Subdural Electrode Corticography
William O. Tatum IV
Sanjeet Grewal
Epilepsy is a common and serious neurologic disease affecting more than 50 million people worldwide.1 Electroencephalography (EEG) has been an elemental diagnostic tool for people with epilepsy. Surgery for epilepsy has been the most effective treatment to achieve seizure freedom when patients with focal epilepsy are resistant to antiseizure drugs.2,3 Drug resistance, manifest as recurrent seizures despite optimal antiseizure medication, is the target for epilepsy surgery. Standard EEG using scalp electrodes is the most common noninvasive technique used to evaluate patients with drug-resistant epilepsy and reflects the combined electrophysiologic activity of billions of neurons.4 However, standard scalp EEG only records electrocerebral activity from one-third of the brain. In addition, EEG using scalp electrodes focuses on the asymptomatic interictal period during the recording to identify epileptiform discharges characterizing people with epilepsy.
Invasive EEG is used when localization is unclear using noninvasive means and requires placement of surgically implanted electrodes to record intracranial EEG (iEEG). Invasive EEG monitoring to obtain a focused “look” at targeted areas of the brain is considered when seizures are either nonlocalized or discordant information is present with noninvasive evaluation. Electrodes are directed at sites that have been preselected based upon presumptive localization of the epileptogenic zone. Direct recording of the EEG from the brain is known as electrocorticography (ECoG), or corticography, and may be acquired either in or outside of the operating room. Chronically implanted or indwelling subdural EEG electrodes are used for iEEG monitoring when intracranial seizure recording is required to guide surgical resection/ablation4 and when there is a resection potentially limited by functional brain tissue (Fig. 15.1).
When intracranial electrodes are utilized for invasive EEG recordings, it is important to remember that “you only see where you look.” Additionally, when intracranial electrodes are used to record ECoG in an effort to localize the seizure onset zone, decision-making is coupled with the monitoring results, or “you only find what you see.” Subdural electrodes are the focus of this chapter and represent a common type of iEEG monitoring used in patients with epilepsy to localize the seizure onset zone. This chapter will serve as an adjunct to others on video-EEG monitoring (Chapter 13) and stereotactic (depth) EEG (Chapter 16) to provide readers with a fundamental understanding of the role played by subdural corticography in patients with epilepsy.
STANDARD (SCALP) EEG
Scalp EEG is a prelude to using iEEG. A scalp-based evaluation using video-EEG monitoring, or phase I, is a crucial component in the presurgical evaluation of people with drug-resistant focal epilepsy. The presence of focal interictal epileptiform discharges (IEDs) in the interictal scalp EEG with a concordant abnormality on highresolution brain MRI is often sufficient to predict a seizure-free outcome following resective epilepsy surgery.2,3,4,5 Video-EEG identifies the electroclinical syndrome in people with epilepsy and varies based on the age of the patient, duration of recording, and clinical setting.4 IEDs are associated with epilepsy but vary in morphology and frequency within an individual patient and between patients and do not reliably reflect a single epileptogenic zone. It may be difficult to detect epileptiform activity using scalp EEG when the epileptogenic zone is small (<10 cm2).6 Furthermore, only a small percentage of intracranial IEDs are detected by standard scalp EEG recordings in people with focal epilepsy.4 Inexperienced EEG readers may mistake normal variants for pathologic IEDs.6,7 Most IEDs reflect radially oriented dipoles detected by scalp EEG. Tangential dipoles produced by developmental lesions or surgically altered cortex are more amenable to magnetoencephalography or iEEG.
