Surgical Strategies and the Pursuit of Minimalism
The past few decades have seen successive quantum leaps forward in the surgical arena known as neurosurgery. Although the basic principles (maximum outcome, minimal morbidity) remain unchanged from the time of Harvey Cushing and Walter Dandy, the application and outcome of these surgical approaches continue to improve on a regular basis.
With the introduction of the microscope into the operating room by Nylén in 1921, momentum was gained throughout the 1950s with multiple refinements that eventually led to Kurze′s application of the microscope to neurosurgery in 1957. The utility of the microscope to neurosurgery, well demonstrated by Donaghy et al1 in the 1960s, brought incredible illumination and magnification to previously unseen tissues. Not only did this permit more precise dissection with less retraction, but also it ushered in the eventual digital era, with remote visualization now possible for purposes of teaching as well as enabling distant intervention. With these newfound advantages came a fresh set of challenges; eye–hand coordination became a paramount skill. These advantages also led to a better understanding and recognition of anatomic boundaries. This in turn led to the development of a whole new set of surgical tools that were necessary to facilitate work within the narrow confines of smaller exposures and yet permit removal of tissues with various densities and consistencies. Subsequently, we witnessed the refinement of the laser (CO2, yttrium-aluminum-garnet [YAG}, etc.)2,3 as well as development of the ultrasonic aspirator (i.e., Cavitron ultrasonic surgical aspirator [CUSA])4,5 and a variety of long-handled bayoneted instruments.
By the 1980s, endoscopic approaches and associated tools were being promoted in multiple and novel manners. Use of the endoscope had been pioneered by Dandy6 in the late 1920s for choroid plexus obliteration in the setting of hydrocephalus and was gradually incorporated in removing intraventricular tumors; it also facilitated endoscopic third ventriculostomies. Today, this tool has been a critical part of the neurosurgeon′s armamentarium in approaching more than ventricular lesions. The endoscope is used routinely for carpal tunnel releases (as well as other peripheral nerves),7 tethered cord release, Chiari decompression, craniofacial surgery,8 pituitary tumor resection,9 removal of skull base lesions (e.g., chordomas),10 and adequate visualization of surgical fields (tumor, vascular, etc.), as well as a mainstay in the treatment of hydrocephalus and ventricular lesions.11–13 Contributing to this explosion of interest and utilization has been the additional refinement of the endoscopes themselves as well as their associated instruments. Ultimately, endoscopic surgery is a further extension of “the most surgery for the least cost.”
Whereas utilization of the surgical microscope has allowed the neurosurgeon to clearly see different tissue planes as well as technically maneuver in tight spaces, another increasingly important challenge has been determining the precise location within the brain at any point in time, ultimately improving overall outcomes. Thus the era of real (or near-time) intraoperative imaging in conjunction with three-dimensional (3D) localization in the brain (and even spine) was born.
The advent of frame-based targeting (Leks-Wells, Brown, Roberts, Wells [BRT])14–16 in the 1980s enabled precise targeting of small or deep lesions that were previously too risky to approach by conventional means. This contributed to an explosion of stereotactic biopsies (particularly at the advent of the HIV era) with an accuracy of 1 to 2 mm and a morbidity of 2 to 5%,17–19 in addition to more precise localization of deep-seated lesions.
The greatest difficulty, however, lay in the cumbersome application of the rigid fixation system and inherent difficulties in setting up the target coordinates as well as tissue acquisition. Subsequently, frameless-based systems were developed in the 1990s, and they used both optical as well as electromagnetic fields for accurate 3D localization in space ( Fig. 9.1 ). With each subsequent generational advance in proffered systems came new levels of accuracy as well as ease of use and integration with real-time imaging.
From the earliest days of neurosurgical intervention, surgeons have been faced with achieving the difficult balance between gross total tumor resection and maintaining an intact neurologic status.
Currently there is an increasing body of evidence demonstrating improved outcomes correlating to degree of resection,20–23 and this in turn has contributed to the inexorable push to minimize exposure and risks while concomitantly maximizing resection and outcomes. Over time, a variety of tools as well as approaches have been developed to offer greater accuracy with increasingly minimal exposure.
Subsequently, endoscopic and minimalistic techniques advanced from the Dandy era to the current arena with the introduction of a multitude of new devices, ultimately leading to greater efficacy in the surgical field. Over time, improved instrumentation, coupled with enhanced illumination, has led to better visualization for microscopic dissection and has subsequently enabled the contemporary neurosurgeon to approach the surgical target utilizing anatomic boundaries previously only seen on postmortem dissection. This is best exemplified by the coordination between real-time surgical exposure and the pre-operative acquisition of diffusion tensor imaging (DTI) studies demonstrating inherent white matter tracts/pathways and their relationship to surrounding normal as well as abnormal tissue (particularly tumors) ( Fig. 9.2 ).

