Microsurgical resection remains the treatment of choice for more than half of all patients with arteriovenous malformations (AVMs). It reduces the treatment window to a span of a few weeks and is curative. Careful patient selection, meticulous surgical planning, and painstaking technical execution of surgery are typically rewarded with excellent outcomes. For dural arteriovenous fistulas (DAVFs), microsurgical obliteration is often reserved for cases in which endovascular therapy either cannot be pursued or fails. When performed, however, microsurgical obliteration of DAVFs is associated with excellent outcomes as well. This article reviews the current state of microsurgical treatment of AVMs and DAVFs.
Microsurgical resection remains the treatment of choice for more than half of all patients with arteriovenous malformations (AVMs). It compresses the treatment window into a span of a few weeks and is curative. Careful patient selection, meticulous surgical planning, and painstaking technical execution of the surgery are typically rewarded with excellent outcomes. The techniques for AVM resection have improved steadily over the past 80 years since the first report of the complete resection of an AVM. Five milestones in this surgical field have been: (1) the introduction of cerebral angiography in 1927 by Antonio Caetano de Abreu Freire Egas Moniz from Portugal, (2) the first reported complete resection of a posterior fossa AVM in 1932 by Herbert Olivecrona from Sweden, (3) the first reported embolization of an intracranial AVM in 1960 by Luessenhop and Spence, (4) the introduction of the operating microscope in neurosurgery in the mid-1960s, and (5) the first large microsurgical series of AVM resections reported in 1988 by M. Gazi Yasargil (414 patients). In the case of dural arteriovenous fistulas (DAVFs), microsurgical obliteration is often reserved for cases in which endovascular therapy either cannot be pursued or fails. When performed, however, microsurgical obliteration of DAVFs is associated with excellent outcomes as well. This article reviews the current state of microsurgical treatment of AVMs and DAVFs.
Arteriovenous malformations
Case Selection
In general terms, the angioarchitecture of the AVM as well as the patient’s age and medical/neurologic condition are the major determinants of whether a patient is a candidate for surgery. Age is the major determinant of the patient’s risk of hemorrhage and also of how well he or she can tolerate surgery. To calculate the actuarial lifetime risk of hemorrhage for a specific patient, one of two methods can be used. The simple method is to subtract the patient’s age from 105. This method assumes a 3% annual risk of hemorrhage. The more accurate, but also more complex method, is to use the formula for the multiplicative law of probability (1 − [1 − annual risk of hemorrhage] expected years remaining of life ), which assumes a constant annual risk of hemorrhage and independent event behavior each year. Older patients and those with debilitating medical conditions are obviously poor surgical candidates.
Determining whether a particular AVM is suitable for surgery, on the other hand, is a more complex issue, given the numerous anatomic variations associated with these lesions. This analysis, however, was simplified by the introduction in 1986 of the Spetzler-Martin scale and by its modification in 2003 by Lawton. The Spetzler-Martin scale reduces the analysis of an AVM to its size (S), pattern of venous drainage (V), and eloquence of adjacent brain (E), and has proved to be predictive of surgical outcomes. In the large Finnish series of 623 AVM cases accrued over 55 years (1951–2005), 13% were Grade I, 29% were Grade II, 32% were Grade III, 19% were Grade IV, and 4% were Grade V. Grade I and II AVMs, which comprise about 42% of all AVMs, are generally considered favorable for surgery. In their original series of 100 cases, Spetzler and Martin reported no morbidity in 23 Grade I AVMs and a 5% morbidity in 21 Grade II AVMs. By contrast, they reported 27% and 31% morbidity in Grade IV and Grade V AVMs, respectively. Therefore, Grade I and II AVMs are favorable for surgery, but Grade IV and V AVMs are not. Han and colleagues as well as Heros have gone on to recommend no treatment for most Grade IV and V AVMs.
Grade III AVMs are a varied group at the boundary between favorable and unfavorable surgical outcomes, and also happen to be the most common type of AVM. In the original Spetzler-Martin series, all Grade III AVMs were associated with 16% morbidity. Lawton studied in detail 76 Grade III AVMs (out of 174 total AVMs) and proposed a modification of the Spetzler-Martin system that has proved valuable in terms of surgical selection. Of the 76 Grade III AVMs, 46.1% were small (S1V1E1), 18.4% were medium/deep (S2V1E0), and 35.5% were medium/eloquent (S2V0E1). (Lawton did not encounter any S3V0E0 AVMs.) In this series of Grade III AVMs, the combined surgical morbidity/mortality was 2.9% for small AVMs, 7.1% for medium/deep AVMs, and 14.8% for medium/eloquent AVMs. Therefore, whereas a small Grade III AVM (<3 cm) with deep drainage and in an eloquent location is still a favorable surgical lesion, a medium Grade III AVM (3–6 cm) in an eloquent location is not. Deep venous drainage alone does not seem to be associated with poor surgical outcomes.
