Chapter 19 Electrical stimulation for enhanced wound healing
INTRODUCTION
Chronic wounds are a continuing problem within the healthcare sector: the costs of care are high, thus effective care is of great importance. Such wounds are treated in a wide variety of ways, one of which is electrical stimulation based on the observed differences in electrical potential resulting from wounding and persisting through the stages of healing. This chapter considers the current evidence for the efficacy of treatment using electrical stimulation linked with the endogenous bioelectric evidence summarized in Chapter 3. The use of electrical stimulation as a means of enhancing wound healing is not new. Reports dating back to the seventeenth century record the use of gold-leaf applications to cutaneous lesions associated with smallpox. Although there have been many publications in this research area, especially in more recent years, there appears to be a reluctance to use the therapy in the clinical environment. Given the drive towards evidence-based practice, this appears to be a strange phenomenon.
A chronic wound is any interruption in the continuity of the body’s surface that requires a prolonged time to heal, does not heal or recurs (Ojingwa & Isseroff 2002, Wysocki 1996). In a recent Cochrane review, Kranke et al (2004) concluded that chronic wounds are common and constitute a significant health problem. The true incidence and impact are difficult to assess accurately because of the wide range of disease, the fact that much care is delivered at home, and also because many wound-care products are purchased directly from a variety of sources. Evidence is cited to suggest that 1% of the population of industrialized countries will experience a leg ulcer at some time (Baker et al 1991).
Healthcare costs for chronic wound vary depending on the method of calculation adopted by the authors, but McGuckin et al (2001) suggest that, in the UK, the financial cost of venous disease has been estimated between £294 and £650 million per year; more recently, Kranke et al (2004) suggested that wound care in the UK costs in excess of £1 billion per year. In the United States, costs are estimated between $2.5 and $3 billion, with a loss of 2 million work days per year.
PROBLEMS ASSOCIATED WITH CHRONIC WOUNDS
A relatively small proportion of wounds present with healing problems and many will heal spontaneously without major therapeutic intervention. However, some wound types are notoriously slow to heal, for example chronic venous ulcers and pressure sores. These tend to be lesions of long duration and are often resistant to many forms of treatment. The most commonly encountered wounds in Western medical practice are a consequence of diabetes, arterial and/or venous disease, sustained pressure or as a result of radiotherapy (Kranke et al 2004). They can result in significant medical, social and economic problems for patients, their relatives and the medical professionals involved.
The factors responsible for poor wound healing are legion and beyond the scope of this chapter, but they remain central to the philosophy of the use of electrical stimulation as a modality to enhance healing. Interference with one or more levels of the cascade of events associated with any healing process can lead to inadequate healing and repair responses. Frank and Szeto (1983) summarized the possible general factors as follows:
Chronic wounds behave differently from acute wounds and some authorities have suggested that they are different in a more fundamental way than just their duration. Cutting (2006) suggests that the overall picture found within chronic wound tissue is not simply one of decreased cellular activity but rather disorder, where unregulated cellular functions can be identified. It is argued that to achieve resolution to these chronic problems, a reaction needs to be initiated, then order established before closure can be achieved. The process of wound healing was reviewed in Chapter 4, which together with the bioelectric background information from Chapter 3 provides a platform for this chapter on electrical stimulation for enhancement of wound healing.
REVIEWS
There have been several detailed reviews relating to the use of electrical stimulation in relation to wound healing and wound management. Gardner et al (1999), for example, reported the outcome of a meta-analysis that aimed to quantify the effect of electrical stimulation on chronic wound healing. Fifteen studies were analysed and the average rate of healing per week was calculated for the electrical stimulation and control samples, giving 22% for electrical stimulation samples and 9% for control samples. Further analysis by type of electrical stimulation failed to demonstrate a clear differential between stimulation modes and it was concluded that although electrical stimulation produces a substantial improvement in the healing of chronic wounds, further research is needed to identify which electrical stimulation devices are most effective and which wounds respond best to this treatment.
A very comprehensive and detailed review by Kloth (2005) did not attempt meta-analysis but did consider a raft of evidence from cellular, animal and clinical studies, with a focus on lower limb wounds. Rather than divide the available research up in terms of stimulation mode, Kloth took a clinical problem focused approach which evaluated over 150 papers. Ojingwa and Isseroff (2002) provide a more traditional review considering the various modalities on the basis of their mode of intervention which acts as a useful complement to the Kloth paper.