Potentials recorded on the scalp are volume-conducted through layers of soft tissue, bone, and cerebrospinal fluid and create an unsolvable inverse problem for source localization.7 Scalp EEG often provides an incomplete representation of the entire
brain due to “buried” cortex such as the insula, mesial and midline frontal-parietaloccipital, and orbitofrontal cortex that is deep to the surface electrodes or located within fissures or sulci.8 These areas may elude detection by scalp electrodes despite being present on invasive recording. With standard EEG, localized onsets during seizures recorded by video-EEG monitoring were found to be more common in patients with mesial temporal lobe epilepsy and dorsolateral frontal lobe epilepsy, while lateralized seizure onsets were more commonly seen with neocortical temporal lobe epilepsy.9 One study found ictal EEG to be the best predictor of a seizure-free outcome following temporal lobectomy.10 Nonlocalized and nonlateralized EEG during seizure onset was seen in patients with mesial frontal lobe epilepsy and occipital lobe epilepsy, while falsely localizing and lateralizing seizure onset was found in patients with parietal-occipital lobe seizures.9 Ictal EEG is more likely to detect propagated patterns as opposed to seizure onset activity, leading to false lateralization and even localization.11,12 Furthermore, when there is rapid cortical propagation of electrographic seizures, or when cortical EEG activity is obscured by movement or myogenic artifact, scalp EEG may not provide useful information.12,13 Newer methods including high-density EEG and magnetoencephalography have led to improved methods of source localization.14 High-frequency oscillations and gamma frequency activity detection using scalp EEG are limited by soft tissue filtering and muscle artifact but may serve as a localizing biomarker when iEEG is performed.15,16,17
brain due to “buried” cortex such as the insula, mesial and midline frontal-parietaloccipital, and orbitofrontal cortex that is deep to the surface electrodes or located within fissures or sulci.8 These areas may elude detection by scalp electrodes despite being present on invasive recording. With standard EEG, localized onsets during seizures recorded by video-EEG monitoring were found to be more common in patients with mesial temporal lobe epilepsy and dorsolateral frontal lobe epilepsy, while lateralized seizure onsets were more commonly seen with neocortical temporal lobe epilepsy.9 One study found ictal EEG to be the best predictor of a seizure-free outcome following temporal lobectomy.10 Nonlocalized and nonlateralized EEG during seizure onset was seen in patients with mesial frontal lobe epilepsy and occipital lobe epilepsy, while falsely localizing and lateralizing seizure onset was found in patients with parietal-occipital lobe seizures.9 Ictal EEG is more likely to detect propagated patterns as opposed to seizure onset activity, leading to false lateralization and even localization.11,12 Furthermore, when there is rapid cortical propagation of electrographic seizures, or when cortical EEG activity is obscured by movement or myogenic artifact, scalp EEG may not provide useful information.12,13 Newer methods including high-density EEG and magnetoencephalography have led to improved methods of source localization.14 High-frequency oscillations and gamma frequency activity detection using scalp EEG are limited by soft tissue filtering and muscle artifact but may serve as a localizing biomarker when iEEG is performed.15,16,17
REVIEW
15.1: Limitations of noninvasive EEG recordings include all of the following EXCEPT:
a. Dipole localization may be inaccurate due to volume conduction that broadly disperses the signal generated by the brain.
b. The ictal onset zone and epileptogenic zone may differ from the source of IEDs present on scalp EEG recording.
c. Scalp EEG cannot detect epileptogenic zones smaller than 20 cm2.
d. Basal, intrahemispheric, deep fissures and sulcal cortex are regions not identified well by scalp EEG.
e. Scalp EEG is vulnerable to movement and muscle artifact that may obscure the recording.
INVASIVE EEG
Invasive EEG monitoring is a specialized technique that is crucial for localizing involved portions of the brain during surgical planning for patients with drug-resistant focal epilepsy.18,19 When initial noninvasive evaluation (including seizure semiology, high-resolution brain MRI, PET, interictal and ictal scalp EEG, neuropsychological evaluation, and functional MRI/Wada testing) yields discordant or insufficient information, invasive electrodes are considered.17 All forms of iEEG bypass the filtering and volume conduction produced by the normal anatomy of the scalp and underlying layers of fluid, bone, and soft tissue, which is normally encountered with standard EEG (Fig. 15.2). iEEG alters the morphology, amplitude, and duration of normal and abnormal EEG waveforms, which look markedly different from their usual appearance on standard EEG recording. When the skull is “breached,” prominent beta frequencies and slower theta and delta frequencies become more apparent.4,7 Normal rhythms appear “spikier” and simulate pathologic IEDs. Abnormal ictal patterns may be encountered on iEEG even when standard EEG demonstrates no visible correlate on scalp EEG recorded focal aware seizures.4
The choice of a specific type(s) of intracranial electrode usage should be based upon a hypothesis of the epileptogenic network and balance the advantages and disadvantages of use, method of application, interpretation of recording, and perioperative management.11,18,20 iEEG normally provides brain signals that have an exceptionally high signal-to-noise ratio, less susceptibility to artifacts than scalp EEG, and high spatial and temporal resolution (eg, <1 cm and <1 ms, respectively). Invasive EEG recordings may be obtained either in the operating room at the time of surgical resection or chronically during prolonged video-EEG monitoring during presurgical evaluation on epilepsy monitoring units (Fig. 15.3).
