Stereotactic radiosurgery for intracranial arteriovenous malformations (AVMs) has been performed since the 1970s. When an AVM is treated with radiosurgery, radiation injury to the vascular endothelium induces the proliferation of smooth muscle cells and the elaboration of extracellular collagen, which leads to progressive stenosis and obliteration of the AVM nidus. Obliteration after AVM radiosurgery ranges from 60% to 80%, and relates to the size of the AVM and the prescribed radiation dose. The major drawback of radiosurgical AVM treatment is the risk of bleeding during the latent period (typically 2 years) between treatment and AVM thrombosis.
Key points
- •
Surgery is the treatment of choice for arteriovenous malformations (AVMs), because only surgery can immediately eliminate the risk of hemorrhage. In those lesions that are high risk for surgery, radiosurgery is an attractive alternative, with high success rates and low complication rates.
- •
When one radiosurgery treatment fails, retreatment frequently produces a cure.
- •
The risk of bleeding after radiosurgery seems to decrease within the natural history risk range until AVM thrombosis occurs.
- •
The risk of permanent radiation-induced side effects after AVM radiosurgery is approximately 2%.
Introduction
Stereotactic radiosurgery is the term coined by Lars Leksell to describe the application of a single, high dose of radiation to a stereotactically defined target volume. In the 1970s, reports began to appear documenting the successful obliteration of arteriovenous malformations (AVMs) with radiosurgery. When an AVM is treated with radiosurgery, a pathologic process seems to be induced that is similar to the response-to-injury model of atherosclerosis. Radiation injury to the vascular endothelium is thought to induce the proliferation of smooth muscle cells and the elaboration of extracellular collagen, which leads to progressive stenosis and obliteration of the AVM nidus, thereby eliminating the risk of hemorrhage.
The advantages of radiosurgery, compared with microsurgical and endovascular treatments, are that it is noninvasive, has minimal risk of acute complications, and is performed as an outpatient procedure requiring no recovery time for the patient. The primary disadvantage of radiosurgery is that cure is not immediate. Although thrombosis of the lesion is achieved in most cases, it commonly does not occur until 2 or 3 years after treatment. During the interval between radiosurgical treatment and AVM thrombosis, the risk of hemorrhage remains. Another potential disadvantage of radiosurgery is possible long-term adverse effects of radiation. In addition, radiosurgery has been shown to be less effective for lesions of more than 10 cm 3 in volume. For these reasons, selection of the optimal treatment of an AVM is a complex decision requiring the input of experts in endovascular, open surgical, and radiosurgical treatment.
This article reviews the literature on radiosurgery for AVMs. Topics reviewed include radiosurgery results (gamma knife radiosurgery, particle beam radiosurgery, linear accelerator radiosurgery), hemorrhage after radiosurgery, radiation-induced complications, and repeat radiosurgery.
Introduction
Stereotactic radiosurgery is the term coined by Lars Leksell to describe the application of a single, high dose of radiation to a stereotactically defined target volume. In the 1970s, reports began to appear documenting the successful obliteration of arteriovenous malformations (AVMs) with radiosurgery. When an AVM is treated with radiosurgery, a pathologic process seems to be induced that is similar to the response-to-injury model of atherosclerosis. Radiation injury to the vascular endothelium is thought to induce the proliferation of smooth muscle cells and the elaboration of extracellular collagen, which leads to progressive stenosis and obliteration of the AVM nidus, thereby eliminating the risk of hemorrhage.
The advantages of radiosurgery, compared with microsurgical and endovascular treatments, are that it is noninvasive, has minimal risk of acute complications, and is performed as an outpatient procedure requiring no recovery time for the patient. The primary disadvantage of radiosurgery is that cure is not immediate. Although thrombosis of the lesion is achieved in most cases, it commonly does not occur until 2 or 3 years after treatment. During the interval between radiosurgical treatment and AVM thrombosis, the risk of hemorrhage remains. Another potential disadvantage of radiosurgery is possible long-term adverse effects of radiation. In addition, radiosurgery has been shown to be less effective for lesions of more than 10 cm 3 in volume. For these reasons, selection of the optimal treatment of an AVM is a complex decision requiring the input of experts in endovascular, open surgical, and radiosurgical treatment.
This article reviews the literature on radiosurgery for AVMs. Topics reviewed include radiosurgery results (gamma knife radiosurgery, particle beam radiosurgery, linear accelerator radiosurgery), hemorrhage after radiosurgery, radiation-induced complications, and repeat radiosurgery.
