11 Tumors of the spine and spinal elements result in a considerable amount of morbidity. Primary tumors of the spine are relatively rare, but when present frequently cause symptoms and historically have proven very difficult to treat.1 Metastatic disease is severalfold more frequent. Nearly 200,000 cases of spinal metastases are identified in North America each year.1–3 Some 10% of these can be expected to result in neural element compression. In the setting of better multimodality treatment for cancer and improved long-term survival for its sufferers, it is likely that these numbers will only grow in the future. Therefore, it is becoming ever more important for clinicians to be well versed in the diagnosis and management of spinal tumors. The goals for the treatment of spinal tumors are several and include the following: prevention of local disease progression, preservation of spinal structural stability, preservation of neurological function, and the abolishment of pain.4 Traditionally, achieving these goals has involved treatment by surgery, radiation therapy, or chemotherapy, alone or in some combination.5 Over the last 2 decades, clinicians have become acutely aware of the benefits of applying the concept of minimal invasiveness to these modalities to offer maximum treatment effect with the least adverse sequelae. Whether molecularly targeting a specific kinase cascade or applying minimally invasive surgical techniques, attempts at the preservation of unaffected normal tissues are clearly advantageous. A similar paradigm has been applied to the field of spinal radiotherapy. Conventional radiation therapy, the delivery of one or two low-precision and nonconformal radiation beams, is a well-established treatment modality for malignancies of the spine and is often the initial approach taken.5–11 Unfortunately, the effectiveness of conventional radiation therapy to the spine is limited by the relative intolerance of certain surrounding tissues to high doses of radiation, in particular the spinal cord and other neural elements. Thus treatment doses far below the optimal therapeutic doses are applied, resulting in frequent recurrence or progression of disease due to an insufficient radiobiological effect.12–14 In contrast, the precise application of a tightly confined radiation dose to the desired treatment area, as has been the well-documented experience for intracranial stereotactic radiosurgery, should serve to allow for targeted optimal dosing, better tumor and symptom control, with a greatly reduced likelihood of injury to adjacent normal tissue structures.12,15–23 With the advent of improved imaging techniques, advances in radiation delivery, and computerized treatment planning, stereotactic spinal radiosurgery has become a valuable tool for the treatment of selected patients with both primary and metastatic tumors of the spine. Radiosurgery can be defined as the precise delivery of a highly conformal, large radiation dose to a specific target via a stereotactic approach.24 Spinal radiosurgery draws its origins from the use of radiosurgery for the treatment of benign and malignant intracranial disease.25–30 Conventional intracranial radiosurgery utilizes a frame-based system that requires the application of a rigid frame to the skull for immobilization and localization of the target lesion in space. Intracranial radiosurgery is feasible because lesions within the skull have a fixed relationship in space to the skull itself. Therefore, with a rigidly applied stereotactic frame serving as a fiducial reference system, the accurate targeting and delivery of a radiation dose via multiple beams is possible. Single-fraction, high-dose treatments have thus become a favorable methodology. This technique has proven an extremely effective tool for the control of pathologies ranging from meningiomas to brain metastases. Within the spine, lesions can have a specific fixed relationship in space to one or more vertebral segments. In contrast to the cranial vault, however, the spine is a highly mobile structure. Thus, to parallel intracranial radiosurgery with spinal radiosurgery, rigid immobilization of the spine near the target lesion with a stereotactic frame would be required. Early efforts utilized such invasive rigid external frames placed directly on the spine (i.e., the Hamilton-Lulu extracranial stereotactic frame), but these have not generally been adopted.31,32 An early alternative was the Lax extracranial stereotactic frame that utilized noninvasive immobilization with a vacuum pillow or foam pad within a computed tomographically (CT) detectable frame covering the patient from the head down to the midthigh.33,34 Since the first suggestion of using linear-accelerator (LINAC) based stereotactic spinal radiosurgery in the mid-1990s, the delivery of highly conformal high-dose radiation to spinal disease has been pursued by several centers.1,12,14,16–18,21,22,24,35–44 Moreover, spinal radiosurgery for primary and metastatic tumors has more recently demonstrated safety and efficacy.12,14,16,17,21,22,24,36,38–40,42,44 Improved imaging techniques for treatment planning and localization, combined with the advent of intensity-modulated radiation therapy (IMRT), allow clinicians to treat lesions anywhere from the paraspinal area to intramedullary locations with high target dose confirmation and sparing of normal tissues. Highly conformal target dosing