Abstract
Perinatal stroke has become increasingly recognized with the increased application of neuroimaging for neonatal seizures and encephalopathy. The frequency of perinatal stroke is estimated at 1 in 5000 births. Perinatal arterial ischemic stroke (AIS) accounts for 80% of neonatal stroke, while cerebral sinovenous thrombosis (CSVT) accounts for 20%. This chapter will summarize the neuropathology, pathophysiology, clinical presentation, management, and prognosis of perinatal AIS and CSVT. These conditions differ in their risk factors, presentation, management, and prognosis emphasizing the importance of their accurate diagnosis in the newborn infant.
Keywords
Neonatal Stroke, Perinatal Arterial Ischemic Stroke, Cerebral Sinovenous Thrombosis, Hemiparesis, Focal cerebral ischemia
The presentation and etiologies of stroke in the newborn differ from those of children and adults and, in many cases, remain unrecognized. Perinatal ischemic stroke has been defined as “ a group of heterogeneous conditions in which there is a focal disruption of cerebral blood flow secondary to arterial or cerebral venous thrombosis or embolization, between 20 weeks of fetal life through twenty-eighth postnatal day confirmed by neuroimaging or neuropathologic studies. ” Thus the clinical entity of ischemic perinatal stroke includes focal or multifocal ischemic injury to the central nervous system of either arterial or venous etiology that can occur during the prenatal, intrapartum, or postnatal periods. The perinatal period carries the highest risk of stroke in the pediatric age range. Stroke affects about 1 in 1600 to 4000 births, including 1 in 140 preterm births (at or before 34 weeks’ gestation). Approximately 80% of neonatal strokes are ischemic and 20% are cerebral sinovenous thrombosis (CSVT) or hemorrhage. Ischemic strokes may be arterial or venous, and arterial strokes may be due to thromboembolism or in situ thrombosis, as in older children.
Classification-Nomenclature
Multiple subclassifications of perinatal stroke have been proposed and relate to three key factors, that is, the type of vessel affected, the timing, and the clinical presentation. We will use the classification outlined in Table 21.1 . Thus perinatal ischemic strokes are divided into those that are arterial or venous in origin. The former are more common and can be divided according to their time of occurrence. Prenatal/fetal arterial ischemic stroke (AIS) includes intrauterine events from approximately 20 weeks of gestation to the time of delivery. Neonatal AIS includes those exhibiting overt clinical phenomena (usually seizures) in the neonatal period (<28 days), and presumed perinatal AIS includes those that present clinically (usually a focal neurological deficit) at >28 days of life and with an infarct on neuroimaging consistent with a perinatal origin. In the following we discuss in sequence the Neuropathology, Pathogenesis, Clinical Features, Diagnosis, Prognosis and Management, first of Prenatal and Neonatal AIS, and then of CSVT. Presumed perinatal AIS, which presents clinically after the neonatal period, is not discussed further.
Perinatal Arterial Ischemic Stroke (AIS) |
Perinatal Cerebral Sinovenous Thrombosis (CSVT) a |
Perinatal Arterial Ischemic Stroke
Neuropathology
For perinatal AIS, we include the localized areas of necrosis that occur within the distribution of single (or multiple) major cerebral vessel or vessels. Involvement of specific vascular distributions thus is the distinguishing hallmark of this lesion.
Frequency at Autopsy, Cellular Aspects, Topography
The relative frequency of these ischemic lesions at autopsy was emphasized by a neuropathological review of 592 infants examined over a 4-year period. Cerebral infarcts with arterial occlusion were seen in 5.4% of infants. The incidence as a function of gestational age was 0% for infants less than 28 weeks, approximately 5% for those between 28 and 32 weeks, 10% for those between 32 and 37 weeks, and 15% for those between 37 and 40 weeks. Involvement of the middle cerebral artery (MCA) occurred in approximately one half of the affected infants ( Fig. 21.1 ). Multiple smaller vessels were the sites of occlusion in the remaining cases. The predominance of the MCA distribution has been more marked in infants identified in vivo by brain imaging modalities (see later discussion).

The cellular aspects of the neuropathology of these lesions are dominated by necrosis of all cellular elements within specific arterial distributions (i.e., an infarction). The cellular features relate primarily to the time after the insult. After 18 to 24 hours, anoxic neuronal change is apparent by light microscopy; earlier, no change may be detectable. Shortly thereafter, activated cells of the monocyte-macrophage type migrate from vessels and enter the lesion as elongated and pleomorphic microglial cells. These cells become foamy macrophages by 36 to 48 hours. Astrocytic hypertrophy, with the characteristically large, eosinophilic, sail-like cytoplasm of the gemistocytic astrocyte, becomes apparent by 3 to 5 days. Astrocytic proliferation, with prominent staining of glial acidic protein in astrocytic fibers, then forms a dense mat of glial fibrillary processes. This process occurs over weeks to months. Mineralization of neurons, sometimes with more diffuse calcification, may occur. Cavity formation is common and is discussed later.
The topography of infarction in arterial distribution , occurring in the perinatal period and identified in the newborn by brain imaging, is distinctive ( Table 21.2 ). Approximately 75% of lesions are unilateral, and nearly all the unilateral lesions involve the MCA. Of all unilateral MCA infarcts, approximately 65% involve the distribution of the left artery.
TOPOGRAPHY OF INFARCTION | PERCENTAGE OF TOTAL (%) |
---|---|
Laterality | |
Unilateral | 75 |
Bilateral | 25 |
Vascular distribution | |
Left MCA | 55 |
Right MCA | 30 |
Bilateral MCA | 10 |
Other arteries | 5 |
When these focal and multifocal necroses of brain of the prenatal and early postnatal periods are associated with dissolution of tissue and cavity formation, the terms porencephaly, hydranencephaly , and multicystic encephalomalacia are used to describe the lesions. In this discussion, we use the term porencephaly to refer to a single unilateral cavity within the cerebral hemisphere that may or may not communicate with the lateral ventricle ( Fig. 21.2 ). When related to ischemia (rather than intracerebral hemorrhage or infection), porencephaly is the sequela of an infarction with involvement of both cortex and cerebral white matter in the distribution of a single major cerebral vessel. Hydranencephaly refers to massive bilateral lesions, in which most or all of both hemispheres are reduced to cerebrospinal fluid (CSF)-filled sacs. When related to ischemia, hydranencephaly most commonly is the sequela of bilateral cerebral infarction, with involvement of both cortex and cerebral white matter in the distribution of both internal carotid arteries (i.e., the anterior circulation). Because the posterior cerebral artery may have its origin from the anterior circulation in nearly 25% of newborns, the posterior cerebral artery distribution may also be affected. Multicystic encephalomalacia refers to multiple cavitated foci of cerebral necrosis, usually bilateral in distribution. Most examples of these serious and relatively unusual lesions, when related to ischemia, are the sequelae of predominantly cerebral white matter destruction caused by generalized ischemia (i.e., more analogous to severe periventricular leukomalacia than to affection of single or several vessels).