Reported efficacy of AVM radiosurgery
Gamma Knife Radiosurgery
The gamma knife is a dedicated radiosurgery machine, invented by Lars Leksell and his colleagues in 1968, in Sweden. Current gamma knife equipment contains 192 cobalt-60 sources, held in a hemispherical array. These sources emit high-energy photons (called gamma rays). Each source creates an independent beam path through normal brain to the radiosurgery target. All of the beams coincide at the target, delivering a high dose of radiation, but each individual beam path has a small dose, creating a steep dose gradient, with little risk to normal tissue.
Steiner pioneered gamma knife radiosurgery for AVMs and has published multiple reports. He reported 1-year occlusion rates ranging from 33.7% to 39.5% and 2-year occlusion rates ranging from 79% to 86.5%. However, these results were optimized by retrospectively selecting patients who received a high treatment dose. For example, in one report he stated that, “…a large majority of patients received at least 20–25 Gy of radiation. Of the 248 patients treated before 1984, the treatment specification placed 188 in this group.” The reported thrombosis rates in this article only applied to these 188 patients (76% of the total series).
Yamamoto and colleagues reported on 25 Japanese patients treated on the gamma unit in Stockholm, but followed in Japan. The 2-year thrombosis rate in those AVMs that were completely covered by the radiosurgical field was 64%. One patient had complete thrombosis at 3-year angiography and an additional patient had complete thrombosis at 5-year angiography, for a total cure rate of 73%. In another article, these investigators reported angiographic cures in 6 of 9 (67%) children treated in Stockholm or Buenos Aires and followed in Japan. Yamamoto and colleagues reviewed the long-term follow-up results of a group of 40 Japanese patients undergoing gamma knife radiosurgery for AVMs in 3 different countries (Argentina, Sweden, and the United States). In this group of patients, the mean lesion volume was only 3.7 cm 3 . Twenty-six patients (65%) were subsequently found to have angiographically confirmed nidus obliteration at 1 to 5 years after radiosurgery.
Kemeny and colleagues reported on 52 patients with AVMs treated with gamma knife radiosurgery. They all received 25 Gy to the 50% isodose line. At 1 year, 16 patients (31%) had complete thrombosis and 10 patients (19%) had almost complete thrombosis. The results were better in younger patients and in patients with laterally located AVMs. There was no difference in outcome between small (<2 cm 3 ), medium (2–3 cm 3 ), and large (>3 cm 3 ) AVMs.
Lunsford and colleagues reported on 227 patients with AVMs treated with gamma knife radiosurgery. The mean dose delivered to the AVM margin was 21.2 Gy. Multiple isocenters were used in 48% of the patients. Seventeen patients underwent 1-year angiography, which confirmed complete thrombosis in 76.5%. As indicated in the article, “this rate may be spurious since many of these patients were selected for angiography because their magnetic resonance (MR) image had suggested obliteration.” Among 75 patients who were followed for at least 2 years, 2-year angiography was performed in only 46 (61%). Complete obliteration was confirmed in 37 of the 46 (80%). This thrombosis rate strongly correlated with AVM size, as follows: less than 1 cm 3 , 100%; 1 to 4 cm 3 , 85%; 4 to 10 cm 3 , 58%. This group also reported on a group of 65 operable AVMs treated with radiosurgery. Of 32 patients who subsequently underwent follow-up angiography, 84% showed complete thrombosis. In a later publication from this group, Pollock and colleagues reported on 313 patients with AVMs. An angiographic cure rate of 61% was achieved.
Karlsson and colleagues reported on 945 AVMs treated in Stockholm between 1970 and 1990 with the gamma knife. The overall occlusion rate was 56%. Shin and Maruyama reported on 400 cases treated with gamma knife radiosurgery. They reported a 72% obliteration rate at 3 years after treatment. Other groups have reported on gamma knife radiosurgery results for specific sites like brainstem, motor cortex, or basal ganglia, or in groups like children.
Particle Beam (Proton or Helium) Radiosurgery
Particle beam radiosurgery facilities use cyclotronlike devices to accelerate subatomic particles to very high speeds before aiming them at patients. They have a unique physical property, called the Bragg peak effect, which results in most of the beam’s energy being deposited at a predictable depth in tissue, with little exit dose. This property is theoretically ideal for radiosurgery but its usefulness has been limited by the need to spread out the Bragg peak to fit anatomic lesions, restrictions in beam number compared with gamma knife or linear accelerator systems, and the high cost of the facilities needed for such systems.