VARIETY OF APPROACHES
Although numerous studies have looked at the basic and clinical science of wound electrical stimulation, one of the key issues, commented on by nearly all reviewers in the field, is the inhomogeneity of the research. Very few of the cellular, animal or clinical studies have employed the ‘same’ form of stimulation, and thus identifying the essential and common threads in the research remains problematic. Almost every review identifies the need for further research and larger-scale randomized controlled trials (RCTs) in particular (e.g. Cutting 2006, Kloth 2005, Ojingwa & Isseroff 2002).
For the purposes of this chapter, the use of electrical stimulation to enhance or stimulate wound healing has been divided into three main approaches. Each approach described has reported beneficial clinical effects and, at present, the available evidence does not appear to identify a distinct advantage of one approach over another. This chapter sets out to consider the effects of electrical stimulation on chronic skin wounds, particularly chronic venous ulcers, pressure sores and allied lesions. The substantial and growing body of literature concerning electrical stimulation for promoting bone healing and other soft-tissue repair is not discussed in any detail in this chapter; reviews of this additional material can be found in Albert and Wong (1991), Black (1987), Childs (2003), Gardner et al (1999), Rodriguez-Merchan and Forriol (2004) and Watson (2006). At the end of the chapter, a summary of the proposed mechanisms by which stimulation is able to bring about the positive clinical effects is considered.
ELECTRICAL ACTIVITY IN THE SKIN RELATED TO WOUNDS AND HEALING
The key issues associated with tissue batteries, endogenous bioelectric activity and the relationship between these phenomena and tissue injury and repair were considered in some detail in Chapter 3. An unreferenced summary will be provided here for continuity.
CELLULAR STUDIES
A substantial volume of work has been published concerning the effects of electrical stimulation on cell cultures and in-vivoanimal experiments. Numerous studies have demonstrated cellular responses to direct current, often at magnitudes comparable to those found physiologically (see Chapter 3). Whereas it can be argued that laboratory-based cell studies are not directly clinically relevant, it is important to establish that cells of various types are electrically sensitive, demonstrate a change in metabolic activity, and also change their direction and rate of movement in response to electric fields (Cutting 2006, Kloth 2005). The key findings are summarized here.
Fibroblasts
Fibroblasts have been investigated by numerous groups, although not all studies have used human cultures. Using an applied direct current (DC) stimulation, Dunn (1988) demonstrated fibroblast invasion of a collagen matrix placed in a skin wound in the guinea pig. The fibroblast ingrowth and collagen fibre alignment were significantly increased compared to controls. Goldman and Pollack (1996) studied the effect of electrical stimulation on human fibroblasts in vitro. Various current intensities and frequencies were evaluated and a field strength of between 31 and 50mV/mm was found to be effective at 10Hz, but not at 100Hz. The concept of frequency and amplitude windows appears to be supported by this work, and the effective parameters match those identified in the endogenous bioelectric state [see Chapter 1 and Watson (2000)].
Erickson and Nuccitelli (1984) demonstrated fibroblast migration towards the cathode when the cells were exposed to a DC field. The threshold field strength was found to be between 1 and 10mV/mm. In addition to cell migration, they demonstrated changes in cell orientation, the fibroblasts realigning with their long axes perpendicular to the field direction. Field strengths of up to 10 times greater than those necessary to induce fibroblast responses have been measured in vivo. Ross et al (1989) also demonstrated (human) fibroblast alignment when exposed to an electric field with field strengths of 0.1–1.5V/mm (100–1500mV/mm). Further studies that have demonstrated significant beneficial effects on fibroblast motility, proliferation and protein synthesis include Bourguignon and Bourguignon (1987), Canseven and Atalay (1996), Goldman and Pollack (1996) and Thawer and Houghton (2001).
Epidermal cells
When considering wound healing and closure, epidermal cells are of the utmost importance. Epidermal cell orientation and migration have been demonstrated in DC fields (Cooper & Schliwa 1985). The threshold for these effects was in the region of 0.5V/cm (50mV/mm), which represents some 1–4mV per cell diameter. The demonstration by Winter (1964) that epithelial cells migrating from the periphery of an ulcer move in response to the voltage gradient is also pertinent to the discussion on the effects of electrical stimulation for wound healing (Kloth 1995, 2005).
In addition to fibroblasts and epidermal cells, several other cell types have been shown to respond to electrical stimuli; some of the key papers for each main cell type are included in Table 19.1.