Factors Determining the Propensity to Cavitation
The time period involved in the propensity to cavitation is from approximately the second trimester of gestation to the first postnatal weeks or months. The major factors determining the propensity of human brain to undergo dissolution and cavitation with necrosis during this time are primarily threefold ( Table 21.3 ). The relatively high water content is characteristic of unmyelinated tissue and approaches 90% of wet weight in fetal brain. In contrast, myelinated white matter in the mature human brain is composed of approximately 70% water. The deposition of myelin lipids occurs principally postnatally pari passu with the diminution of water content and the development of tightly packed fiber bundles. This axonal fiber development is reflected in the increasing anisotropic diffusion demonstrated in human brain of 28 to 40 postconceptional weeks by diffusion tensor magnetic resonance imaging (MRI). Thus the first two factors, high water content and relative paucity of tightly packed myelinated fibers, are complementary and result in a tissue that is quite different from the relatively dense, mature cerebrum. Therefore dissolution of tissue is prone to occur with ischemic or other types of necrosis. The additional evolution from dissolution to cavitation relates importantly to the deficient astroglial response to injury. This deficient response is both quantitative and qualitative. As discussed in Chapter 7 , rapid proliferation of glia in human cerebrum does not begin until the last half of gestation and continues for many months postnatally; therefore the number of astrocytes to respond to injury may be relatively less than at later ages. In addition, some deficiency in the astrocytic response per se (i.e., the proliferation and hypertrophy of the cell and the development of glial fibers important for the formation of a tight glial scar) does appear to occur. As a consequence, areas of necrosis become areas of cavitation with deficient glial lining. The glial components in multicystic encephalomalacia are more obvious than in most cases of porencephaly and hydranencephaly, at least in part because of the less complete destruction of entire regions of brain and relative sparing of some glial cells in the former lesion, as opposed to the latter two lesions.
High content of water Relative paucity of tightly packed, myelinated fiber bundles Deficient astroglial response |
The importance of timing of ischemic injury in the development of porencephaly and hydranencephaly is well demonstrated by experiments with fetal monkeys. Animals whose carotid arteries were ligated in the second trimester of gestation (latter part of the second trimester) developed cavitated areas of necrosis, similar to those in hydranencephaly or porencephaly and observable at term. Hydranencephaly resulted especially with early second trimester ligation, and porencephaly resulted from late second trimester ligations. An example of porencephaly produced experimentally in the fetal monkey by unilateral carotid ligation in the latter part of the second trimester is shown in Fig. 21.3 . Vascular ligation before the early to middle second trimester of gestation resulted in cerebral dysgenesis , characterized by gyral abnormalities that were not further defined. A combination of destructive and dysgenetic features occurred with middle second trimester ligations in the monkey. The analogy in the human is the finding of polymicrogyria in the margins of porencephalies of prenatal origin in the human fetus.

Prenatal ultrasonographic studies followed by postnatal imaging and neuropathology clearly demonstrated the human correlates of the studies with monkeys concerning the importance of timing of the insult for the occurrence of cavitation. Porencephaly and hydranencephaly have been documented to develop after insults as early as 20 to 27 weeks, and multicystic encephalomalacia has occurred after insults as early as 30 weeks. Causes of the fetal ischemia in these well-studied cases have included severe maternal hypotension secondary to cardiac failure, anaphylaxis, attempted maternal suicide with butane gas, maternal abdominal trauma, placental and umbilical cord catastrophes, and death of one twin (see later discussion).
Pathogenesis
Although the pathogenetic factors of perinatal stroke related to arterial or venous origin differ in many respects, there is considerable overlap among these two categories. Moreover, in both perinatal arterial and venous stroke, multiple coexisting pathogenetic factors are often involved. Although there is considerable overlap in causes of prenatal and neonatal AIS, we will discuss each entity separately. The principal causes are outlined in Table 21.4 according to the major pathogenetic mechanism.
Focal and multifocal cerebrovascular occlusion-insufficiency |
Vascular abnormality (prenatal) |
Vascular maldevelopment Vasculopathy Familial, proliferative Collagen IV A1 mutation Isoimmune thrombocytopenia Vasospasm Cocaine Vascular distortion |
Embolus (prenatal or neonatal) |
Placental thromboses or tissue fragments, detritus (twin pregnancy with dead co-twin) Involuting fetal vessels (thrombi) Catheterized vessels (thrombi or air) Cardiac: congenital heart disease with right-to-left shunt, patent foramen ovale, atrial myxoma, rhabdomyoma (tuberous sclerosis), |
Thrombus (arterial or venous) (prenatal or neonatal) |
Meningitis with arteritis or phlebitis Trauma Dissection Fibromuscular dysplasia Vascular ligation-manipulation: extracorporeal membrane oxygenation (ECMO) Disseminated intravascular coagulation (e.g., sepsis, twin pregnancy with dead co-twin) Prothrombotic/hypercoagulable, endogenous factors: factor V Leiden mutation, protein C deficiency, protein S deficiency, prothrombin mutation, antithrombin III deficiency, antiphospholipid antibodies, MTHFR mutation, elevated lipoprotein α, elevated factor VIIIc Hypernatremia-dehydration Polycythemia |
Generalized systemic circulatory insufficiency |
Prenatal |
Maternal hypotension or cardiac arrest Maternal trauma (?) |
Neonatal |
Perinatal asphyxia Systemic hypotension or cardiac arrest Congenital heart disease with cardiac failure (exclusive of thromboembolic phenomena) |
Prenatal Arterial Ischemic Stroke
Vascular Abnormalities.