Kjellberg and colleagues published multiple reports on the use of Bragg peak proton particle radiosurgery for AVMs. Their New England Journal of Medicine article in 1983 provided details on long-term follow-up of their first 75 patients. It includes a well-known diagram of doses versus complications. However, only 20% of these patients had complete nidus obliteration on follow-up angiography. Seifert and colleagues reported on 63 patients referred to the United States for Bragg peak proton beam therapy and followed in Europe. Complete nidus obliteration was only seen in 10 patients (15.9%). Several patients had radiation-induced side effects.
In contrast, Steinberg and colleagues, in an analysis of 86 AVMs treated with a helium particle beam radiosurgical system, reported a 29% 1 year thrombosis, 70% 2 year thrombosis, and 92% 3 year thrombosis rate. The best results were obtained with small lesions and high doses. At first a treatment dose of 34.6 Gy was used but a higher than expected neurologic complication rate (20% for the series) led to lower doses (7.7–19.2 Gy). No patients treated with the lower dose range had complications.
Linear Accelerator Radiosurgery
Linear accelerators are devices that use microwave energy to accelerate electrons to very high speeds. The energetic electrons collide with a heavy metal alloy in the head of the machine. Most of the collision energy is lost as heat, but a small percentage results in high-energy photon radiation (called x-rays because they are electronically produced). These photons are virtually identical to those produced by the spontaneous decay of radioactive cobalt in the gamma knife. They are collimated and focused on the radiosurgical target. Linear accelerator systems rotate the beam around the patient, from many different angles, to create the hundreds-of-beams approach used by the gamma knife to provide high doses at the target but low doses to normal tissues.
Betti pioneered linear accelerator radiosurgery and reported on the results of 66 AVMs treated with a linear accelerator radiosurgical system. Doses of no more than 40 Gy were used in 80% of patients. He found a 66% 2-year thrombosis rate. The percentage of cured patients was highest when the entire malformation was included in the 75% isodose line (96%) or the maximum diameter of the lesion was less than 12 mm (81%).
Colombo and colleagues reported on 97 patients with AVMs treated with a linear accelerator system. Doses from 18.7 to 40 Gy were delivered in 1 or 2 sessions. Of 56 patients who were followed for longer than 1 year, 50 underwent 12-month follow-up angiography. In 26 patients (52%), complete thrombosis was shown. Fifteen of 20 patients (75%) undergoing 2-year angiography had complete thrombosis. He reported a definite relationship between AVM size and thrombosis rate, as follows: lesions less than 15 mm in diameter had a 1 year obliteration rate of 76% and a 2 year rate of 90%. Lesions 15 to 25 mm in diameter had a 1-year thrombosis rate of 37.5% and a 2-year rate of 80%. Lesions greater than 25 mm in diameter had a 1-year thrombosis rate of 11% and a 2-year rate of 40%. In a later report, Colombo and colleagues reported follow-up on 180 radiosurgically treated AVMs. The 1-year thrombosis rate was 46%, and the 2-year rate was 80%.
Souhami and colleagues reported on 33 AVMs treated with a linear accelerator system. The prescribed dose at isocenter varied from 50 to 55 Gy. A complete obliteration rate of 38% was seen on 1-year angiography. For patients whose arteriovenous malformation nidus was covered by a minimum dose of 25 Gy, the total obliteration rate was 61.5%, whereas none of the patients who had received less than 25 Gy at the edge of the nidus obtained a total obliteration.
Loeffler and colleagues reported on 16 AVMs treated with a linear accelerator system. The peripheral prescribed dose was 15 to 25 Gy, typically to the 80% to 90% line. The total obliteration rate was 5 of 11 (45%) at 1 year and 8 of 11 (73%) at 2 years after treatment.
Engenhart and colleagues reported on the treatment of 212 patients in Heidelberg. “Above a threshold dose of 18 Gy, the obliteration rate was 72%. Radiation induced late complications were seen in 4.3%.”
Schlienger and colleagues reported on 169 patients treated in Paris. The overall obliteration rate was 64%. Success rates were higher in smaller lesions, in lesions not embolized, in lesions treated with higher doses, and lesions treated with 1 isocenter. Two patients experienced radiation-induced side effects.