Table 19.1 Key papers relating to cell reactivity in response to electrical stimulation
Cell type | References |
---|---|
Fibroblasts | Bourguinon & Bourguinon 1987, Canseven & Atalay 1996, Cheng & Goldman 1998, Goldman & Pollack 1996, Reger et al 1999, Thawer & Houghton 2001 |
Neutrophils | Fukushima et al 1953, Gentzkow & Miller 1991 |
Macrophages | Cho et al 2000, Orida & Feldman 1982 |
Mast cells | Gentzkow 1993, Reich et al 1991, Taskan et al 1997 |
Endothelial cells | Chang et al 1996, Goldman et al 2004, Li et al 2002, Nissen et al 1998, Zhao et al 2004 |
Myofibroblasts | Brown & Gogia 1987, Gabbiani 2003 |
Epidermal cells | Farbould et al 2000, Nishimura et al 1996, Nuccitelli 2003, Pullar et al 2001, Sheridan et al 1996, Zhao et al 1999 |
There has been considerable interest, over the last few years, in the role of angiogenesis in tissue repair (see Chapter 4) and a recent paper by Bai et al (2004) demonstrated a significant beneficial effect of DC stimulation on the angiogenic response in human-derived cells. Furthermore, Zhao et al (2004) demonstrated a similar effect, and identified vascular endothelial growth factor (VEGF) as the mediator of the response, whereas Goldman et al (2004) demonstrated an increase in capillary bed density with electrical stimulation.
Antibacterial effects
The delay of wound healing due to local infection is not doubted (Bowler et al 2001) and healing will inevitably be delayed while the infection persists. Electrical stimulation has been shown to have both bacteriostatic and bacteriocidal effects. It is also suggested that even wounds that do not demonstrate overt signs of infection are very often ‘colonized’ (Hansson 1995), which will almost certainly have an inhibitory effect. It is a well-demonstrated clinical principle that dealing with the contaminated wound is an essential early phase of wound management (Schultz et al 2003) and successful wound closure cannot expected if the infection cannot be managed.
Kincaid and Lavoie (1989) published a series of results highlighting the effects of high-voltage pulsed current (HVPC) on cultured bacterial species in a series of in-vitro experiments. Three commonly isolated bacterial strains were exposed to positive and negative HVPC. All three strains were affected equally by 2 hours of HVPC above 250V. Cathodal (−ve) exposure resulted in bacterial death, whereas at the anode (+ve), toxic electrochemical end-products appeared to be responsible for the bacterial demise. The authors suggested that HVPC could have significant antibacterial effects in the clinical environment. Szuminsky et al (1994) have also identified a definite antimicrobial effect of high-voltage stimulation (monophasic twin peak).
Merriman et al (2004) compared the efficacy of various electrical stimulation modes on bacterial growth in vitro. Microamp stimulation (DC, pulsed DC monophasic and biphasic) and HPVC were compared in their effect on culture plates containing Staphylococcus aureus. The zone of inhibition surrounding each electrode was measured following stimulation and a significant inhibitory effect was shown for continuous DC and HVPC but not for the pulsed monophasic and biphasic modes.
ANIMAL STUDIES
Before considering the effects of electrical stimulation for wound healing in the clinical environment, it is pertinent to summarize the evidence generated from numerous animal experiments. It is difficult to extrapolate directly from this animal work as the wound-healing process is not the same as in humans and, although there are similarities between species, there are no directly equivalent animal-healing models. The experimentation does, nevertheless, provide useful background material for the principles supporting clinical intervention. A more comprehensive review of animal research is found in Kloth (2005) and Ojingwa and Isseroff (2002). The key issues will be identified below to provide a foundation for the clinical studies. In addition, a substantial number of studies have considered the electrical correlates of healing and regeneration in amphibian and vertebrate species, reviewed in a series of papers by Borgens and colleagues (Borgens 1981, 1982, Borgens & McCaig 1989, Borgens et al 1977, 1989). Research evidence will be briefly considered under three themes: collagen content and tensile strength, wound closure and tissue necrosis.
Collagen content and increased wound tensile strength
Several animal wound models include tensile strength measures as a key outcome, although this is not a commonly reported clinical outcome (for obvious reasons). Some doubt has been raised as to the validity of measuring wound healing in terms of tensile strength alone (Forrest 1983) and strength changes combined with collagen content analysis appear to be preferable.