Prenatal stroke has been increasingly identified in utero with advances in fetal imaging. Many of these disorders are related to vascular abnormalities (see Table 21.4 ). A particularly well-documented case of a prenatal focal parenchymal defect with porencephaly, secondary to vascular maldevelopment (see Table 21.4 ), involved the lenticulostriate branches of the MCA and the anterior choroidal arteries. Of additional interest in this case was the occurrence of polymicrogyric cortex in the margins of the porencephalic defect, compatible with a destructive process in the sixth month of gestation. The similarity to the experiments involving second trimester carotid occlusion in fetal monkeys discussed earlier is interesting.
Intrauterine focal or multifocal ischemic injury secondary to vasculopathy (see Table 21.4 ) has been shown in several disorders. A multifocal vascular disorder in association with hydranencephaly in utero was observed in association with a proliferative vasculopathy. The likelihood of a genetic disorder was supported by the occurrence of multiple affected siblings in three families. In two cases, a mitochondrial disorder was suspected because of the finding of low levels of complexes III and IV of the electron transport chain. Microangiopathy related to a mutation in collagen IV A1 appeared to underlie another familial disorder with congenital porencephaly. In a second disorder, neonatal isoimmune thrombocytopenia , intrauterine multifocal cystic lesions have been described and are generally considered to represent a sequela of intracerebral hemorrhage. However, the apparent relation to vascular territories suggests an ischemic lesion (perhaps with secondary hemorrhage). Endothelial injury and even thrombosis have been observed in other types of immune thrombocytopenia.
Vasospasm may underlie the focal cerebral infarctions observed with intrauterine exposure to cocaine (see Chapter 38 ). The vasospasm is postulated to occur secondary to transplacentally acquired cocaine as well as to the surge of catecholamines caused by the systemic and local cerebral effects of cocaine, as detailed in Chapter 38 .
Emboli.
Sources of emboli (see Table 21.4 ) may include placental fragments or clots (e.g., with placental infarcts), or thrombi in fetal vessels. The placenta has been recognized increasingly as a potential source of emboli and cerebral infarction. The principal placental lesions have involved fetal vessels (fetal thrombotic vasculopathy, or fetal vasculitis) and have been associated with intrauterine infection with chorioamnionitis and maternal and, perhaps, fetal coagulopathies. Related placental lesions could underlie in part the increased risk of perinatal stroke with preeclampsia, ovarian-hyperstimulating treatments in women with a history of infertility, and severe intrauterine growth retardation. Emboli from the fetal venous circulation could enter the arterial circulation by passage across the foramen ovale.
Twin-Twin Transfusion Syndrome.
The most dramatic example of prenatal AIS occurs as a component of the twin-twin transfusion syndrome (TTTS). Twin gestation deserves special consideration as a cause of focal and multifocal ischemic brain injury, often related to thromboembolic phenomena ( Tables 21.4 and 21.5 ). As discussed later, generalized as well as focal disturbance of cerebral blood flow is involved in the origin of the neuropathology. Most of the clinically significant brain injury observed with twin gestation occurs in monozygotic monochorionic (i.e., a single placenta) twin gestations. Approximately 65% of twin gestations are dizygotic, diamniotic, dichorionic, and generally not associated with brain injury (except for a modest risk related to prematurity or the rare occurrence of stroke). Approximately 35% of twin gestations are monozygotic. Of the latter, approximately 30% are diamniotic, dichorionic (if the zygote splits early before blastocyst formation). However, approximately 70% of monozygotic twin gestations are diamniotic but monochorionic (when the zygote splits after blastocyst formation). In the latter group, in which each fetus shares the single placenta, the risk of brain injury is considerable (see Table 21.5 ). In this context occur placental vascular anastomoses , especially arteriovenous connections, in which placental tissue perfused by an artery from one fetus is drained by a vein from the other. These vascular connections involve one or more arteries or veins from one fetus inserting into a common placental cotyledon of the other fetus and, thereby, allow for potential abnormal volume distribution between the fetuses. In most situations, the anastomoses are balanced, but in 10% to 20%, they are unbalanced, and the TTTS results. The TTTS is the setting for most of the brain injury associated with twin gestations. Although this brain injury may occur consequent to the fetal death of a co-twin, most such injury occurs without such fetal death.
General features |
Incidence of brain injury overall ≈30%, before modern day treatment Occurrence of brain injury associated with placental vascular anastomoses, particularly artery to vein, with twin-twin transfusion syndrome ; incidence of death or brain injury in untreated severe twin-twin transfusion syndrome >80% Intrauterine death of one twin commonly associated with brain injury in the surviving twin, but most twin gestations with brain injury in one or both twins not complicated by fetal death |
Neuropathology |
Injury in first half of pregnancy |
Porencephaly-microcephaly Polymicrogyria Rarely, anencephaly, exencephaly, encephalocele |
Injury in second half of pregnancy |
Isolated or multiple infarcts Porencephaly with or without polymicrogyria Hydranencephaly Multicystic encephalomalacia Periventricular leukomalacia Rarely, venous thrombosis with hemorrhagic infarct Pathogenesis |
With death of co-twin |
Severe hypotension with cerebral ischemia (hemorrhage into dead fetus) Disseminated intravascular coagulation (thromboplastin material from dead fetus) Thromboembolic (placental material, detritus from dead fetus) |
With or without death of co-twin |
Fetofetal transfusion leading to, in the donor , hypovolemia, hypotension, severe anemia, resulting in oligohydramnios (“stuck twin”), and cerebral hypoxic-ischemic injury, and in the recipient , hypervolemia, polycythemia, hyperviscosity, cardiac failure, resulting in polyhydramnios, premature delivery, and cerebral hypoxic-ischemic injury Mechanical factors: transient disturbance of umbilical blood flow by compression or distortion, placental circulatory stasis with thromboembolism |
The neuropathology of brain injury associated with twin gestations relates particularly to the timing of the injury, as outlined in Table 21.5 . Disturbances in early brain development (e.g., anencephaly and encephalocele) occur only rarely, and such early lesions are considered to be caused by vascular insufficiency. Also of presumed vascular basis are the somewhat more common occurrences of microcephaly with multiple porencephalies and of polymicrogyria. The polymicrogyria may contain features both of a disorder of neuronal migration (nonlayered cortex, heterotopias) and of a postmigrational encephaloclastic process (cortical laminar necrosis). The most characteristic lesions are associated with insults in the second trimester and later and consist of the full range of focal, multifocal, and generalized ischemic lesions (i.e., isolated infarction, porencephaly, hydranencephaly, multicystic encephalomalacia, and periventricular leukomalacia). Concerning the most serious of the ischemic lesions (i.e., hydranencephaly and severe porencephaly), twin gestations accounted for 11% of all cases in one series. In most such cases, a deceased co-twin is identified. (The incidence of deceased co-twin in these most severe cases could be higher because the remnants of such a co-twin are easily missed without careful examination of the placenta. In addition, the prenatal mortality in twins is high [i.e., approximately 20% to 80% of twins detected in the first trimester of gestation were singletons by the time of birth].)