![]() FIGURE 15.3. 3-D coregistration of intracranial EEG with (A) 4 × 5 grids (blue arrow), strip electrodes (black arrow), and (B) 8-contact depths (yellow arrows). (Copyright W.O. Tatum.) |
To characterize the epilepsy syndrome for the purposes of a presurgical evaluation, noninvasive video-EEG monitoring initially confirms the diagnosis of focal epilepsy and establishes the presence of a single epileptogenic zone. Invasive EEG monitoring sessions or phase II evaluations are predicated upon a hypothesis of the electrophysiologic network obtained from a phase I evaluation.18 Results of the prior evaluations should be used to guide the type, placement, and design of the invasive monitoring session. The more refined the hypothesis for lateralization and localization, the greater the likelihood of definitive localization. The disadvantage of a highly focused hypothesis is that some forms of invasive EEG require surgical placement of electrodes in the targeted region, resulting in very restricted spatial sampling (Fig. 15.4). Additionally, the frequency of surgical complications from
invasive electrodes is directly related to the number of electrodes required, so that increased sampling leads to greater risk of complication.21 Invasive EEG may also be used acutely for brief periods of time. Acute iEEG electrodes are typically placed during a neurosurgical intervention (ie, temporal lobectomy, laser ablation, intraoperative lesionectomy) and incur less risk, time, and expense than chronic recordings.
invasive electrodes is directly related to the number of electrodes required, so that increased sampling leads to greater risk of complication.21 Invasive EEG may also be used acutely for brief periods of time. Acute iEEG electrodes are typically placed during a neurosurgical intervention (ie, temporal lobectomy, laser ablation, intraoperative lesionectomy) and incur less risk, time, and expense than chronic recordings.
![]() FIGURE 15.4. A. Stereo-EEG and subdural EEG (left pane) appearance on a radiograph. B. Hemosiderin track from stereo-EEG electrode on axial T1-weighted brain MRI. |
Safety issues for invasive electrode placement (Fig. 15.5) revolve around the techniques of placing and maintaining electrode integrity.22 Supervising EEG technologists should be certified by the American Board of Registration of Electroencephalographic and Evoked Potential Technologists (ABRET, or similar agencies in other countries) for performing EEG with special expertise (preferably certified) in invasive neuromonitoring. Cerebral edema, shifting of midline structures, and infection from subdural electrode placement are the complications of primary concern.22,23 A postoperative CT brain and perioperative antibiotic use are part of a standardized neurosurgical protocol to ensure the absence of intracranial blood accumulation or other unexpected structural changes during intraoperative placement. Clinical symptoms of purulent wound drainage, unrelenting fever, disproportional changes in mental status, or a notable increase in seizure frequency or status epilepticus should be a “red flag” for the possibility of a perioperative structural lesion (ie, subdural hematoma) or CNS infection.
Video-EEG monitoring with invasive electrodes is continued until an adequate number of seizures (usually three or more) are recorded and all extraoperative functional mapping is completed. Interpretation of invasive monitoring is performed by neurophysiologists who are board-eligible or board-certified by the American Board of Clinical Neurophysiology or American Board of Psychiatry and Neurology with at least 1 year of additional training in clinical neurophysiology. Subdural electrodes are explanted in the operating room after seizure monitoring to ensure safe removal but also to facilitate resection of the identified epileptogenic region, which usually takes place immediately following subdural iEEG seizure monitoring.
REVIEW
15.2: Which of the following is TRUE about invasive EEG monitoring?
a. Invasive monitoring can be used to confirm the diagnosis of nonepileptic attacks.
b. Acute invasive EEG reduces some of the risks associated with chronic intracranial recording.
c. Intracranial EEG electrodes need to avoid white matter tracts and eloquent cortex.
d. Ictal EEG recordings using intracranial electrodes appear identical to those obtained from scalp EEG.
e. Phase II evaluations can proceed without prior phase I studies.
View Answer
15.2: b. Acute use of intracranial EEG avoids some of the risks associated with chronic intracranial EEG recording (ie, infection, blood/fluid accumulation, electrode migration). Intracranial monitoring is not appropriate to confirm nonepileptic spells. The electrodes can sample eloquent cortex and white matter without causing damage. Intracranial electrode recordings differ significantly from scalp recordings due to absence of filtering and typically show increased low- and high-frequency activities and “sharpening” of some higher-frequency waves. Phase II studies should never be done without prior phase I studies that may be sufficient to answer the clinical question.