Thawer and Houghton (2001) demonstrated an increase in collagen deposition in diabetic mice wounds. Skin incision work in rabbits is reported by Konikoff (1976), who used bilateral, full-thickness paravertebral incisions with one side receiving DC stimulation and a sham treatment to the contralateral wound. A DC current (20mA) was used and the wounds were tested at 1 week for tensile strength, at which time the treated lesions demons-trated an average 53% increase in tensile strength compared to the sham-treated wounds.
Bach et al (1991) used a rat-skin wound model to compare the effects of DC, AC and sham treatments on wound strength. Neither type of electrical stimulation had a significant effect on wound strength when compared with controls, but both electrical stimulation groups showed significant increases in collagen content in and around the wound compared with the sham group.
The effects of electrical stimulation (through a metallic suture) in abdominal muscle lesions were investigated by Wu et al (1967). Two suture materials were compared for effects: stainless steel and platinum. DC stimulation at 40–400mA was used and it was found that the rabbits with steel sutures gained greater wound strength than those with platinum sutures. The increases in wound strength did not appear to be related to stimulation polarity or intensity, and it was therefore suggested that the benefits of stimulation might be due to electrode products (Fe) rather than the stimulation itself.
There are many animal experiments in this field, and Ojingwa and Isseroff (2002) provide a useful summary, concluding that several forms of stimulation have shown an increase in wound strength, but on balance, the DC stimulation modes appear to have generated the stronger results.
Wound closure
The widely cited experimental work of Alvarez et al (1983) used a pig model to compare the effects of DC stimulation, sham stimulation and no treatment on skin wounds that were evaluated forre-epithelialization and collagen synthesis. It was found that collagen content increased in the DC stimulation group and the epithelial coveringwas more rapid than in the sham and no-treatment groups.
Taskan et al (1997) compared the effect of ultrasound and electrical stimulation in a rat wound model. Four groups were compared: real and sham electrostimulation (ES), and real and sham ultrasound (US). Both real ES and US have beneficial effects but the ES results were found to be superior to those attributed to the US.
Stromberg (1988) reported the results of different electrical stimulation protocols on skin wounds in pigs, measuring wound contraction rates and the open wound area. The stimulation groups received either DC stimulation (35mA unipolar square-wave stimulation for 30 minutes twice a day with the negative electrode at the wound) or an identical electrical stimulation but with the wound electrode polarity reversed every 3 days. The first group, with the consistently negative wound electrode, appeared to gain no benefit from the treatment, with a trend towards a retarded healing process. By contrast, in the group where the stimulating polarity was reversed the wound size decreased to 18% of the original size in 2 weeks, and down to 5% of the original size by the end of 3 weeks of treatment.
Reger et al (1999) compared the efficacy of DC and AC stimulation with a control condition on experimental skin ulcers in a pig model. Interestingly, both the AC and DC stimulation resulted in reduced healing time compared with the control condition, but the DC group showed the most rapid reduction in the wound area and the AC stimulation showed the most rapid reduction in wound volume.
Tissue necrosis
Using a full-thickness skin excision and replacement model in rats, Politis et al (1989) used a necrotic area of skin that resulted under control conditions following this procedure. After 1 week, the size of the necrotic area was compared in a control group and two treatment groups who had received DC stimulation with opposite-polarity currents. The group with the anode to the skin surface and the cathode implanted deep to the wound showed least necrosis (50%), whereas the reverse polarity stimulation group and the sham treatment group both had 80–90% necrosis after the same time period.
Wound healing in pig skin has been extensively studied as it provides an animal model that more closely resembles human skin. Im et al (1990) raised bilateral bipedicle skin flaps, the central portion of which is known to become ischaemic without intervention. This central zone was treated with electrical stimulation (pulsed DC) at 35mA, 128Hz, for 30 minutes twice a day over 9 days. The treatment protocol involved negative stimulation on days 1–3, positive stimulation on days 4–6 and negative stimulation again for days 7–9. The necrotic area in the treated animals was significantly smaller than in the control group.