to the spine provides the theoretical advantage of greatly limiting the likelihood of radiation myelitis. Despite an extensive experience with spinal radiation, little is known clinically regarding the tolerance of the human spinal cord to large single-fraction doses.22,45,46 Thus, the basis for our understanding regarding cord tolerance is derived from series in which external beam radiation was used and the entire thickness of the cord was irradiated. The total doses (TD) at which there is a 5% probability of radiation myelitis after 5 years for 5–20 cm lengths of spinal cord has been estimated to be 5 Gy.47 These conclusions are extrapolated from data produced in the 1940s. Despite this, these estimations have been adopted widely by those administering spinal radiation. Thus, to decrease the risk of spinal cord necrosis, standard fractionation schemes have stated cord tolerance to be 45 to 50 Gy. A common fractionation scheme of 1.8 to 2 Gy per fraction for a total of 45 to 50 Gy falls within the radiation tolerance of the spinal cord. Doses of 8 Gy in a single fraction have been delivered to long portions of the spinal cord without reported myelopathy.48,49 One study of 172 patients receiving fractionated radiation therapy to cervical and thoracic segments reported nine cases of radiation-induced myelopathy with a fractionation schedule of 40 to 70 Gy over 2 to 3 weeks.50 Another series of 387 patients receiving large single-fraction treatments for bronchial carcinoma had 17 patients suffer radiation myelitis.51 The average total dose for these patients was 38 Gy. Of 109 patient receiving fractionated regimens of 57 to 62 Gy for head and neck cancers, seven developed myelopathy.7 Eight of 203 cases developed radiation myelitis in another series where patients were treated with a total dose of 54 to 60 Gy to the cervical and thoracic spine.52 Only one of another 652 patients, all of whom received standard fractionation total doses greater than 60 Gy, showed evidence of myelitis.53 Finally, of 350 patients treated with a total radiation dose of 33 to 43.5 Gy for chest tumors, three cases of radiation myelitis were reported.54 A spinal radiosurgery procedure can be divided into four unique components. First, the patient must be immobilized and/or have fiducials implanted for image guidance. Second, CT imaging is performed for treatment planning and generation of digitally reconstructed radiographs (DRRs). Third, the radiation dose planning is completed. Finally, the actual radiation dose is delivered to the patient. Completion of these tasks involves a team of individuals including a surgeon, a radiation oncologist, and a medical physicist. Spinal radiosurgery is usually performed in an outpatient setting. Treatment prescription involves a combination of volume and dose quantifications. The clinical target volume (CTV) includes the gross tumor volume (GTV) and any adjacent areas interpreted as having a high likelihood of tumor involvement. Both the CTV and all the structures for which it is critical to avoid toxic radiation dosing are identified during planning. This can be done by outlining the lesion (GTV) from CT or magnetic resonance imaging (MRI) fusion capability. Dosing is also determined on the basis of tumor histology and previous radiation exposure to normal tissues, especially the spinal cord. However, to date, little or no published data are available that provide guidelines for the most efficacious and least toxic dosing schedules. Dosing and fractionation schedules vary widely. It has been the practice at the University of Pittsburgh to apply a single-fraction radiosurgery paradigm on the basis of the large experience with the Leksell Gamma Knife (Elekta, Inc., Norcross, GA) for intracranial disease. A tumor dose maintained at 12 to 20 Gy to the 80% isodose line contoured to the edge of the target volume is used for single-fraction therapy. This provides a maximum intratumoral dose ranging from 15 to 30 Gy. Sixteen Gy to the tumor margin with a maximum intratumoral dose of 20 Gy has demonstrated excellent tumor control with minimal risk of radiation toxicity to the spinal cord or cauda equina.38 Other dosing schedules have been reported with 6 to 30 Gy in 1 to 5 fractions.12,17,18,22,23,55–58 During treatment planning, the spinal cord and/or cauda equina are outlined as critical structures. From the cauda equina distally, the entire spinal canal is outlined. For single-fraction schemes, the recommended maximum spinal cord dose is below 10 Gy to avoid the possibility of radiation-induced myelitis. A dose of up to 14 Gy appears to be a safe maximum dose to the cauda equina.46 The aforementioned constraints are applied together with the target dose prescription during treatment planning. To accomplish this, the treatment team uses the desired dose distribution and applies inverse treatment planning to design a field setup (beam angles) and appropriate beam intensities. Two principles required for successful radiosurgery are those of target localization and target immobilization. Precise patient setup and lesion localization are critical for the execution of a radiosurgery treatment, and various methodologies have been utilized to position and immobilize patients.1,48 One paradigm mirrors that of intracranial radiosurgery and involves the placement of a rigidly fixed frame in which the patient undergoes CT imaging and ultimately