Pathogeneses of the brain injuries in monochorionic twin gestations are best divided into those associated with a dead co-twin and those associated with severe TTTS , but not necessarily fetal demise. Pathogenesis in cases with death of a co-twin includes the following: (1) now considered most common, fetal exsanguination from the surviving to the dead fetus through placental anastomoses, resulting in severe hypotension and cerebral ischemia; (2) thrombosis caused primarily by transfer of thromboplastin material from the dead twin, with disseminated intravascular coagulation resulting; and (3) embolus from the placenta or the dead fetus through the fetal vascular anastomoses (see Table 21.5 ). Pathogenesis in the more common situation of severe TTTS relates principally to the cerebral hemodynamic consequences of TTTS (see Table 21.5 ). Thus the donor fetus experiences hypovolemia, hypotension, and severe anemia, with diminished cerebral blood flow and cerebral hypoxic-ischemic injury the consequence. Because of decreased renal blood flow, oliguria leads to oligohydramnios with the additional potential for umbilical cord disturbance or placental compression. The recipient fetus experiences hypervolemia, polycythemia, hyperviscosity, and cardiac failure and thereby risks generalized and focal (thrombotic) cerebral circulatory insufficiency. Polyhydramnios and resulting premature delivery may contribute to risk. These hemodynamic factors may be complicated by or conceivably superseded by mechanical factors (e.g., disturbances by compression or distortion of umbilical blood flow or of placental flow, with risk of thrombosis; see Table 21.5 ).
Therapy has been directed toward the pregnancies complicated by severe TTTS ( Table 21.6 ). Two fundamental approaches (i.e., serial amnioreduction and fetoscopic laser occlusion of the vascular anastomoses) have been used. Diagnosis of severe TTTS in the second trimester of gestation and institution of therapy shortly thereafter have comprised the usual protocol. The benefit of laser therapy relates to the reduction of the vascular anastomoses (a single amnioreduction to reduce polyhydramnios is carried out at the time of the laser surgery; see Table 21.6 ). In 2004, following the publication of the Eurofetus randomized control trial, laser therapy became the treatment of choice and indeed standard of care for this condition. Combining subsequent published series, perinatal survival of at least one twin after laser therapy was reported in the region of 81% to 88%, and survival of both twins, in 52% to 54% of pregnancies. Most recent directions in the management of TTTS have involved refinements in the prediction of the disease, clarification of the optimum frequency of surveillance, technique of laser ablation, prediction of adverse outcome after treatment, and development of other vascular ablative techniques. Improvements in intrauterine monitoring, including fetal echocardiography and advanced Doppler studies, have guided earlier treatment, which now includes more advanced methods of selective fetoscopic laser photocoagulation with laser photocoagulation of the surface of the placenta. In the largest and most recent follow-up study comparing the standard fetoscopic laser surgery approach to the Solomon technique of more extensive laser photocoagulation, no differences were observed in fetal deaths (16% to 18%), neonatal deaths (4%), and survival with neurodevelopmental impairment (9% to 11%). The Solomon technique is now favored because of the reduction in short-term complications, that is, twin anemia–polycythemia sequence or recurrent TTS.
NONE | AMNIOREDUCTION | LASER | |
---|---|---|---|
Survival at 28 days | 20%–30% | 55% | 75% |
Normal neurological outcome | 20%–30% a | 50%–60% | 85% |
Brain injury | 20%–30% | 15% | 5% |
Vascular Distortion.
Vascular distortion is a potential cause of intrauterine stroke (see Table 21.4 ). We raise this possibility because extremes of neck extension or rotation have the potential to produce impairment of blood flow in the vertebrobasilar system or in the carotid system, respectively. Precedent for such occurrences in older patients is available. The vertebrobasilar system may be particularly vulnerable in the fetus and newborn because of poorly developed ligamentous structures of the upper cervical spine that allow sliding and slipping movements between the atlantooccipital and atlantoaxial articulations. Hyperextension of the head may cause inversion of the atlas through the foramen magnum and impair flow coursing through the vertebral arteries. In a careful postmortem study, cerebral artery compression was shown in three of five infants with neck extension and in three of nine cases of neck rotation.
Intrauterine Trauma.
Intrauterine trauma , secondary to blunt trauma to the maternal abdomen, has resulted in prenatal stroke (see Table 21.4 ). The mechanism of this effect is not clear.
Neonatal Arterial Ischemic Stroke
Pathogenesis of NAIS.
Neonatal arterial ischemic stroke (NAIS) includes those examples of perinatal stroke that present clinically in the neonatal period , usually with seizures (see later). Before discussing the major causes of NAIS, that is, thrombosis or embolus, it should be emphasized that (1) the cause most often is unclear, and (2) multiple maternal, neonatal, and placental risk factors are often present. Some of the more common of these risk factors are shown in Table 21.7 .
SOURCE OF RISK FACTOR | RISK FACTOR |
---|---|
Maternal (prepartum) | Primiparous Infertility Smoking Intrauterine growth retardation Preeclampsia Thrombophilia |
Maternal (peripartum) | Maternal fever Maternal infection Prolonged rupture of membranes Intrapartum complications |
Neonatal | Male Apgar score <7 (5 min) Prolonged resuscitation Hypoglycemia Early-onset sepsis/meningitis Congenital heart disease Vascular abnormality Thrombophilia |
Placenta | Cord complication Chorioamnionitis Chronic villitis with obliterative fetal vasculopathy, thrombotic vasculopathy, small placenta |
Maternal risk factors include primiparous pregnancy, infertility, maternal smoking, intrauterine growth retardation, thrombophilia, and signs of an intrauterine inflammatory state, for example, maternal fever (intrapartum), maternal infection, prolonged rupture of membranes, chorioamnionitis, and placental inflammatory features (see later; Table 21.7 ).