It follows that because Grade I and II AVMs make up about 42% of all AVMs, and Grade III AVMs make up about 32% of all AVMs, and because S1V1E1 and S1V1E0 lesions combined make up about 64% of all Grade III AVMs, the total proportion of AVMs that are favorable for surgery is about 62% (42% + [32% × 64%]).
Although these two scales provide helpful guidelines for surgical selection, many other factors will affect this decision. Lawton and colleagues have proposed a further refinement of their selection criteria by considering older age, hemorrhagic presentation, nidal diffuseness, and a deep perforating arterial supply as negative predictive features. Patients with Grade I and II AVMs but with serious medical conditions, such as severe coronary disease, are best treated radiosurgically. By contrast, a patient with a Grade IV AVM previously treated with serial embolizations and several rounds of radiosurgery, who presents with recurrent hemorrhages, then becomes a reasonable surgical candidate. Incidentally, the authors continue to favor the original formulation of the Spetzler-Martin scale over its recent 3-tier modification.
Preoperative Imaging
Conceptually, AVMs are best approached as both vascular lesions and mass lesions. As such, they should be imaged with computed tomography (CT) and magnetic resonance imaging (MRI) scans, as well as full catheter angiography, which should include external carotid artery circulation injections. The CT scan is best for identifying acute and resolving hemorrhages, calcifications, and the location of embolic material. The MRI, particularly the T2 sequence, provides an excellent correlation of the AVM components with the surrounding parenchyma. The angiogram obviously provides detailed imaging of the arterial feeders and the draining veins. One should recognize that the angiogram typically shows only the major arterial feeders and anticipate that more will be encountered during surgery as the dissection proceeds, especially in the deepest portions of the AVM. The venous phase of the angiogram is particularly important because once the surface of the brain is exposed at the time of surgery, one will use the surface veins to become oriented to the location of the nidus. The benefits of functional MRI in the planning of AVM resection remain to be established.
Preoperative Embolization
Preoperative embolization of an AVM should be considered strategically, keeping in mind that each embolization carries approximately 3% morbidity and 1% mortality. AVM embolization has dramatically changed the surgery of complex AVMs. Deep arterial feeders likely to be encountered late in the dissection are excellent candidates for embolization. Embolization can also be used to demarcate an arterial boundary of an AVM in eloquent cortex, or to reduce flow through a hyperdynamic AVM. From the perspective of surgical manipulation, both n-butyl-2-cyanoacrylate (NBCA; Cordis Microvascular Inc, New Brunswick, NJ) and ethylene vinyl alcohol copolymer (Onyx; ev3 Inc, Irvine, CA) are easy to displace and cut. In general, the authors prefer to dissociate the risks of embolization from those of surgery by allowing 7 to 10 days between the two interventions.
Associated Aneurysms
Approximately 7% to 8% of AVM patients have at least one intracranial aneurysm. Nidal aneurysms are an integral part of the AVM and do not require separate surgical consideration. Prenidal aneurysms, by contrast, are potentially treacherous and should be treated at the time of AVM resection, preferably before occlusion of the main arterial feeders ( Fig. 1 ) or before surgery if they are distant from the surgical field. The concern is that serial occlusion of the AVM arterial feeders can result in an increase in pressure and rupture of a prenidal aneurysm.

Intraoperative Adjuvants
Electrophysiologic monitoring, in the form of electroencephalography, somatosensory evoked potentials and, in the case of posterior fossa AVMs, brainstem auditory evoked responses, is essential during AVM surgery. Although motor evoked potentials (MEPs) have been advocated by some groups, the muscular contractions during MEP runs are inconvenient during microsurgical dissection. MRI-guided or CT-guided intraoperative navigation can be helpful in centering the craniotomy over small or medium convexity AVMs. For very small convexity AVMs, the authors use intraoperative angiography for localization. Intraoperative angiography is essential to confirm complete resection of the nidus and detect inadvertent compromise of a normal vessel. A recent review of 14 series (330 patients) of intraoperative angiography in AVM surgery showed that in 15% of cases management was changed by the angiogram results. The benefits of microscope-integrated indocyanine green angiography during intracranial AVM surgery remain to be established, although its usefulness in DAVFs is becoming apparent.