Present-day navigational systems enable the precise localization of particular areas of interest within the brain, and not only permit accurate discovery of such areas but also may facilitate avoiding critical structures. With the improved accuracy and ease of use of contemporary frameless navigational systems, the role of up-to-date conformational imaging data has become more important.
Radiographic information acquired before the start of surgery has inherent inaccuracies built in from the start. One has to assume that the anatomic parameters used for navigation have not changed since obtaining the study and, perhaps more importantly, will not be altered by the actual procedure itself. Whereas “old” information is being used to guide navigational parameters used in localization of lesions and surrounding tissues, this same information is part of the overall assessment for establishing an adequate boundary when completing the tumor resection. There are many areas whereby this information is less than accurate. Initially, the brain′s conformation may have changed considerably from the time of the magnetic resonance imaging (MRI)/computed tomography (CT) to the time the dissection is performed. This may be problematic for localization of small lesions or in scenarios where millimeters may make the difference between success and medical misadventure. Furthermore, the brain commonly changes configuration, relatively quickly, when tumors are debulked or when cystic compartments are fenestrated. For the same reasons, this may lead to considerable inaccuracy in visualizing the underlying brain. Finally, although the surgeon may be able to visualize as well as intuit the degree of tumor resection, there may still be surprising inaccuracies that will be seen on postoperative radiological studies.


In the context of the above issues, the advent of “real-time” or “near-time” radiographic imaging during tumor resection has represented a quantum leap forward in this arena. Present-day digitalization of radiographic data has allowed the manipulation of countless preoperative studies with data acquired at the time of surgery for greater accuracy and safety. This has enabled the preoperative functional MRI (fMRI) ( Fig. 9.3 ) or DTI studies to be used in the surgical planning and eventual exposure (unlikely to be acquired intraoperatively due to the long scan acquisition times) and may be extrapolated to include angiographic information. Yet the greatest advantage of real/near-time information lies not only in improved surgical accuracy but also perhaps in accessing the extent of surgery and whether the surgical objectives have been met. The degree of tumor resection is commonly challenging to fully appreciate, particularly in areas of limited surgical exposure as well as infiltrative lesions. The advantages of knowing the actual extent of resection as well as determining the presence of any hematoma or shift in adjacent anatomy cannot be overstated. It is not uncommon to find surprising amounts of residual tumor, and on occasion one may be gratified to know that the surgery is now complete. Acquisition of a final image before leaving the operating room may also preclude the need for subsequent staging studies in the immediate postoperative period, reducing the need for further anesthesia and risk.
Intraoperative Imaging
To date, the principal intraoperative radiographic tools are ultrasound, CT, and MRI. They may be used singly or in conjunction with one another.
Ultrasound has been a standard tool in the neurosurgeon′s operating room for a considerable period of time, and has demonstrated its efficacy in multiple surgical arenas beyond tumor surgery. Its low cost (relative), speed, ease of use, and provision of immediate “real-time” data, allowing visualization of motion, have enabled ultrasound to become an invaluable tool for the surgeon. Current systems provide remarkable high-definition images as well as Doppler studies to assess vascular structures and flow, and have the capability of digitizing their images to allow for simultaneous incorporation into CT or MRI intra-operative studies. The ability to merge/blend multiple studies effortlessly increases the accuracy and amount of information available to the surgeon. Most importantly, the dual use of the quick and simultaneous information provided by the ultrasound in conjunction with an intraoperative MRI/CT removes the blind period between MRI images (which can take 30 to 60 minutes to acquire). Thus, we have the difference between real- and near-time data. The only disadvantage, aside from the high cost of $100,000, may be the unfamiliarity of orthogonal views to the “non-expert” surgeon performing as a radiologist. Nonetheless, the learning curve is reasonable in this setting and this becomes even less of an issue when the ultrasound image is merged with the intraoperative CT or MRI picture.
Although the obvious advantages of a near-simultaneous CT or MRI may be intuitive, it should also be clear from the previous discussion that the inherent value of 3D localization and accurate assessment of the brain before and after tumor re-section contributes to the unparalleled importance of this tool. This is not meant to imply that all surgical resections of tumors benefit from intraoperative imaging, but in certain settings this tool may allow for safer and more accurate resections.24–26 Considerable work has accumulated over the past decade demonstrating the efficacy and excellent return on investment of such systems.27–29 This has perhaps been best seen for surgery of invasive gliomas, pituitary lesions, spinal cord tumors, and any other type of surgical problem in which it is critical to appreciate actual anatomic landmarks before reaching a decision-making end point about the need for further resection.