Andrade-Souza and colleagues reported on 38 rolandic area AVMs treated in Toronto. Complete nidus obliteration was seen in 60.5%. Two patients experienced radiation-induced side effects.
Friedman and colleagues published multiple reports on the University of Florida experience with radiosurgery for AVMs. They documented occlusion rates of 80% for lesions less than 10 cm 3 in volume, with radiation-induced complications in the 2% range. Factors favoring occlusion include small AVM size, low Spetzler-Martin score, high peripheral radiation dose, and compact nidus morphology. Detailed dosimetric analysis suggested that 12 Gy volume and eloquent location correlated with transient radiation-induced complications.
Results of AVM radiosurgery at the University of Florida
Between 5/18/88 and 2/5/13, 699 AVMs were treated on the University of Florida radiosurgery system. There were 350 men and 349 women in the series. The mean age was 39 years (range 4–85 years). Patients presented with hemorrhage (225), seizure (264), headache/incidental finding (232), and progressive neurologic deficit (33). Thirty-eight patients had undergone prior subtotal microsurgical AVM excision. Fifty-five patients had undergone at least 1 embolization procedure. Patients were screened with a vascular neurosurgeon before consideration of radiosurgery.
The median lesion volume was 6.7 cm 3 (0.1–80 cm 3 ). The treatment volume was determined in all cases by performing a computerized dose volume histogram of the treatment isodose shell (which was constructed to conform closely to the AVM nidus). Lesion volumes were stratified as follows: A, less than 1 cm 3 ; B, 1 to 4 cm 3 ; C, 4 to 10 cm 3 ; D, greater than 10 cm 3 . Spetzler-Martin grades were distributed as follows: grade I, 41 patients; grade II, 229 patients; grade III, 291 patients; and grade IV, 118 patients. The median radiation dose to the periphery of the lesion was 17.5 Gy (range 7.5–25 Gy). This treatment dose was delivered to the 80% isodose line when single isocenter plans were used, and to the 70% line when multiple isocenters were used. Three-hundred and eleven patients were treated with a single isocenter, 96 patients with 2 isocenters, 81 patients with 3 isocenters, 53 patients with 4 isocenters, and 143 patients with 5 isocenters or more.
Mean follow-up duration for the AVM group was 33 months (2–166 months). Follow-up generally consists of clinical examination and MR imaging (MRI) scanning at 1-year intervals after treatment, unless clinical symptoms indicated more frequent follow-up. When possible, follow-up is performed in Gainesville, Florida, otherwise scan and examination results are forwarded by the patient’s local physician.
At first, all patients were asked to undergo angiography at yearly intervals, regardless of the MRI findings. After the first 50 patients were treated, it was decided to defer angiography until MRI/MR angiography strongly suggested complete thrombosis. Furthermore, if complete thrombosis was not identified 3 years after radiosurgery, repeat radiosurgery was undertaken in an effort to obliterate any remaining nidus (discussed later).
An angiographic cure required that no nidus or shunting remain on the study, as interpreted by a neuroradiologist and the treating neurosurgeon. Of the 192 follow-up angiograms performed to date, 147 (77%) have shown complete AVM obliteration. Using this traditional method of reporting, the following angiographic cure rates were seen in the various size categories: A, 93%; B, 86%; C, 83%; D, 53%. However, angiographic success rates can be misleadingly high, because angiography may not be done if MRI shows residual nidus. If angiography or MRI follow-up results at 3 years are accepted, the following results are seen in 367 patients: A, 93%; B, 83%; C, 63%; D, 35%. In addition, if radiosurgical retreatments are included (which can salvage several initial failures, as discussed later), the patient success rates are as follows: A, 100%; B, 93%; C, 84%; D, 75%.
Why does radiosurgery fail?
In an effort to clarify the causes of radiosurgical failure, we examined 36 patients who underwent repeat radiosurgery after an initial failure to obliterate their AVMs and compared them with 72 patients who were cured during the same time period. An image fusion methodology was used to fuse the treatment plan created at the first treatment to the computed tomography (CT) scan obtained at the time of retreatment. Two patients were excluded from the targeting error analysis because the nidus was too small to visualize on CT. Of the remaining 34 patients in whom the original treatment failed, 9 (26%) had a partial targeting error at the time of the first treatment.
The retreatment group had statistically significantly higher Spetzler-Martin grade, larger AVM size, and lower treatment dose compared with the group of patients who were cured. Statistical analysis also showed that patients treated with a peripheral dose of less than 15 Gy had a higher failure rate. In addition, patients with AVM volumes greater than 10 cm 3 had a higher failure rate.
