed obliteration rates of 60% and 72%, respectively. Toxicity was observed in 8% of patients in both groups. The subsequent studies for the Gamma Knife and LINAC have also suggested no difference in efficacty. However, these studies are small and further comparative studies are necessary.
Choosing the appropriate AVM and AVF for radiosurgery
In determining the role of SRS for AVM patients, various scales are used to guide treatment. In unhemorrhaged AVMs, case selection can be guided by a number of AVM grading scales, such as the Spetzler-Martin scale (SM), modified Spetzler-Martin scale (mSM), and Pittsburgh radiosurgery-based AVM grading scale ( Table 2 ). Key components shared by these scales are AVM size and location. Although the SM scale was developed for predicting surgical morbidity and mortality, its role in guiding surgery has led to its use in guiding SRS. General recommendations are microsurgery for mSM Grades I to IIIA, whereas SRS or multimodal therapies are applied for mSM Grades IIIB to V. It is important to note that SM and mSM grading does not reflect the degree of protection from AVM hemorrhage, which is nearly 100%, but is dependent on extent of resection. Microsurgery remains the gold standard for AVM treatment; therefore, typical cases that are considered for SRS are SM Grade III or higher: greater than 3 cm involving eloquent cortex or deep drainage, or greater than 6 cm.
In SRS, successful obliteration of the AVM nidus has been found to depend on nidus volume, marginal dose, age, and gender, in addition to the SM grade. The Radiosurgery Based AVM Scale (RBAS) captures the effect of nidus volume, age, and location (hemisphere, corpus callosum, and cerebellum vs basal ganglia, thalamus, or brainstem). The location is closely related to the marginal dose, because the location determines the maximum dose deliverable to the nidus without clinically significant damage to neighboring structures. The RBAS score is calculated as follows: (0.1) (volume, mL) + (0.02) (age, yr) + (0.5) (location). Cases scoring less than 1 have 90% chance of AVM obliteration without a new neurologic deficit, whereas cases scoring greater than 2 have greater than 50% chance of such an outcome. For lesions greater than 15 cm 3 , nearly half have new neurologic deficits or incompletely obliterated AVMs when treated with SRS. In eloquent locations, such as the basal ganglia, brainstem, and the optic nerve, SRS can be challenging for lesions as small as 10 cm 3 .
Because the variables that predict radiosurgical success overlap with the criteria used for selecting microsurgery patients, some patients are not suitable candidates for either treatment alone. Giant AVMs (>25 cm 3 ) may require a combination of surgery, embolization, and SRS. Moderately sized lesions in challenging locations, such as the brainstem, may also necessitate combination therapy.
AVMs in pediatric patients present a distinct risk–benefit balance. Pediatric patients are exposed to a longer posttreatment at-risk period, increasing the cumulative lifetime risk. Therefore, successful obliteration and reduction of hemorrhage risk is very important. Many groups have resorted to a multidisciplinary treatment team delivering combination therapy.
Patients who present with a history of a prior bleed may not be good candidates for SRS. Studies have reported an increase in the relative risk of hemorrhage: the annual incidence of AVM hemorrhage is 2%, but the incidence of recurrent AVM hemorrhage is 18%. This is reflected in a 9.09 (confidence interval [CI], 5.44–15.19; P <.001) relative risk of recurrent hemorrhage. However, sometimes SRS can be an appropriate choice in patients who present with a prior hemorrhage if the risks of microsurgery or observation are also high.
In addressing AVFs, some AVFs have been reported to spontaneously resolve, particularly cavernous sinus AVFs. Therefore, some AVFs that present incidentally may not require intervention. In addition, up to 81% of patients receiving conservative treatment may have improvement or resolution of their symptoms. However, AVFs presenting with hemorrhage or severe neurologic deficit are likely to require treatment. Embolization or surgery, which both achieve immediate obliteration of the nidus, is the treatment of choice for cases with high risk of bleeding or with severe symptoms. Surgery can also be used as an adjunct to embolization for large AVFs that are incompletely obliterated by embolization or that are not amenable to embolization. Therefore, SRS monotherapy is most often used to treat small AVFs that are difficult to access surgically and present with significant but not severe symptoms. Larger lesions that are not amenable to surgery may also be responsive to combined embolization and SRS. Treatment of AVFs can be complicated by venous sinus thrombosis, which leads to increasing venous hypertension and edema after occlusive therapies, such as embolization or SRS. These patients can also be treated with SRS, but may require adjunctive therapies to restore cerebral drainage before SRS.