There are two intraoperative CTs in use today. Both systems permit accurate definition of surrounding tissues and may be helpful for navigation. The CereTom™ system (NeuroLogical Corp; Danvers, MA) ( Fig. 9.4 ) employs a portable CT unit that is commonly used in the intensive care unit but can easily be moved to the operating room (or any other space). This machine provides a simple and rapid way to obtain a near-immediate CT scan that may be used to check anatomic landmarks, the degree of tumor resection, and the placement of ventricular (or cystic) catheters, as well as to perform as part of any navigational localization needed. The advantages of this system include speed, ease of use, excellent definition, and reasonable cost (relative to other approaches). Unfortunately, one must consider the downside of additional radiation to the patient as well as limited definition of tissues (CT versus MRI), in addition to the current challenges of the machine′s small bore when used in conjunction with a Mayfield pin-fixated head holder. The arrival of newer models with a larger bore to accommodate the whole body (as well as Mayfield head holders) is imminent. Advocates of the above system claim the cost–benefit ratio of a $300,000 machine versus a $2 million to $8 million MRI speaks for itself. Nevertheless, this will depend on the need for high definition as well as incorporated fMRI/DTI. An alternative approach to utilization of CT in the operating room is the O-arm™ (Medtronic; Minneapolis, MN) commonly employed for spinal instrumentation. The size and scope of this instrument make this a less likely tool for intracranial surgery, as does the lack of a marketable cranial algorithm requiring bone/intermediate windows for visualization of the brain (less than desirable).

Although MRI has been used in the operating room over the past decade, it has clearly undergone significant modifications over time. There are presently two types of systems in use: low field versus high field. Low-field magnets (Polestar™, Medtronic) employ magnetic field strengths of 0.15 tesla (T) in a compact, portable instrument that is part of the surgeon′s armamentarium ( Fig. 9.5 ). The machine is commonly rolled into the operating room and is raised to the level of the patient′s head for acquisition of the MRI image and then lowered to remain out of the way during any surgical intervention. The machine can also be rolled out of the way, although this requires re-registration of the patient and the data when it is returned to use (essentially taking 10 to 15 minutes of additional time).
The lower magnetic field and its much smaller Gauss effect enable the use of standard surgical instrumentation as well as offering a subsequently safer footprint. In addition to the general simplicity of the portable unit, including the lack of need for a radiologist/technologist/repair technician, the costs are 20 to 25% of the standard high-field units. On the other hand, the small-bore size of the magnet entails a steep learning curve in how to maximize patient positioning to be able to obtain a desired and helpful intraoperative image with a limited pin-fixation system. Although the manufacturers have made considerable progress in simplifying and improving original fixation systems, one may still encounter challenges at the time of initial setup and patient positioning. Another drawback is the low-field magnetic strength that not only produces poorer resolution imaging but also is prone to significant image degradation from spurious noise (electronic) within the immediate physical environment. For the most part, the quality of images after adjustment of all extraneous factors is sufficient to make accurate surgical decisions (especially if one uses higher resolution pre-operative imaging merged with low-field intraoperative images) ( Fig. 9.6 ).
Higher field intraoperative magnets (1.5 or 3 T) obviously provide improved resolution on the order of routine MRI, studies but this comes with a steep cost. Aside from the $8 million to $10 million initial outlay, such instruments require the assistance of an MRI technologist, radiologist, and, not infrequently, a repair technician. Due to the high cost of amortization, it is often necessary to utilize this instrument for nonsurgical means (diagnostic studies). Within the operating room environment, this requires having mechanisms in place to maintain a sterile environment as well as safeguard the patients from the highfield safety concerns. Construction of MRI surgical suites is another variable, and these suites are set up either to maintain the surgical patient in the same room as the magnet or to move the patient into an adjacent room (which can also be used for diagnostic studies when not employed for a surgical case) for the MRI scan.


Considerable caution must be taken to avoid any potential projectiles from gaining entrance into the MRI suite as well as safeguarding the still-anesthetized patient during the 10 to 20 minutes it takes to transport the patient 20 to 30 feet from the anesthesiologist′s operating room station. The distinct advantages lie in the superior quality of the MRI images and in the ease of positioning the patient at the outset. Although it is considerably easier for the surgeon, the use of high-field MRI has significant costs for the entire team and adds a necessary level of scrutiny for safety concerns. Nonetheless, this is now becoming a more common instrument within busy and sophisticated operating theaters. Although there is accumulating data on the clinical and financial efficacy of the intraoperative MRI, it remains to be seen where this surgical tool will be most cost-effective in the long-run. Undoubtedly, there will be bigger and more expensive machines as part of the technological push into medicine, perhaps leading to the all-in-one (ultrasound/CT/MRI) machine or the “hand-held medical tricorder” popularized in the Star Trek series. There is currently a $10 million prize being offered by Qualcomm (Bridgewater, NJ) and the X Prize Foundation (Playa Vista, CA) for the first mobile tool that can diagnose patients better than a panel of qualified specialists.