Other radiosurgery groups have also published analyses designed to identify factors that might predict radiosurgical success or failure. Pollock and colleagues found that the following factors predicted success: low AVM volume, few draining veins, young age, and superficial location. Prior embolization of the AVM was a negative predictor. This group also published a review of 45 patients who underwent repeat radiosurgical treatment after an initial treatment failed to obliterate their AVMs. In this study, causes of radiosurgical failure were identified as follows: in 5 patients (11%), the entire AVM was not visualized secondary to incomplete angiography (2 vessels instead of 4 vessels) or inadequate angiographic technique (failure to perform superselective angiography). In 3 patients (7%), the AVM recanalized after previous embolization. In 4 patients (9%), the AVM nidus reexpanded after resorption of a prior hematoma that had compressed the vessels within the nidus. In 21 patients (46%), the three-dimensional shape of the AVM nidus was not appreciated secondary to reliance on biplanar angiography alone. In the remaining patients, a definite cause for failure could not be determined. The investigators thought that the AVMs in these patients had some form of radiobiological resistance; that is, failure to be obliterated despite proper planning and adequate dose delivery. In an earlier analysis by the same group, the dose to the periphery (D min ) of the target was found to be the most significant predictor of success. In that analysis, neither volume nor maximum dose was predictive. Problems defining the complete AVM nidus were cited as significant limitations to successful AVM obliteration.
The Stockholm group published an analysis designed to define predictive factors for radiosurgical obliteration of AVMs. Analyzing a 945-patient subset of the 1319 patients with AVMs treated with the gamma knife from 1970 to 1990, they again identified peripheral dose as the most significant predictive factor. The higher the minimum dose, the higher the obliteration rate, up to 25 Gy. The obliteration rate in the 268 cases that received this minimum dose (25 Gy) was 81%. High average dose and low AVM volume also predicted success. A high average dose shortened the latency to AVM obliteration. They proposed that the product of the cubed root of the AVM volume and the peripheral dose (the K index) would serve as a good combined predictor of success and found that a K index of 27 was optimal. Obliteration rates increased with increasing K values up to 27, beyond which no further improvement was observed.
Yamamoto and colleagues reviewed the long-term follow-up results of a group of 40 Japanese patients undergoing gamma knife radiosurgery for AVMs in 3 different countries (Argentina, Sweden, and the United States). In this group of patients, the mean lesion volume was only 3.7 cm 3 . Twenty-six patients (65%) were subsequently found to have angiographically confirmed nidus obliteration at 1 to 5 years after radiosurgery. Thirteen (32.5%) patients failed radiosurgery based on follow-up angiogram at 3 to 7 years after treatment. In their retrospective analysis, they discovered that the nidus had only partially been covered at the time of the first treatment in 6 of the 13 (46%) patients who subsequently failed.
The importance of targeting error as a cause for radiosurgical failure merits brief discussion. In the Pittsburgh series, 67% of all failures were attributed to targeting error resulting from inadequate imaging or obscuration of the AVM nidus by a hematoma. In Yamamoto’s analysis, targeting error was responsible for 46% of all failures. A similar analysis by Gallina and colleagues attributed 10 of 17 (59%) AVM radiosurgery failures to inadequate targeting. In our experience, targeting error was also an important cause of failure, accounting for 26% of failures, which may support the importance of using a three-dimensional database, such as contrast-enhanced CT or MR angiography, for targeting, as opposed to stereotactic angiography alone.
Zipfel and colleagues explored the effect of AVM morphology on radiosurgical success. In 268 patients, diffuse nidus morphology and neovascularity were statistically significant predictors of failure. Meder and colleagues, in an earlier study, also documented the importance of angioarchitecture.
Pollock and Flickinger developed an AVM grading system that accurately predicts the chances of an excellent outcome (meaning occlusion and no radiation-induced side effects) after radiosurgery. In a multivariate analysis of 220 patients, 5 variables related to excellent outcome: AVM volume, patient age, AVM location, previous embolization, and number of draining veins. Further analysis permitted the removal of 2 variables (embolization and draining veins), yielding the following predictive equation: AVM score = 0.1 (AVM volume) + 0.02 (patient age in years) + 0.3 (location of lesion). Locations scores are: frontal or temporal = 0; parietal, occipital, intraventricular, corpus callosum, cerebellar = 1; basal ganglia, thalamic, brainstem = 2. All patients with an AVM score less than 1 had an excellent outcome, compared with only 39% of patients with a score higher than 2. The Spetzler-Martin score and the K index did not correlate with excellent outcome. This group subsequently showed that the AVM score also predicted the chance of a worsened Modified Rankin Scale score after radiosurgical treatment. Andrade-Souza and colleagues verified the predictive value of the AVM score in 136 patients treated with linear accelerator–based radiosurgery. The University of Florida series was analyzed with this technique and we also confirmed its validity.