Factors impacting outcome of radiosurgery in AVM s and AVF s
To determine the factors that affect the outcome of SRS, one must acknowledge that there are varying opinions on the definition of successful treatment of an AVM or AVF. Success of SRS has been defined in terms of hemorrhage prevention and symptom management, or nidus obliteration. Typically, the most severe consequence of AVMs and AVFs is morbidity and mortality from hemorrhage, so seizure control is less well studied. However, only a subset of AVMs and AVFs hemorrhage each year, which limits statistical power. Therefore, many studies also define success as nidus or fistula obliteration. The likelihood of SRS success is associated with the patient’s clinical history, characteristics of the AVMs and AVFs, and the SRS technique.
History of embolization decreases the likelihood of SRS-induced AVM obliteration by an estimated 86%. This has not been shown to be a result of direct interaction between embolization therapy and SRS. Rather, embolization has an associated failure rate, either from inadequate penetration or from local recanalization; one study found this in 18.2% of embolizations. A nidus may develop where the prior embolization site is thought to be obliterated, and is therefore excluded from the radiation plan. Persistence of this nidus is referred to as an “out-of-field nidus.” Embolization may also create multiple segments of AVM, complicating SRS treatment design, but this has not been studied. This may be resolved by including the embolized region in the SRS plan. SRS has been shown to reduce local angiogenesis, which may decrease recanalization. However, this may not be feasible in cases where embolization is used to obliterate nidus that is near radiation-sensitive structures.
Characteristics of the AVM (size, venous drainage, and location) are associated with success of SRS. AVM size is often measured by maximum linear dimension or by nidus volume. A common threshold is maximum linear dimension of 3 and 6 cm, but studies have used numerous different thresholds for their statistical analysis. Increased AVM size is consistently associated with decreased obliteration rates or increased treatment-associated deficits. For example, AVMs greater than 10 cm 3 may have as much as a fivefold increase (7%–35.7%) in posttreatment hemorrhage rate. The number of draining veins and pattern of venous drainage are associated with microsurgical success, but are unclear factors for hemorrhage after SRS. One study observed that a single draining vein increases the relative risk of hemorrhage (relative risk, 1.66; P <.01), but another study found no difference in AVMs: 5 of 66 (7.6%) single draining vein AVMs hemorrhaged, compared with 8 (7%) of 115 in the overall population. Deep venous drainage was associated with double the odds of hemorrhage, but another study suggests that this may be confounded by an association with the location of the AVM. Proximity to eloquent structures is associated with decreased obliteration rates, but is not associated with hemorrhage rates. Lower nidus density, as defined on CT, is associated with a decreased obliteration rate (odds ratio, 0.246; P = .008), and as defined on MRI, is associated with an increased hemorrhage rate (relative risk, 1.64; P <.01). A history of AVM hemorrhage and prior treatment are not included in the common scoring systems, but are strongly associated with SRS success. RBAS and SM scales classify AVMs by characteristics of the patient and AVM at presentation.
Scales such as the RBAS and mSM may be used to help predict the success for SRS in AVMs because they incorporate the aforementioned clinical characteristics. RBAS and mSM scales are designed for ease of use and incorporate significant, clinically applicable predictive variables. The RBAS score is a simple method of determining the likelihood of obliteration without new neurologic deficits, but only considers the AVM size, patient age, and location. In the RBAS, the likelihood of obliteration without new neurologic deficit is 91.7% for less than 1, 74.1% for 1 to 1.5, 60% for 1.5 to 2, and 33.3% for greater than 2. Although designed for assessing microsurgery success, the mSM scale evaluates based on size (<3, 3–6, and >6 cm); location (eloquent or not); and venous drainage (superficial or deep). It approximately correlates with the RBAS score: 88.9% for Grade 1, 69.6% for Grade 2, 61.5% for Grade IIIB, and 44.8% for Grades IIIA and IV.
Finally, hemorrhage and nidus obliteration are also associated with radiation marginal dose, dose homogeneity, and fraction of nidus treated, but are not associated with the system used to deliver SRS. Increasing marginal dose of radiation correlates with increasing rates of nidus obliteration, as measured on MRI. At a threshold of 15 Gy, the odds ratio of successful obliteration is 3.743. A detailed study of dose-response suggests that this is a logistical function, with the inflection point near 16 Gy, and the plateau between 18 and 25 Gy, with a maximum obliteration rate of about 88% ( Fig. 1 ). However, it is also important to note that increasing dose can also lead to increased complications, such as radiation necrosis, and dose selection should also take into account the various regions of the brain. For example, the dose tolerance of surrounding brain tissue is significantly lower in the area around the thalamus than around the frontal lobe. The homogeneity index is another measure of radiation delivered. It is a ratio of the maximum dose to the prescribed dose. Increased homogeneity index is associated with slower nidus obliteration.