Neonatal risk factors include male gender, early-onset sepsis-meningitis, thrombophilia, congenital heart disease, hypoglycemia, and signs of intrapartum hypoxic-ischemic events (depressed Apgar score, need for resuscitation). In one large study (International Pediatric Stroke Study), among newborns with stroke, 30% received resuscitation, 23% had systemic infection, and less than 20% exhibited prothrombotic or cardiac abnormalities.
Placental factors include chorioamnionitis and, particularly, chronic villitis with obliterative fetal vasculopathy and fetal thrombotic vasculopathy.
The principal pathogenetic mediators of NAIS in these settings are arterial occlusion by thrombosis or embolus or generalized circulatory insufficiency with particular affection of a single vessel (see Table 21.4 ). Some specific examples of these mechanisms follow.
Thrombus, Embolus.
Arterial occlusion by embolus or thrombus (see Table 21.4 ) is a well-documented neonatal cause of focal and multifocal parenchymal defects. a
a References .
Often it has been difficult by clinical imaging or even pathological criteria to distinguish between a thrombotic and embolic process. As noted earlier, the lesions have been observed principally in the regions of the middle (and sometimes anterior) cerebral arteries (see Figs. 21.1 and 21.2 ).Emboli.
Sources of emboli (see Table 21.4 ) may be similar to those in some cases of prenatal stroke (see earlier) and include placental fragments or clots, for example, with placental infarcts; thrombi in involuting fetal vessels, for example, umbilical vein, portal vein, or ductus arteriosus; and thrombi or air in punctured or catheterized vessels. As noted earlier, the placenta has been recognized increasingly as a potential source of emboli and occurrence of cerebral infarction (see earlier). Emboli from the fetal or neonatal venous circulation could enter the arterial circulation by passage across the foramen ovale. In 50% to 90% of normal newborns at rest interatrial left to right shunting is documented readily, and in approximately 60% of healthy newborns studied at 24 hours of age, right-to-left blood flow could be demonstrated across the foramen ovale.
Other sources of emboli include thrombi in the internal carotid artery, portal vein, central venous catheter (thrombus or air), and the heart. Cardiac sources of emboli are relatively uncommon in the newborn period, although infants with congenital heart disease, especially with right to left shunts , may exhibit thromboembolic phenomena of cerebral arteries. Stroke with congenital heart disease can occur in the preoperative, intraoperative, or postoperative periods. The characteristics of the cardiac lesion and the management thereof are important determinants for stroke, with strongest associations with an intracardiac right-to-left shunt, preoperative balloon atrial septostomy, transposition of the great arteries, or single ventricle physiology. In addition, such operative features as duration of bypass, age at surgical correction, and need for reoperation increase the likelihood of occurrence of stroke with congenital heart disease. Diagnostic procedures, including cardiac catheterization and the more recent use of ventricular assist devices as a bridge from extracorporeal membrane oxygenation (ECMO) have been associated with AIS. Rarer causes of cardiac emboli include single cases of atrial myxoma and rhabdomyoma (infant with tuberous sclerosis) that we have seen.
Our suspicion has been that emboli may be more common causes of NAIS than currently recognized. It is noteworthy that cerebral emboli at later ages more commonly affect the left hemisphere (perhaps because of the direct route from the aorta of the left common carotid artery), and the left hemisphere predominance in neonatal MCA stroke is striking (see Table 21.2 ). In addition, transient right-to-left shunting through the ductus arteriosus also might favor the occurrence of left hemispheral emboli. Further support for a relative frequency of embolic infarction in NAIS is the finding by serial Doppler studies of four infants with focal MCA strokes of an ipsilateral decrease in cerebral blood flow velocity at the time of diagnosis of the infarct and a reemergence of blood flow in the ensuing days, consistent with embolic occlusion with subsequent fragmentation and distal migration of embolic fragments.
Thrombi.
Thrombosis may involve arteries or veins (see Table 21.4 ). The most common cause of NAIS related to an abnormality of the artery or vein per se is the vasculitis associated with bacterial meningitis (see Chapter 35 ). Cases of apparent occlusion caused by extremes of neck movement or cranial trauma may relate to vascular injury and resulting thrombosis (see earlier discussion of vascular distortion , concerning prenatal AIS). a
a References .
Neonatal stroke has also been described in relation to carotid artery dissection and to fibromuscular dysplasia affecting multiple intracranial vessels.ECMO may lead to focal cerebral infarction by several mechanisms, including thrombus (see Table 21.4 and Chapter 22 ). In one series of 180 infants subjected to serial brain imaging studies, 16 (9%) exhibited a major brain lesion, 6 of the 16 infants exhibited focal ischemic lesions, and 10 exhibited hemorrhagic lesions. Of the 6 ischemic lesions, 5 were in the right hemisphere, that is, ipsilateral to the carotid artery ligation. Of the 10 hemorrhagic lesions, 7 were in the left hemisphere and only 3 in the right hemisphere; the origin of the hemorrhages in the infants treated with ECMO is discussed in Chapter 22 . A preponderance of right hemispheric ischemic phenomena has been observed by others. The mechanisms for the right hemispheral infarcts include the following: (1) prior ischemic injury, secondary to persistent pulmonary hypertension and the associated systemic hypotension and impaired cerebrovascular autoregulation (secondary to hypoxemia or hypercarbia or both), partially compensated by cerebral vasodilation before institution of carotid ligation and ECMO; (2) ischemia caused by the carotid artery ligation because of insufficient collateral circulation through the circle of Willis; (3) ischemia caused by impaired cerebrovascular autoregulation during ECMO with disproportionate decrease in cerebral blood flow with hypotension in the right hemisphere because of the ligated carotid artery; and (4) thrombosis propagated into the anterior cerebral circulation from the ligated carotid. The occurrence of ischemic lesions in the left hemisphere could relate to emboli emanating from the ECMO circuit or to a steal phenomenon caused by the strong collateral flow from the left cerebral circulation to the right.