Hemorrhage after radiosurgery
There are significant pitfalls to be avoided in the analysis of hemorrhage risk after radiosurgery. First, because radiosurgery is usually successful, a large number of patients are eliminated from the at-risk pool during the first and second years after radiosurgery. Failure to adequately account for this leads to the false impression that the smaller number of hemorrhages occurring greater than 1 year after treatment are caused by some protective effect. The decreasing number of hemorrhages is statistically caused by the decreasing number of patients at risk. Second, because a significant number of patients present with hemorrhage in any radiosurgery series, and because these patients may have an increased risk of hemorrhage for the first 6 months after hemorrhage, the incidence of bleeding in the first 6 months after treatment may seem, in some series, to be increased. This observation is likely not a direct effect of radiosurgery, but rather a reflection of the inclusion of this group of patients with a higher-than-normal risk of hemorrhage. Series that treat a large percentage of patients presenting with hemorrhage (as opposed to seizures or headache) might for this reason be expected to have an increased hemorrhage rate during the first 6 months after treatment. Third, and most important, the incidence of hemorrhage in patients with AVMs is small, which means that the effect of a slight alteration in the number of hemorrhages over a given period of time may, without the benefit of statistical analysis, significantly skew the conclusions in this type of analysis. Assuming a constant baseline AVM hemorrhage rate of 3 per 100 person-years follow-up (or 3% per year) in untreated patients, 726 person-years of follow-up would be required to have a 95% chance of detecting a reduction in the hemorrhage rate to 1 per 100 person-years of follow-up (or 1% per year) in treated patients at a 0.05 significance level. Given the high cure rate of radiosurgery, a large number (thousands) of patients treated would be needed to generate a sufficient number of patient follow-up years in years 2, 3, 4, and so forth, in order to yield statistically valid information. These factors must be kept in mind when interpreting some of the articles discussed later. Any attempt to elucidate the question of AVM bleeding after radiosurgery without benefit of detailed statistics must be viewed with skepticism.
The issue of AVM hemorrhage after radiosurgical treatment was first discussed by investigators using particle beam methodology. Kjellberg and colleagues initially reported 2 deaths from hemorrhage in the first year after treatment of their first 75 patients. In a subsequent report of 389 patients followed for at least 2 years, 8 had succumbed from hemorrhage in the first 2 years after treatment. Only 1 had died thereafter, for a 0.27% mortality in those patients greater than 2 years after treatment. At first, these investigators suggested that only those patients presenting with hemorrhage were at risk for subsequent lethal rebleeding. However, later reports document fatal hemorrhage in patients presenting with seizure only. It should be emphasized that these investigators reported a total hemorrhage rate of 2.4% per year (lethal and nonlethal) for those patients more than 2 years out from treatment. Whether this differs from the natural history of the disease is debatable.
Other particle beam proponents have also addressed the hemorrhage question. Steinberg and colleagues reported 10 hemorrhages (2 fatal), occurring between 4 and 34 months after radiosurgical treatment, in a series of 86 patients (12%). Two of these patients had hemorrhages in the third year after treatment, suggesting no protective effect unless complete obliteration was achieved. Seifert and colleagues analyzed a series of 68 patients treated with proton beam therapy in the United States. Eighteen patients deteriorated neurologically. Five of them had hemorrhages, of which 2 were fatal.
Gamma knife radiosurgeons have studied this question as well. Lunsford and colleagues, in an initial report on AVM treatment with the Pittsburgh gamma knife, noted that 10 patients (4%) in a series of 227 patients had experienced hemorrhage. Two of these patients died. Pollock and colleagues, in a study of 65 patients with operable AVMs, noted that 5 patients had a hemorrhage (7.7%), all within 8 months of radiosurgery. Two of these patients died. Steiner and colleagues statistically analyzed bleeding in 247 consecutive AVM cases. The Kaplan-Meier approach showed a risk of nearly 3.7% per year until 5 years after radiosurgery, at which point a plateau was reached. This plateau was thought to be caused by the small number of data points for that time period and not to indicate any protective effect. Karlsson and colleagues reported an analysis of bleeding in 1565 patients treated with the Stockholm gamma knife. They thought that the risk of hemorrhage was decreased, even with incomplete obliteration. They also reported that increasing age and increasing AVM volume correlated with an increased risk of hemorrhage.