INDICATIONS
Valid reasons for selecting invasive EEG include localizing the epileptogenic zone to a single site within one hemisphere, localizing that site within a single lobe, lateralizing to one side of the brain, or defining the boundaries of the epileptogenic zone and its relationship to eloquent cortex necessary to sustain clinical function. Subdural electrodes are indicated when there is suspicion of a cortically based generator in surgically accessible cortex. Subdural corticography is electrophysiologically similar to other forms of EEG monitoring; however, the disc electrodes used for recording and electrically stimulating the brain represent waveform appearance and dynamics differently from other types of iEEG recording. Nonetheless, potentials recorded by subdural ECoG reflect the same electrocerebral potentials as those recorded by standard EEG recorded on the surface of the scalp.4,7 The iEEG signal is complex and is typically interpreted by visual analysis to extract clinically relevant features during seizure monitoring.8
Any form of invasive monitoring should be reserved for those with nonlocalizing or discordant results after a phase I evaluation.4,8,9,10,11,12 Many patients who undergo subdural corticography, including up to one-third of patients undergoing epilepsy surgery, have a normal high-resolution brain MRI. Subdural corticography plays a pivotal role in these individuals to assist in predicting outcome.11,18,20 However, the use of subdural ECoG may be especially useful when brain regions require direct electrocortical stimulation for functional brain mapping prior to ablation or resection. The presence of a structural lesion is the most powerful predictor of success in resective epilepsy surgery even when invasive recordings are utilized.5 Functional brain mapping with electrical stimulation is a principal indication for subdural corticography to define regions of eloquent (or clinically functional) cortex
and minimize the risk of producing new neurologic deficits from surgery. Features of subdural ECoG may also be used to speculate about the underlying pathology.24 In the presence of a structural abnormality, lesionectomy with resection of the surrounding epileptogenic tissue has yielded the greatest likelihood of a seizure-free outcome. Subdural corticography has been used to successfully guide resection of epileptogenic tissue.25
and minimize the risk of producing new neurologic deficits from surgery. Features of subdural ECoG may also be used to speculate about the underlying pathology.24 In the presence of a structural abnormality, lesionectomy with resection of the surrounding epileptogenic tissue has yielded the greatest likelihood of a seizure-free outcome. Subdural corticography has been used to successfully guide resection of epileptogenic tissue.25
REVIEW
15.3: Which of the following is an inappropriate indication for subdural ECoG?
a. Drug-resistant focal impaired awareness seizures with bilateral independent left and right temporal seizure onset.
b. The seizure onset zone localizes to the left frontotemporal lobe, and semiology includes sudden onset of speech arrest.
c. The seizure onset is left temporal but MRI shows a right temporal cavernous vascular malformation.
d. Semiology suggests temporal lobe seizure onset but standard ictal EEG is nonlocalized.
e. Right temporal epileptiform discharges and focal seizures recorded on scalp-based video-EEG monitoring and right hippocampal sclerosis on brain MRI.
View Answer
15.3: e. Concordant MRI lesion and noninvasive video-EEG monitoring results (especially in the nondominant temporal lobe) predict a good outcome independent of intracranial EEG. Such patients are sometimes known as “skip candidates” since they can skip intracranial monitoring.