Abnormalities of blood volume and coagulability account for most of the remaining cases of thrombosis in NAIS. Hypernatremia-dehydration, which leads to thrombosis probably by a combination of vascular and hypovolemic causes, is among the unusual causes of neonatal thrombosis, affecting either arteries or veins, most commonly veins (see Table 21.4 ). Disseminated intravascular coagulation in association with sepsis is among the most commonly documented causes for thrombosis in many neuropathological series. For example, in the study of 29 newborns with cerebral infarcts and arterial occlusions by Barmada and co-workers, disseminated intravascular coagulation, usually with sepsis, was present in approximately two thirds. Polycythemia rarely has been associated with neonatal ischemic lesions, often complicated by hemorrhage. Polycythemia may lead to arterial or venous thrombosis, and the nature of the pathology in neonatal cases is rarely clear. Impaired cognitive outcome, possibly related to ischemic cerebral phenomena, has been documented in infants with polycythemia and hyperviscosity. Increased cerebrovascular resistance and diminished cerebral blood flow velocity, determined by Doppler studies, also have been observed in polycythemic infants with hyperviscosity, and decreased cerebral blood flow and oxygen delivery have been shown directly by studies of neonatal piglets with hyperviscosity produced by infusion of cryoprecipitate. The hemodynamic factors are probably important in genesis of the overt ischemic lesions associated with polycythemia, but it is necessary to recognize that such lesions are very unusual even among polycythemic infants with neurological symptoms.
Prothrombotic/hypercoagulable endogenous factors and other genetic factors are recognized to be of importance in the genesis of perinatal NAIS ( Tables 21.4 and 21.8 ). Similarly, these factors are frequent contributing factors in sinovenous thrombosis in newborns (see later). The most commonly implicated factors in NAIS have been the factor V Leiden mutation, prothrombin mutation, methylene tetrahydrofolate reductase (MTHFR) deficiency (with resulting hyperhomocysteinemia), protein C deficiency, antithrombin III deficiency, and elevated lipoprotein a . Although present in 30% to 70% of cases of neonatal stroke, these factors are usually combined with other pathogenetic factors that favor thrombosis or embolus, that is, preeclampsia, placental vasculopathy, chorioamnionitis, signs of perinatal asphyxia , sepsis, and congenital heart disease (see later). Indeed, although we classify these disorders here under thrombosis , the likely mechanism of cerebral stroke in these disorders may relate less to thrombosis in situ in cerebral vessels but rather to embolus from thrombi in other sites, such as placenta, involuting fetal vessels, or the heart.
Prothrombotic factors associated with neonatal arterial stroke in 30%–70% of cases Most common factors: factor V Leiden mutation, prothrombin mutation, MTHFR mutation, protein C deficiency, protein S deficiency, antithrombin III deficiency, antiphospholipid antibodies, elevated lipoprotein a , elevated factor VIII c Usually (50%–80%) associated with other pathogenetic factors (i.e., preeclampsia, gestational diabetes, placental vasculopathy, chorioamnionitis, signs of “perinatal asphyxia,” sepsis, congenital heart disease) |
The potential importance of the inherited deficiencies was suggested initially by the detection of a syndrome of multiple thromboses, primarily venous, and particularly associated with hemorrhagic, ischemic skin lesions termed purpura fulminans in newborns with inherited deficiencies principally of protein C or protein S. In general, in these two disorders thromboses were predominantly systemic, although cerebral ischemic lesions in arterial distributions were observed. Several newborns with isolated cerebral ischemic lesions have been described. The mechanism is related to failure of the protein C regulatory system to limit coagulation and augment fibrinolysis. This system is composed of protein C, the pivotal regulatory vitamin K–dependent protein, protein S, a cofactor of activated protein C, and two additional proteins. Of particular interest, protein C and protein S levels are low in the neonatal period, although the activity of protein S is less impaired than that of protein C because of low levels also of a protein that inactivates protein S. Indeed, protein C activity is extremely low in approximately 30% of preterm twin gestations and in approximately 35% of preterm infants with respiratory distress syndrome.
One of the most important of the prothrombotic factors is the factor V Leiden mutation or so-called resistance to activated protein C . In this disorder there is a defect in factor V, a procoagulant molecule, such that the factor cannot be inactivated by activated protein C. This so-called factor V Leiden mutation thus causes a resistance to the normal inactivating property of activated protein C. The result is an excess of the procoagulant, factor V. The newborn may have a particular propensity for thrombosis with the factor V Leiden mutation because, unlike the relatively low levels of protein C in the newborn, factor V levels are similar to adult values. Therefore the capacity of protein C to inactivate the factor V, even without the Leiden mutation, is diminished. This defect has been described in association with neonatal cerebral infarction in many infants. a
a References .
Careful examination of the placenta has led to detection of placental thromboses, a finding suggesting that one mechanism of stroke in this setting is embolus originating in the placenta and reaching the fetal cerebral circulation via the foramen ovale. (Approximately 60% of fetal cardiac output enters brain from this right-to-left shunt.) In approximately 50% of cases, other risk factors, for example, perinatal complications, infection, cardiac disease, or presence of another endogenous prothrombotic factor, were present.Generalized Systemic Circulatory Insufficiency.
An apparently generalized disturbance of systemic circulation with impaired perfusion of brain in the past has been considered to account for a considerable proportion of ischemic cerebral lesions, occurring especially in the peripartum period (see Table 21.4 ). Indeed the frequent occurrence of “prolonged second stage of labor,” “fetal heart rate abnormalities,” “signs of perinatal asphyxia,” placental and cord “complications” and related features have suggested that a generalized circulatory insufficiency could contribute to the pathogenesis of neonatal stroke. a
a References .
However, it is unclear how the strikingly unilateral predominance, in the distribution often of only a single vessel, could occur in the presence of an apparently generalized disturbance of perfusion. The phenomenon has been observed in the fetal and neonatal monkey. Possible explanations include variations in the development of the cerebral vessels or their responses to regulatory effectors . It is noteworthy that the vessels of the anterior circulation, unlike those of the vertebrobasilar circulation, have a dense sympathetic innervation. Thus asphyxia, a potent sympathetic stimulator, may be particularly likely to induce vasoconstriction in the anterior circulation and thereby favor the preponderance of focal cerebral ischemic lesions in the distribution of the MCA. A speculation would be that this innervation may exhibit asymmetries during development and that this developmental feature might explain some of the unilateral lesions seen with this apparently generalized insult. A second major possibility involves the potential of emboli (clot and/or debris) from the involuting placenta to the brain. Thus in fetal sheep rendered hypoxic, the ductus venosus dilates and leads to a marked increase in flow through this vessel. This vascular structure bypasses the liver and provides a direct pathway from the placenta to the brain via the foramen ovale. Whether this phenomenon occurs in the hypoxic human fetus is unknown, although the enhanced flow through the ductus venosus has been shown in human infants with intrauterine growth retardation, placental compromise, and hypoxia.Importance of Multiple Risk Factors.