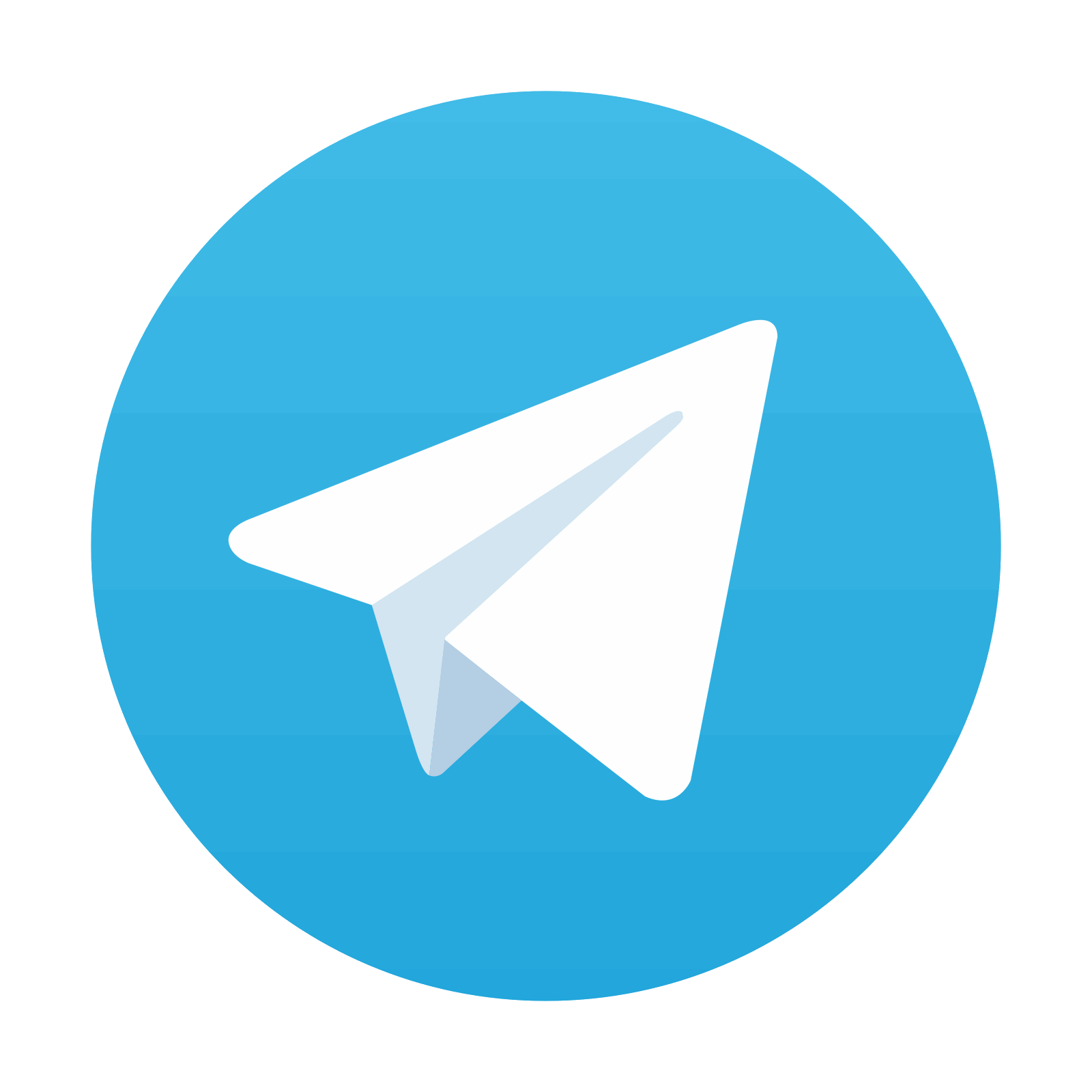
Stay updated, free articles. Join our Telegram channel

Full access? Get Clinical Tree
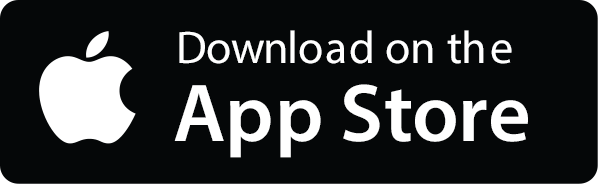
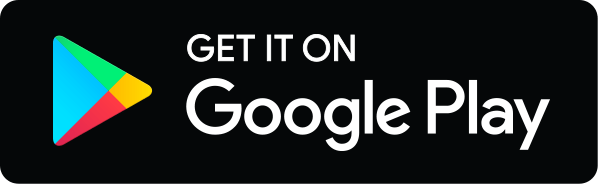