SUBDURAL INTRACRANIAL EPILEPSY MONITORING
Advantages
Subdural corticography has a number of advantages over standard EEG, primarily because it records local field potentials directly from the brain and produces greater signal fidelity.26 Subdural corticography can access areas of the cerebral cortex that are not discoverable with scalp EEG. Like focal seizures recorded using scalp EEG, seizures recorded with subdural electrodes display the same electrophysiologic potentials with a wide variety of expression patterns affecting frequency, amplitude, distribution, rhythmicity, and evolution. Advantages of subdural EGoG lie in removing the filtering effect of the intervening skull and soft tissues that may otherwise dampen or diffuse the surface EEG. Several manufacturers produce thin (<0.6 mm) electrode sheets (grids) and serial electrodes (strips) that are embedded in a flexible, clear, polyurethane (Silastic) material, with or without numbered electrode positions (see Fig. 15.5). The strip and grid electrodes are composed of stainless steel or platinum electrodes that are <5 mm in diameter and separated by 0.5-1 cm interelectrode distances. Common strip electrodes are composed of 2, 4, 6, and 8 electrodes, and 4 × 4, 6 × 6, and 8 × 8 grids are routinely available. These strips are MRI-compatible and create fewer artifacts due to the type of metal used. In addition, subdural grids can be trimmed and tailored in- or outside the OR, to accommodate the individual craniotomy site or array that is needed. The electrodes are arranged according to a particular question that necessitates invasive recordings. For example, when a known temporal localization is evident on noninvasive recording but lateralization is unclear, bilateral strip (or depth) electrode placement can answer the question of lateralization (Fig. 15.6). Those with unclear localization despite lateralization (as in the case of a focal structural lesion) can be approached with grid electrodes (see Fig. 15.2). Each individual electrode has a single insulated wire that joins those from other electrodes to form a cable from the strip (or grid) of electrodes to exit the scalp via a second surgical “stab” site that minimizes infection and cerebrospinal fluid leaks. During seizure monitoring, movement of the electrodes may occur altering the localizing information relative to the brain. To minimize this, sutures are placed to secure the electrode to the dura, especially in the cases of large grid electrodes. Post-op brain CT and skull films (see Figs. 15.2, 15.4, and 15.6A) have been used to allow for independent interpretation of the electrode placement relative to the surrounding anatomy. Postoperative high-resolution brain MRI may permit electrode colocalization with three-dimensional T1-weighted imaging to more closely define cortical coverage when needed.
Subdural strips are placed through either burr holes or craniotomies, though grid placement requires craniotomy. Subdural electrodes offer the advantage of recording neocortical iEEG and also delivering electrical stimulation when needed for functional mapping. Subdural electrodes record EEG from broad regions and offer a more versatile recording technique for neocortex compared to depth electrode recording (Table 15.1). They are ideal for interhemispheric and basal cortex where a large or ill-defined epileptogenic zone is suspected or encountered.27 Patients with localization-related epilepsy and a focal neocortical structural lesion on high-resolution brain MRI (such as a tumor, cortical dysplasia, encephalomalacia, etc.) are ideal candidates for subdural EEG recording with either a grid or strips placed directly over the lesion to record seizure onset or define the surrounding cortex by electrical stimulation.28 Characteristic EEG patterns may reflect pathology, with interictal paroxysmal fast activity and runs of repetitive spikes correlating with the ictal onset zone in patients with isolated focal cortical dysplasia.20,29,30
The morbidity of strip electrodes is low in experienced Level 4 epilepsy centers. Less than 1% of people with epilepsy have complications involving infection and hemorrhage.28 Strips can be removed at the bedside with local anesthesia, though they are often removed in the OR at completion of the phase II recording
session when resective surgery is performed. Grids are placed and removed through craniotomy sites in the operating room. Perioperative prophylactic antibiotics are used for 1-2 days and longer if cultured craniectomy bone demonstrates colonization. Perioperative steroids are required when large or multiple subdural grids are used. We prefer subdural monitoring techniques over stereo-EEG when there is concern about mapping eloquent cortex or when we need to define the margins of the epileptogenic zone for potential resection, while stereo-EEG is preferred for cases of presumed limbic network epilepsy where sampling of the deep structures is critically important. However, combined use of different types of electrodes31 and sequential use31 are successful clinical strategies that may be utilized depending upon the presurgical hypothesis for localization.
session when resective surgery is performed. Grids are placed and removed through craniotomy sites in the operating room. Perioperative prophylactic antibiotics are used for 1-2 days and longer if cultured craniectomy bone demonstrates colonization. Perioperative steroids are required when large or multiple subdural grids are used. We prefer subdural monitoring techniques over stereo-EEG when there is concern about mapping eloquent cortex or when we need to define the margins of the epileptogenic zone for potential resection, while stereo-EEG is preferred for cases of presumed limbic network epilepsy where sampling of the deep structures is critically important. However, combined use of different types of electrodes31 and sequential use31 are successful clinical strategies that may be utilized depending upon the presurgical hypothesis for localization.