Staged AVM Surgery
Up until the early 1990s, the authors treated 3 large AVMs in two scheduled surgical sessions. During that time they were still operating on many Grade IV and a few Grade V AVMs while assessing the efficacy of combined treatment with serial embolizations followed by radiosurgery. At present the authors rarely use this option, but staged surgeries should be kept in mind in the case of a large and complex AVM that may require more than 12 hours of surgery or one that has arterial feeders that cannot be reached through a single exposure. In the Andrews and Wilson series of 319 AVMs, 9 (3%) were treated in two staged surgeries without apparent adverse effects from the staging itself. In the authors’ 3 cases the first surgery was limited to occlusion of the major feeders and taking even minor draining veins was avoided. At the conclusion of the first surgery, the dissection plane was marked with oxidized regenerated cellulose sheets (Surgicel; Ethicon SARL, Neuchatel, Switzerland). The patients were extubated and kept sedated in the intensive care unit. Their mean arterial pressure (MAP) was kept below 100. The two surgeries were separated by 1 to 3 days. The day before the second surgery, a catheter angiogram was obtained to update the anatomy of the AVM. No adverse sequelae from the staging itself were found.
Surgical Approach
From an angioarchitectonic perspective, AVMs are highly variable and each lesion requires an individualized surgical strategy that builds on the 4 basic steps described below. Surgically AVMs can be divided into cortical (surface) lesions, which are visible on the surface, or subcortical (deep) lesions. Cortical AVMs can be limited to the cortex, or can traverse the cortex and white matter, and breach the subependymal layer into the ventricle ( Fig. 2 ). Subcortical AVMs can be found within the deep white matter with or without extension into the ventricular surface, within the deep gray matter structures, or, in rare instances, exclusively within the ventricle (choroidal AVMs).

In almost all cases, the basic strategy for microsurgical AVM resection consists of: (1) creating a wide craniotomy centered over the nidus and comfortably encompassing the arterial feeders and all the draining veins, (2) gradually devascularizing the AVM by occluding the arterial feeders, (3) circumferentially separating the AVM from the adjacent parenchyma, and (4) finally dividing the draining veins ( Fig. 3 ). Early compromise of a major draining vein should be avoided at all costs. When properly executed, an AVM resection is one of the most elegant and satisfying procedures in neurosurgery. With the exception of life-threatening hematomas, AVM surgery should be scheduled electively.

Positioning
The authors use either the supine or the lateral decubitus (“park bench”) position for AVM surgery. The head should always be above the level of the heart. Whenever possible it is ideal to keep the head straight (in relation to the chest) and slightly extended to maximize venous return in the neck (the “sniffing position”). The authors avoid extreme head rotation in the supine position, which can compromise contralateral venous drainage, and prefer the lateral decubitus position in such cases. The authors rarely use the prone or Concorde position because it compromises venous return in both chest cavities, requires marked flexion of the neck, and often causes brain swelling and increased venous pressure intracranially. Chi and Lawton have discussed the advantages of the lateral versus prone positions in 46 patients (23 lateral and 23 prone) who underwent the posterior interhemispheric approach for vascular lesions. The authors have found that interhemispheric and posterior midline AVMs can be reached comfortably with the patient in the lateral decubitus position. Whether the patient is placed in the right lateral or left lateral position depends on how far off the midline the lesion lies (keeping in mind the advantages of the contralateral interhemispheric transcallosal or transcingulate approach ), and on maximizing gravity retraction on the dependent lobe or hemisphere. An additional advantage of the lateral position for interhemispheric lesions is that the surgeon can work with his hands side by side as opposed to one on top of the other, as required in interhemispheric cases positioned either supine or prone.
The authors use lumbar drains intraoperatively for AVM locations in which large cisterns will not be accessible in the early stages of the surgery, such as interhemispheric (parafalcine, callosal, cingulate) or occipital AVMs. In such cases, lumbar cerebrospinal fluid (CSF) drainage can be used for relaxation early on in the case until a large cistern or the ventricle is reached for removal of larger quantities of CSF. The authors no longer use the sitting position because of the risks of hypotension and venous air embolism.
Craniotomy and Dural Opening
The craniotomy should be wide enough to comfortably expose the normal brain surrounding the AVM. The entire superficial draining venous system should be exposed, including the superior sagittal sinus in the case of parafalcine, callosal, cingulate, or convexity AVMs, or the transverse sinus in the case of posterior temporal, inferior parietal, or occipital AVMs. If external carotid circulation feeders are present, they should be incorporated into the flap to occlude them early in the approach. When making and connecting the burr holes, one should be mindful of the course of the major draining veins to avoid inadvertent injury or compression of bulging veins. In general, more burr holes and short passes between burr holes are preferable ( Figs. 4–7 ).