The importance of multiple risk factors and the nature of these factors in the genesis of perinatal arterial stroke is illustrated by the data shown earlier in Table 21.9 . In this careful study of 37 cases, although data on endogenous prothrombotic factors were not available, the importance of such factors as preeclampsia, chorioamnionitis, prolonged rupture of membranes, and cord abnormality (tight nuchal cord, umbilical cord knot, body cord) is shown (see Table 21.9 ). Moreover, the markedly increased risk related to multiple rather than single risk factors is apparent. The data further illustrate that the pathogenesis of perinatal arterial stroke is very often multifactorial.
A. RISK FACTORS | ODDS RATIO (95% CONFIDENCE INTERVAL) | P VALUE |
---|---|---|
Preeclampsia | 5.3 (1.3–22.0) | .02 |
Oligohydramnios | 5.4 (0.9–31.3) | .06 |
Cord abnormality | 3.6 (1.0–12.7) | .05 |
Primiparity | 2.5 (1.0–6.4) | .05 |
Prolonged rupture of membranes | 3.8 (1.1–12.8) | .03 |
Chorioamnionitis | 3.4 (1.1–10.5) | .03 |
B. NUMBERS OF RISK FACTORS a | PERCENTAGE OF CASES ( N = 37) | ODDS RATIO (95% CONFIDENCE INTERVAL) |
≥1 | 86% | 4.2 (1.4–14.8) |
≥2 | 69% | 6.5 (2.6–16.5) |
≥3 | 60% | 25.3 (7.9–87.1) |
≥4 | 31% | 24.1 (4.7–230.0) |
a Includes risk factors shown in A, and in addition, decreased fetal movement, prolonged second stage of labor, fetal heart rate abnormalities, and history of infertility (treated with ovarian-stimulating drugs, which are prothrombotic).
Clinical Features of NAIS
The clinical hallmark of NAIS in term infants is seizure, especially focal seizure, with onset after 12 hours of life. This timing of seizures is different than in hypoxic-ischemic encephalopathy when seizures occur most often in the first 12 hours. In one careful study, the mean time of seizure onset in NAIS was 27.8 hours versus 5.1 hours in hypoxic-ischemic encephalopathy. In a larger cohort ( n = 79) median postnatal age of seizure onset was 19 hours. Notably, again in contrast to hypoxic-ischemic disease, among infants with stroke only 10% ( n = 8) exhibited encephalopathy, and of these most ( n = 6) had mild encephalopathy.
The clinical presentation of NAIS in preterm infants differs from that in term infants. Indeed, commonly the former infants are asymptomatic and NAIS is identified on routine cranial ultrasonography. This observation raises the question of whether NAIS is more common in premature infants than currently thought because cranial ultrasonography is not as sensitive for detection of NAIS as is MRI (see Chapter 10 ). In a carefully studied series, most preterm infants with NAIS presented with respiratory difficulties or apnea (83%) whereas only 30% presented with seizure.
Diagnosis of NAIS
Diagnosis of NAIS is best considered in terms of the initial assessment and more definitive diagnosis. The latter is accomplished with neuroimaging.
Initial Evaluation
As noted earlier, the most common initial clinical sign of NAIS is a seizure, usually focal, occurring in the first 24 hours of life in an infant without other overt neurological signs. As in any other infant with seizures, laboratory studies should be carried out to rule out seizure etiologies requiring prompt and often specific intervention, such as hypoglycemia, infection, electrolyte or metabolic abnormalities (see Chapter 12 ). Electroencephalography (EEG) is of particular value because focal findings can aid in identification of the location of the stroke. In one large series of infants with stroke or with hypoxic-ischemic encephalopathy, 75% of infants with focal seizures were shown to have stroke.
Neuroimaging
After the initial evaluation suggests NAIS, neuroimaging is performed. The initial assessment often is cranial ultrasonography. MRI is the definitive approach. Computed tomography (CT), formerly used in diagnosis, is not recommended because it is less effective than MRI and subjects the infants to radiation exposure (see later).
Cranial Ultrasonography.
Cranial ultrasonography commonly is used initially. The evolution of the ultrasonographic appearance of acute infarction may consist in the hours after onset of symptoms of no definite abnormality. However, over the ensuing days the evolution of the ultrasonographic appearance of acute infarction consists of echodensity in a vascular distribution, usually that of the MCA ( Fig. 21.4 ). Over the ensuing weeks the echodensity evolves to echolucency, often passing through a stage of heterogeneous appearance termed checkerboard. Nevertheless, the sensitivity of ultrasound for detection of stroke is not optimal and is time-dependent. In two large studies the sensitivity of early ultrasound scan (1 to 3 days) for detection of MRI-proven arterial infarction was 30% to 70%, although scans later (4 to 14 days) detected abnormality in 86%. Nevertheless, because the distribution of the MCA is affected in most cases and the region of the Sylvian fissure is visualized well on coronal ultrasound scans, careful cranial ultrasonography can be useful. Unfortunately, detection of smaller strokes, especially those involving superficial cortical branches, can be problematic with cranial ultrasonography.

Computed Tomography.
Cranial computed tomography (cranial CT) offers higher image resolution than ultrasound but exposes the infant to irradiation. The demonstration by CT of the uncommon occurrence of hemorrhagic transformation of a MCA stroke is shown in Fig. 21.5 . Unfortunately, CT may underestimate the presence and size of the stroke ( Fig. 21.6 ).


Magnetic Resonance Imaging.