treatment.40 Subsequently, success has been achieved with frameless techniques. One approach for spinal radiosurgery has been to immobilize the patient in a noninvasive stereotactic body frame or immobilization cradle. These devices do not ensure that the patient will remain perfectly positioned, but by obtaining pre- and posttreatment imaging, acceptable immobilization results have been obtained for devices using this technique. One example of this is the near-simultaneous CT image-guided stereotactic radiotherapy system at MD Anderson Cancer Center. This system integrates a CT-on-rails scanner with a linear accelerator. Each patient is immobilized in a moldable body cushion vacuum wrapped with a plastic fixation sheet. Patients are transferred directly from the CT scanner to the linear accelerator couch via a rail system.23 Another example is the Memorial Stereotactic Body Frame (MSBF), developed at Memorial Sloan Kettering. The patient is externally immobilized with a series of pressure plates and has CT images obtained on a scanner in the same room as the linear accelerator. This pretreatment CT is automatically registered with the planning CT using bony landmarks. A second registration of the fiducial system in the treatment and planning scans allows positioning error in the body frame to be determined. Final positioning verification is completed through comparison of the cone beam CT, orthogonal portal images, and digitally reconstructed radiographs from the initial planning CT.57 A second approach involves the frequent acquisition of localizing images during the actual treatment and adjusting the patient’s position accordingly. The CyberKnife Image-Guided Radiosurgery System (Accuray, Inc., Sunnyvale, CA) exemplifies this approach. The CyberKnife system consists of a 6 megavolt (mV) compact linear accelerator mounted on a computer-controlled, six-axis robotic manipulator, and two orthogonally positioned diagnostic x-ray cameras.59,60 Images from the x-ray cameras are acquired and processed to identify radiographic features. They are then automatically compared with the planning CT so that precise tumor position is communicated via a real-time control loop to the robotic manipulator that aligns the radiation beam with the planned target.39,61–63 No additional immobilization is required other than the treatment couch because the positions of the bony structures are checked numerous times during treatment. As a result, any changes in tumor position are quickly detected and corrected during the course of treatment.1 Radiation delivery for radiosurgery requires precisely shaped beams. This is accomplished in part via 360 degree rotatable gantries to allow for multiple beam directions. Beam angles are selected to provide the ideal coverage of the target volume while sparing normal tissues. The beams can be further modified by the use of collimators that can attenuate the beam and precisely define the treatment field. A multileaf collimator (MLC) continuously adjusts the field size as the beams are shaped through movements of its leaves. For radiosurgery, the leaves are very small, allowing for accurate delivery of radiation to extremely small field sizes (micro MLC). The concept is referred to as intensity-modulated radiotherapy (IMRT). The Novalis Shaped-Beam Surgery unit (BrainLAB, Westchester, IL) is an example of this approach to spinal radiosurgery. It is a specialized treatment device consisting of a 6 mV linear accelerator equipped with a micro MLC and dual in-room kilovolt-age (keV) x-ray units. Two digitally reconstructed radiographs are generated from the simulation CT scan at the same orientation as the x-ray images. The system then compares the internal anatomy noted on the x-rays with that of the digitally reconstructed images and automatically adjusts the patient position based on isocenter deviations.56 The ability to acquire volumetric or three-dimensional pretreatment imaging has improved treatment accuracy and precision, and it allows for the detection of rotational errors in patient setup and enables robust automatic registration procedures. The TomoTherapy Hi-Art system (TomoTherapy, Inc., Madison, WI) integrates treatment planning and CT-based image-guided helical IMRT.35,64 It consists of a small 6 meV linear accelerator that has been mounted directly upon a CT scanner gantry. A 64-multileaf collimator modulates the beam output, making this device capable of delivering highly conformal radiation doses to multiple targets simultaneously. Finally, daily pretreatment megavolt CT scans can be co-registered with the planning CT scan to ensure accurate patient setup. Cone beam imaging uses a gantry-mounted kilovolt source and detector that can acquire several hundred projection images with each full gantry rotation. These images can be converted into CT-like axial slices via image reconstruction software. Cone beam scans provide high spatial resolution of bony and soft tissue structures, thus allowing for the setup of sites with submillimeter targeting errors.1
Stereotactic Spinal Radiosurgery for Primary and Metastatic Disease
Background of Stereotactic Spinal Radiosurgery
Spinal Cord Tolerance
Treatment Planning and Dosing
Image Guidance and Treatment Conformality
Stay updated, free articles. Join our Telegram channel

Full access? Get Clinical Tree
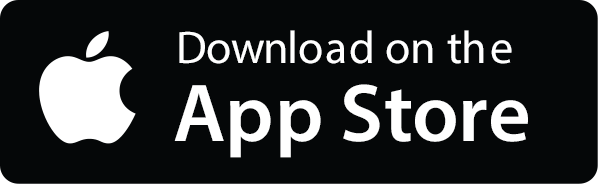
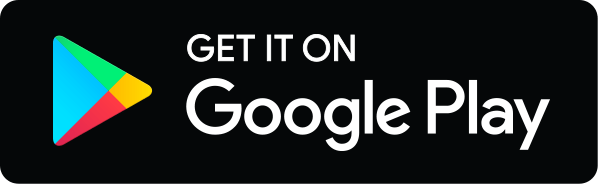