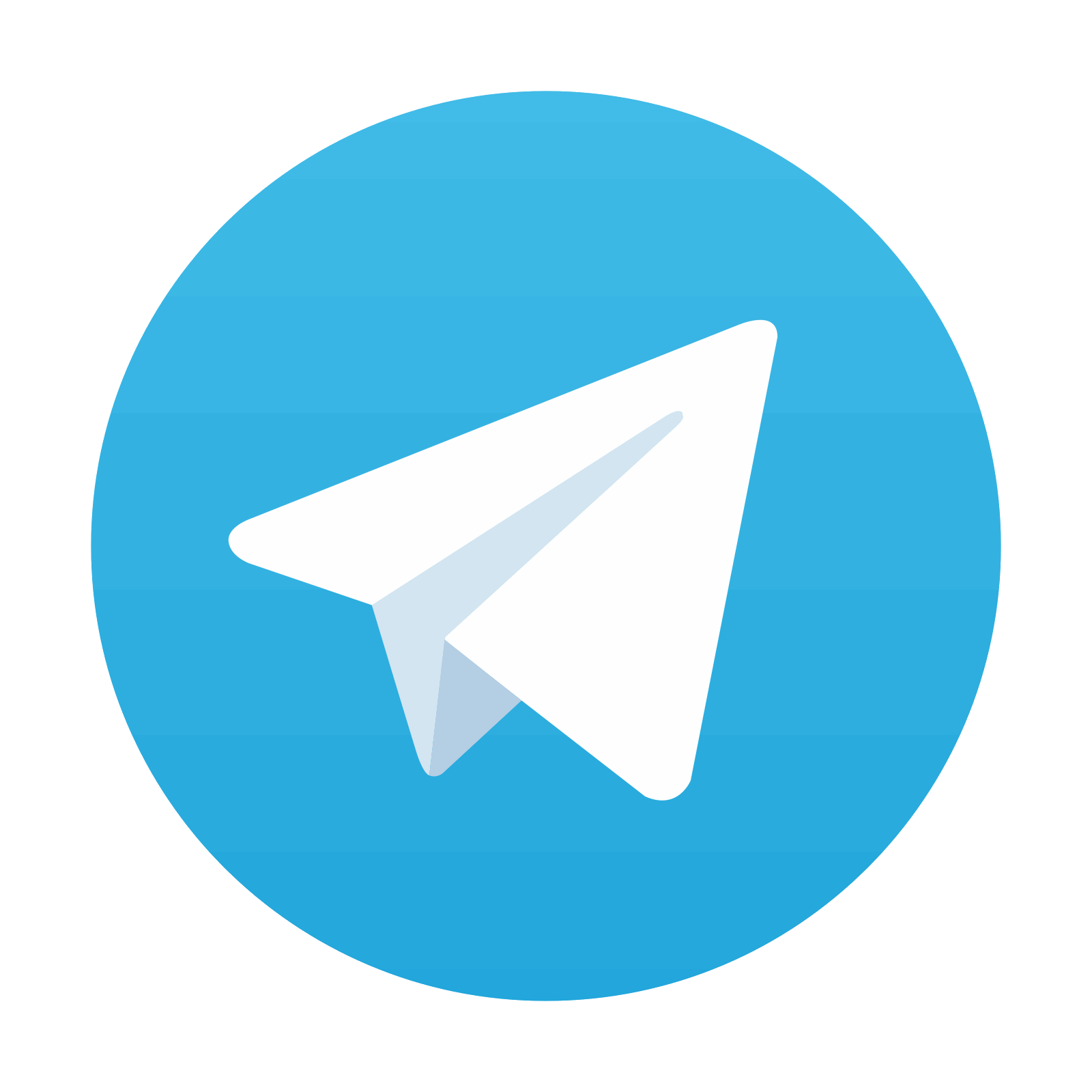
Stay updated, free articles. Join our Telegram channel

Full access? Get Clinical Tree
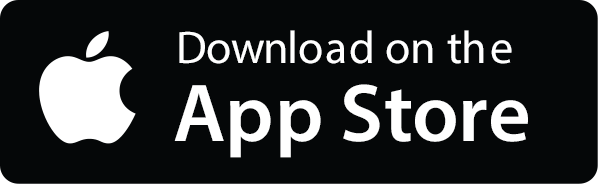
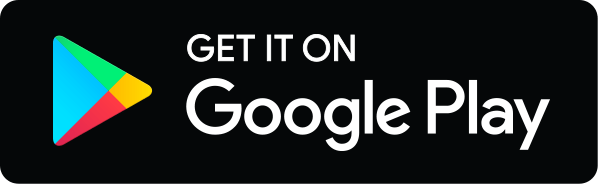