Although the natural history of AVF is unpredictably variable, AVF outcomes in patients receiving treatment are known to depend on the pattern of drainage. The Borden Classification system classifies AVFs into three types (see Table 1 ). It associates lower rehemorrhage rates for drainage into a dural venous sinus or meningeal vein versus a cortical vein, and also with anterograde flow versus retrograde flow. The Cognard system further distinguishes AVFs based on dilation of the draining vein and considers spinal perimedullary veins as a separate type. Because higher-grade lesions more often present with hemorrhage and acute risk of rehemorrhage, they are typically treated with surgical or endovascular techniques, limiting the statistical power of studies that report SRS outcomes to identify differences between grades. Of note, AVFs involving the cavernous sinus are associated with better obliteration rates. However, this may be confounded by a higher rate of spontaneous resolution in cavernous sinus AVFs.
Radiosurgery outcomes in treatment of AVM s and AVF s
SRS may aim to control AVM symptoms or prevent morbidity and mortality from AVM hemorrhage, but many studies focus on the prevention of hemorrhage. In addition to hemorrhage events, studies also measure AVM nidus or AVF obliteration, which is typically very strongly associated and is thought to be causatively related to hemorrhage.
Hemorrhage Prevention
Hemorrhage occurs after SRS, particularly during the latent period, before complete obliteration by the initial SRS, repeat SRS, or other definitive treatment ( Tables 3 and 4 ). Although a number of conflicting studies have analyzed the risk of hemorrhage in the latent period, a recently published large retrospective study of 500 patients found that the risk is unchanged or slightly decreased (hazard ratio, 0.26; 95% CI, 0.10–0.68). After SRS but before