Betti and colleagues, in their pioneering report on linear accelerator radiosurgery for AVMs, documented hemorrhage in 5 of 66 (8%) of their patients. These hemorrhages occurred at 12, 18, 22, 25, and 29 months after treatment. All had a prior history of hemorrhage. Two of these patients died. Colombo and colleagues also reported a detailed analysis of 180 patients. Fifteen patients had hemorrhages and 5 of them succumbed. In cases in which the AVM nidus was totally irradiated, the bleeding risk decreased from 4.8% during the first 6 months to 0% starting from the 12th month of follow-up. In partially irradiated cases, the bleeding risk increased from 4% in the first 6 months to 10% from the sixth to the 18th month and then down to 5.5% from the 18th to the 24th month. No bleeding was observed thereafter.
Our analysis of this issue did not reveal any postradiosurgical alteration in bleeding risk from the 3% to 4% per year expected based on natural history. A similar study by the Pittsburgh group confirmed that “stereotactic radiosurgery was not associated with a significant change in the hemorrhage rate of AVMs during the latency interval before obliteration.”
As discussed earlier, Karlsson and colleagues reported an increased risk of AVM hemorrhage with increasing AVM size. Colombo and colleagues found a higher incidence in subtotally irradiated AVMs, most of which were presumably large lesions. He also reported a statistically increased risk in patients treated more inhomogeneously (to a lower isodose line). In a subsequent article, his group attributed this observation to the earlier thrombosis of the portion of the AVM receiving the highest dose of radiation, with shunting of blood into the remaining nidus, increasing the risk of hemorrhage.
In our series, a correlation between AVM volume and the risk of hemorrhage was also found. Ten of the 12 AVMs that bled were greater than 10 cm 3 in volume. In addition, the correlation of hemorrhage with low dose and low isodose line treated was likely caused by the deliberate use of low doses and multiple isocenter treatments (to low isodose lines) in large AVMs. Of equal importance are those factors that did not statistically correlate with bleeding risk. In this study, neither age nor history of prior hemorrhage correlated with the incidence of hemorrhage.
Pollock and colleagues found a significant correlation between the incidence of postradiosurgical hemorrhage and presence of an unsecured proximal aneurysm, and recommended that such aneurysms be obliterated before radiosurgery. The same group also studied factors associated with bleeding risk of AVMs and found 3 AVM characteristics to predict greater hemorrhage risk: (1) history of prior bleed, (2) presence of a single draining vein, and (3) diffuse AVM morphology. Nataf and colleagues analyzed bleeding after radiosurgery for 756 patients with AVMs. The actuarial hemorrhage rate was 3.08% per year per patient. It increased from 1.66% the first year to 3.87% in the 5th year after radiosurgery, but was never statistically different from the rate before radiosurgery. Intranidal aneurysms, complete coverage, and minimum dose correlated with the risk of hemorrhage.
Maruyama and colleagues performed a retrospective observational study of 500 patients. Compared with the period between diagnosis and radiosurgery, the risk of hemorrhage decreased by 54% during the latency period, and by 88% after obliteration. The risk of hemorrhage was not eliminated by angiographic obliteration. Other investigators have also reported rare cases of AVM bleeding after documented angiographic obliteration.
In summary, the major drawback of radiosurgical AVM treatment is the risk of bleeding during the latent period (typically 2 years) between treatment and AVM thrombosis. Most studies suggest that the natural history risk of hemorrhage remains unchanged until thrombosis. Some suggest that the bleeding risk decreases, even before thrombosis. Even after thrombosis occurs, there seems to be a small risk of continued hemorrhage. At this time, only surgery can immediately and completely eliminate the risk of AVM bleeding.