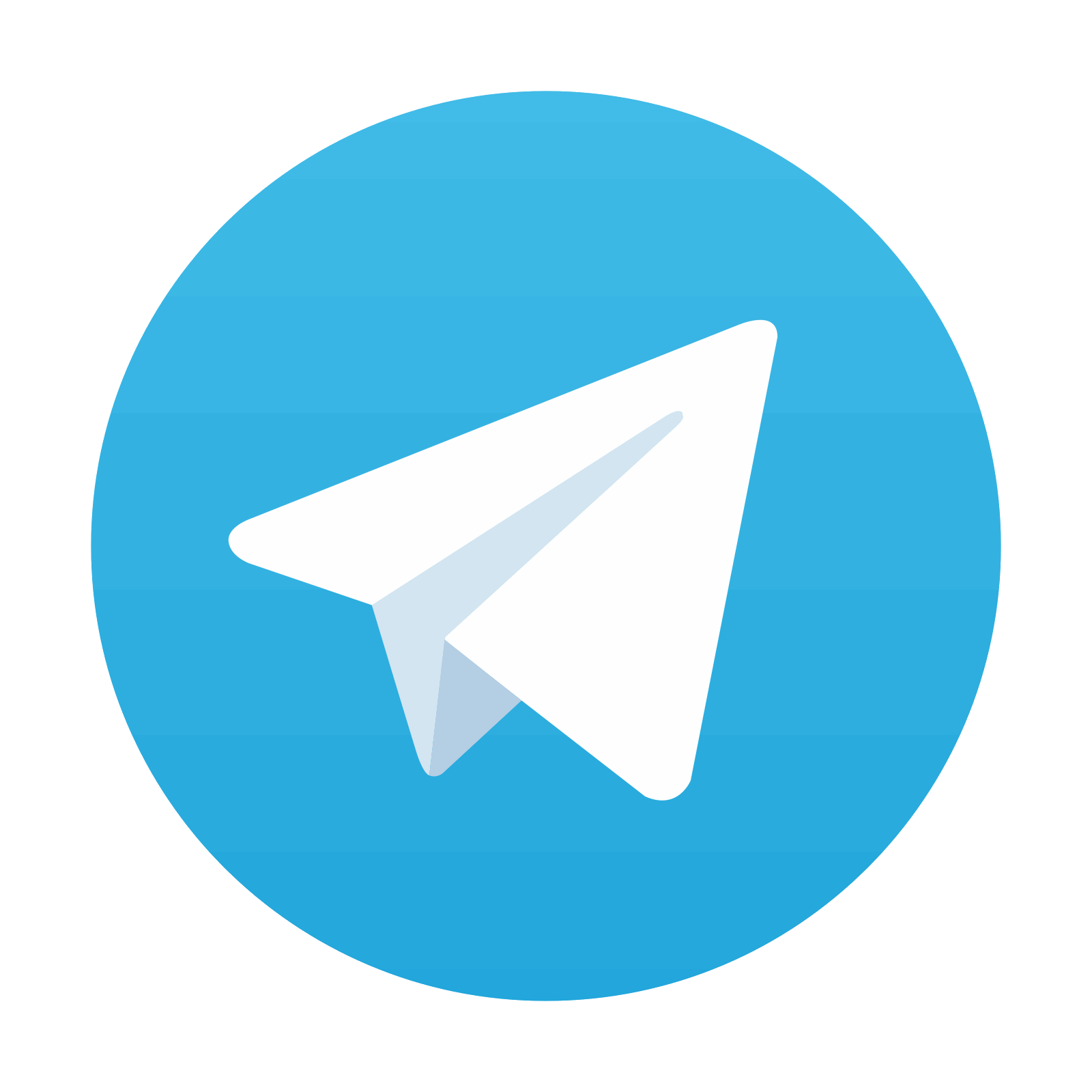
Stay updated, free articles. Join our Telegram channel

Full access? Get Clinical Tree
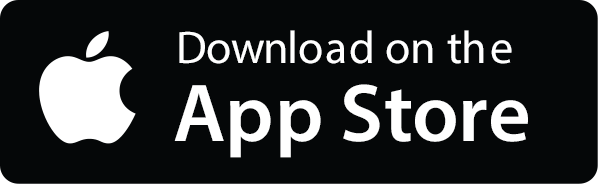
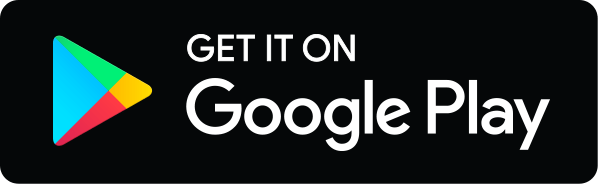