nidus obliteration, annual hemorrhage rates are estimated at 4.8% to 7.9% per year for the first 2 years, then 2.2% to 5% per year in the third, fourth, and fifth years. However, after the nidus is obliterated, the risk of hemorrhage is near zero. In one large study where post-obliteration hemorrhage was observed, the risk of hemorrhage is estimated at 0.12 (hazard ratio; CI, 0.1–0.68) of the pretreatment risk.
Median Volume (cm 3 ) | Median Marginal Dose (Gy) | Follow-up (mo) | N | Obliteration (%) | Imaging Modality | Hemorrhage (%) | Death (%) | Source |
---|---|---|---|---|---|---|---|---|
3.3 | 20 | 39 | 293 | 68 | MRI or angiography | 6 | 3 | |
3.8 | 20 | 38 | 108 | 71 | angiography | 14 | 2 | |
4.48 | — | — | 54 | 76 | CT or MRI or angiography | 13 | — | |
6.64 | 16 | 26.8 | 62 | 35 | CT or MRI | 5 | — | |
7.3 | 18 | 42 | 127 | 64 | MRI or angiography | — | 1 | |
9.9 | 15 | — | 330 | 55 | MRI | 8 | — | |
17.7 | 18 | — | 75 | 56 | MRI or angiography | 12 | 6 | |
48.8 | 13.9 | 109 | 44 | 34 | MRI or angiography | 7 | — |
Grade (Mean RBAS, Median SM) | Median Volume (cm 3 ) | Median Marginal Dose (Gy) | Follow-Up (mo) | N | Obliteration (%) | Imaging Modality | Hemorrhage (%) | Death (%) | Source |
---|---|---|---|---|---|---|---|---|---|
1.69 | 5.2 | 18 | 24 | 65 | 55 | CT or angiography | 9 | — | |
1.83 | 3.8 | 18 | 45 | 56 | 43 | MRI or angiography | 13 | 9 | |
1.63 | 1.5 | 20 | 72 | 45 | 66 | Angiography | 4 | 0 | |
— | 1.35 | 18.4 | 52.2 | 29 | 41 | Angiography | 17 | 14 | |
III | 4.3 | 25 | 28 | 19 | 74 | Angiography | — | — | |
— | 9.1 | 18.75 | 23 | 15 | 47 | Angiography | — | — | |
1.51 | 2.8 | 16.2 | 39 | 42 | 62 | MRI or angiography | 14 | 7 |
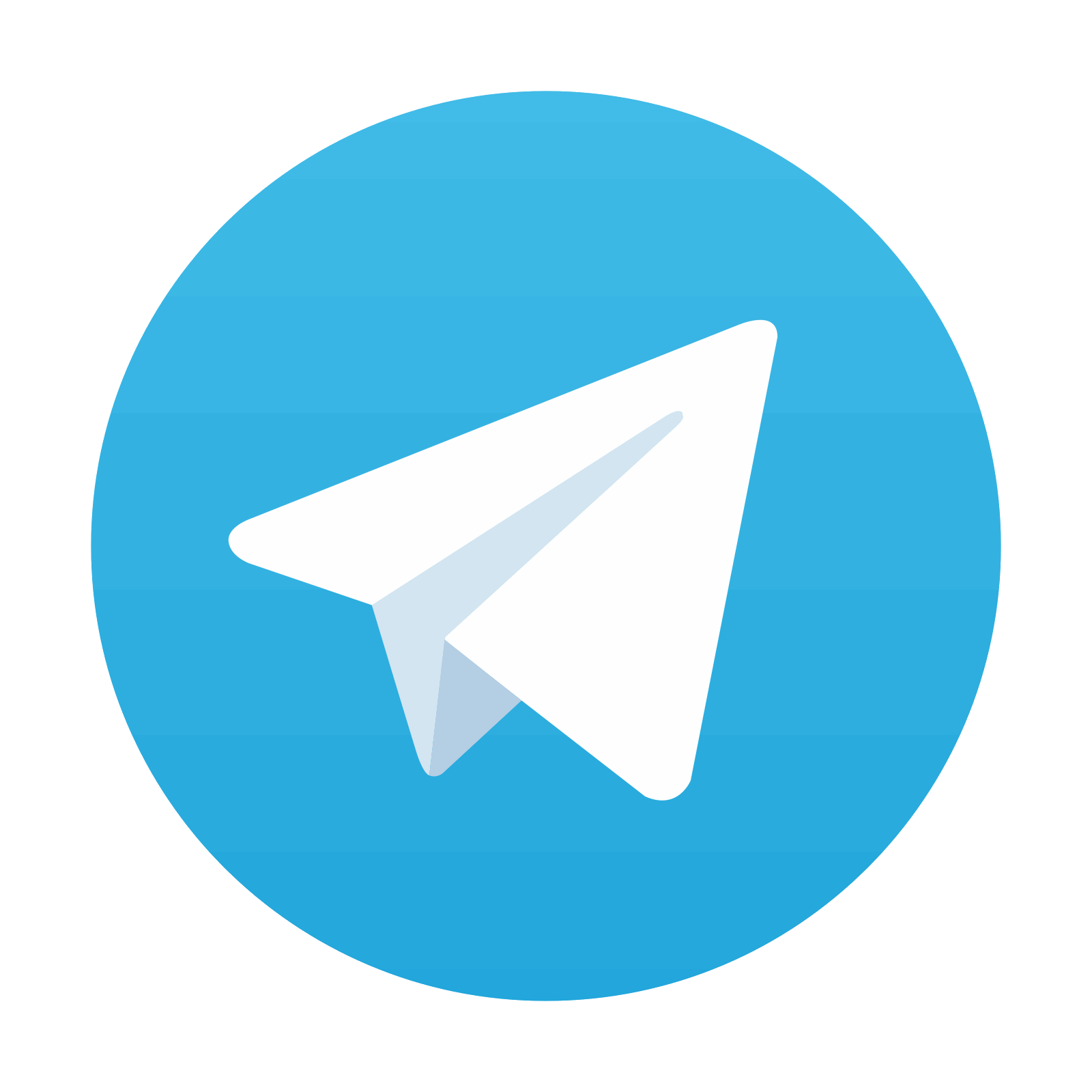
Stay updated, free articles. Join our Telegram channel

Full access? Get Clinical Tree
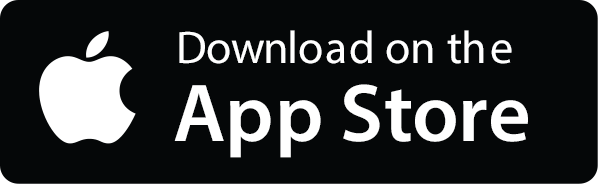
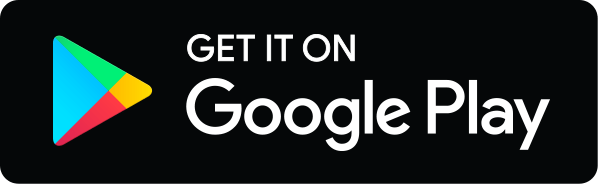