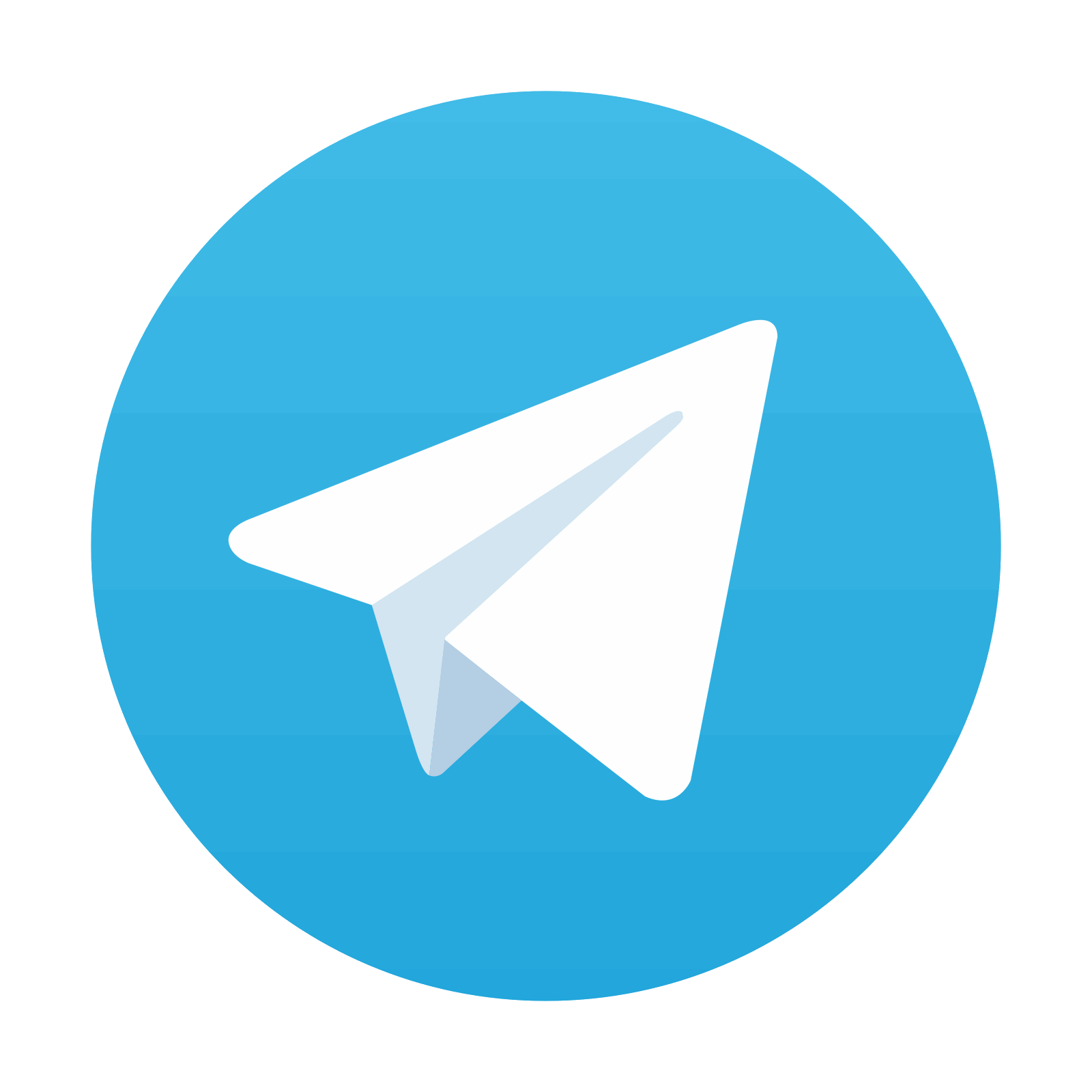
Stay updated, free articles. Join our Telegram channel

Full access? Get Clinical Tree
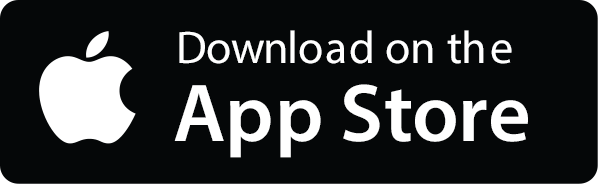
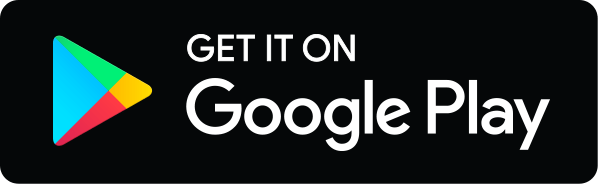