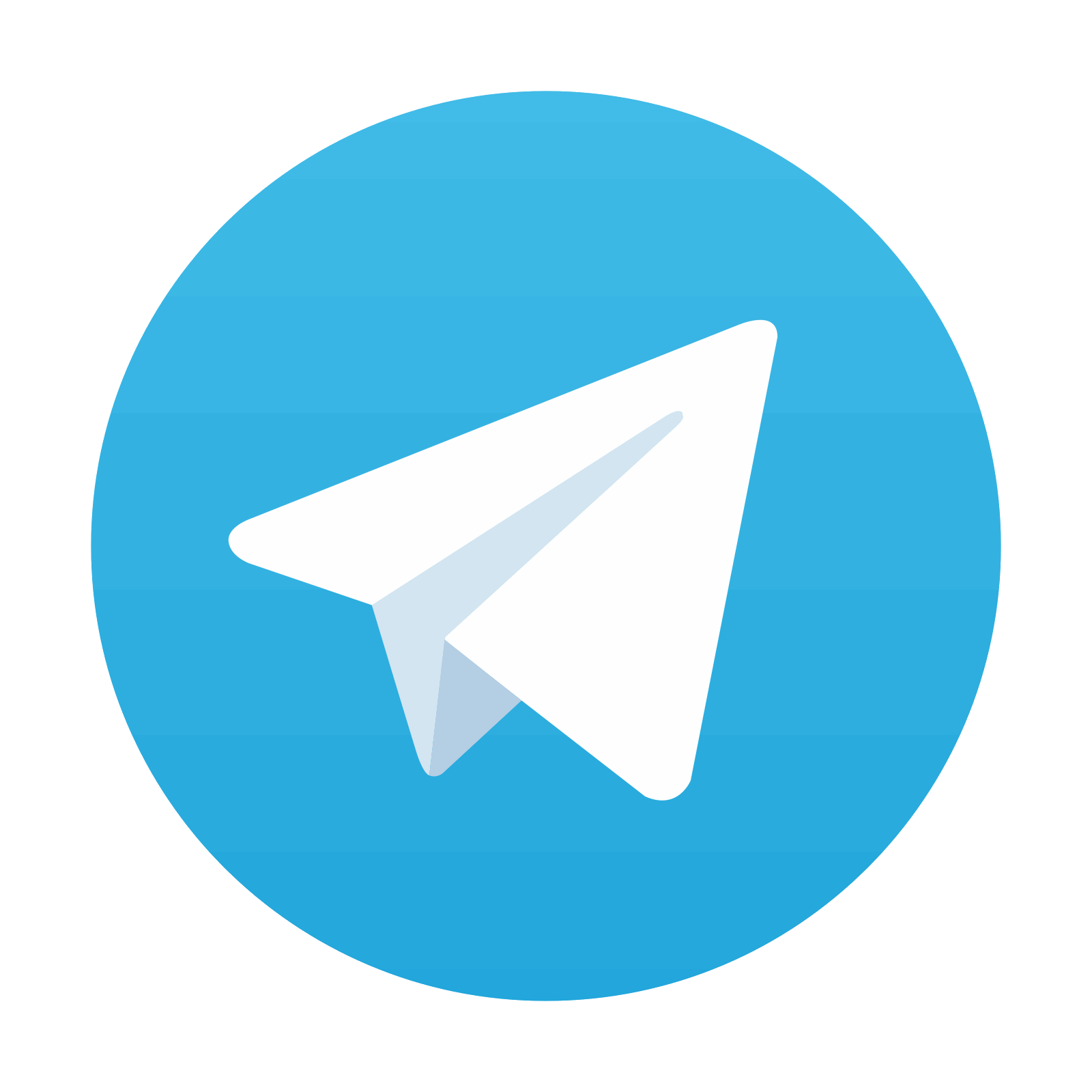
Stay updated, free articles. Join our Telegram channel

Full access? Get Clinical Tree
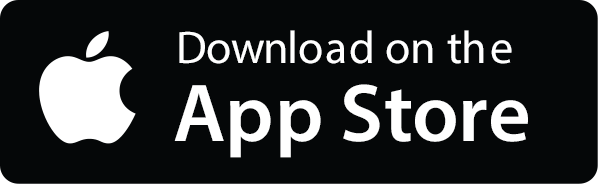
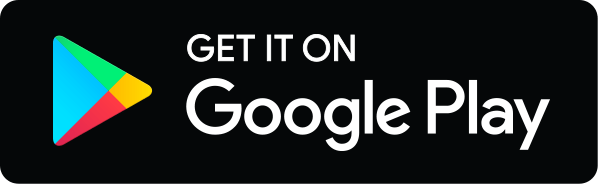