MRI not only is the most sensitive technique for detection of neonatal stroke, but the modality provides the best available anatomic resolution ( Figs. 21.7 and 21.8 ; see also Fig. 21.6 ). The specific magnetic resonance (MR) modalities and their value have been effectively reviewed by Lehman and Rivkin. Thus the specific sequences include diffusion-weighted imaging (DWI), T1- and T2-weighted imaging (T1W and T2W), and susceptibility weighted imaging (SWI). Magnetic resonance angiography (MRA) of the head and neck for assessment of craniocervical arteriopathy is useful because MRA can be added readily to the initial MRI evaluation. Vascular imaging has not been studied as much in neonatal stroke as it has in older children and adults. Several reports illustrate the ability of MRA to detect cervicocephalic arterial dissection and asymmetry of cerebrovascular trees in newborn infants with NAIS. MRI is also important because the clinical presentations of neonatal arterial ischemic and sinovenous strokes overlap.


The several MRI sequences outlined above are important not only for careful anatomical identification of NAIS but also for timing and evolution of the injury. DWI is useful for detection of cytotoxic edema and thus is sensitive within hours of the initial stroke. Unlike ischemia/reperfusion injury, as in hypoxic-ischemic encephalopathy, when cytotoxic edema is slow to evolve and is detectable by DWI after 48 hours, in neonatal stroke (as in adult stroke) vascular occlusion leads to cytotoxic edema within hours ( Fig. 21.9 ; see Chapters 10 and 20 ). The DWI abnormality is very prominent at 2 to 4 days after the initial injury. In a detailed study of the evolution of changes on T1W and T2W, Dudink et al. showed that in the first postnatal week, T2W images showed high signal intensity in affected cortical gray matter and white matter and T1W images showed low signal intensity in the involved cortical gray matter. In the ensuing several weeks, cortical gray matter signal intensity was high on T1W imaging and low on T2W imaging. After 1 to 2 months with tissue dissolution, the infarcted area evolved into areas of tissue loss and cysts ( Figs. 21.10–21.12 ).


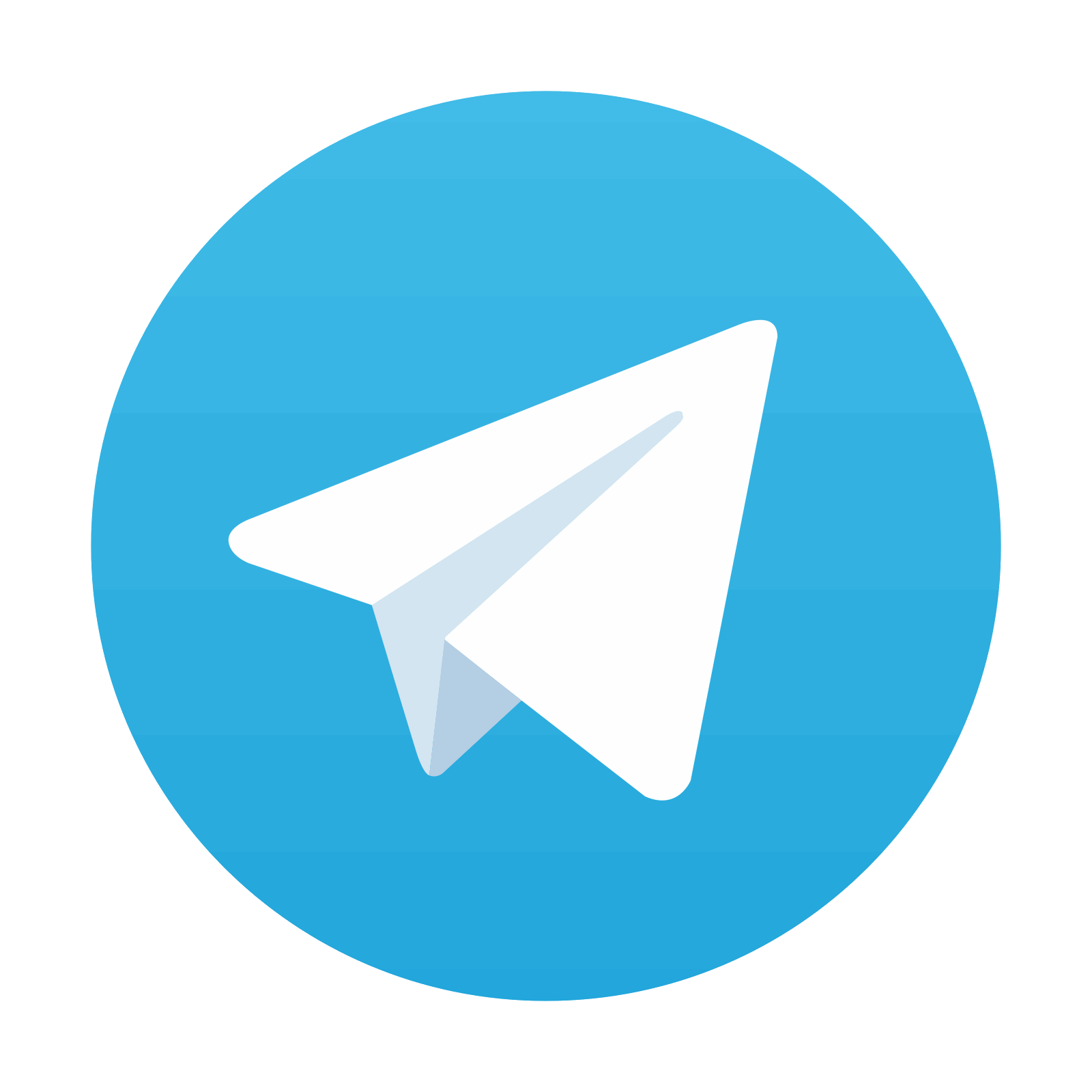
Stay updated, free articles. Join our Telegram channel

Full access? Get Clinical Tree
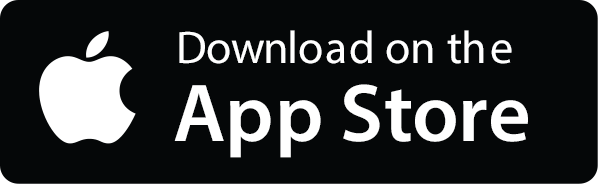
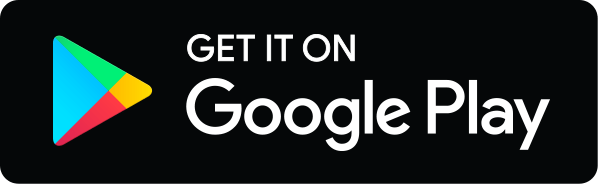
