Fig. 1
Analogy between the coordination of muscles by means of muscle synergies driven by the CNS and the coordination of an orchestra by a director
Making an analogy, let us consider muscles as musicians in an orchestra (Fig. 1). The overall symphony normally results from several melodies combined together, each of them played by a different group of musicians (e.g., violins, trumpets, and cellos). From the point of view of the director (corresponding to the brain), the apparently complex task of coordinating all music players is turned into the simpler task of modulating a limited set of basic melodies, each of them associated to a defined group of musicians. We call ‘synergies’ the groups of musicians playing each melody. In conclusion, the appropriate ‘activations’ of ‘synergies’ produce the coordinated activity of body muscles, which results in functional movements (the ‘symphony’).
If the CNS combines a small number of muscle synergies to generate motor commands, we expect to recognize regular patterns in the motor output. In particular, we expect to recognize a number of synergies (muscle groups) smaller than the number of muscles (back to the analogy: having a number of basic melodies smaller than the total number of instruments). Conversely, in the absence of any synergistic control, we expect to find as many synergies as the number of muscles, meaning that muscles are not grouped together, but rather they are activated independently. In this extreme case, the term ‘synergy’ will lose meaning, because each synergy would drive just one muscle and not a group of muscles. Mathematically, this concept can be modeled in different ways, whose description goes beyond the goal of this chapter. For those readers interested in these mathematical formalizations, please read [45, 65].
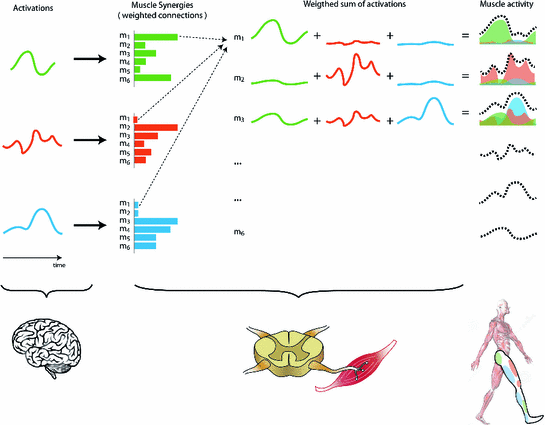
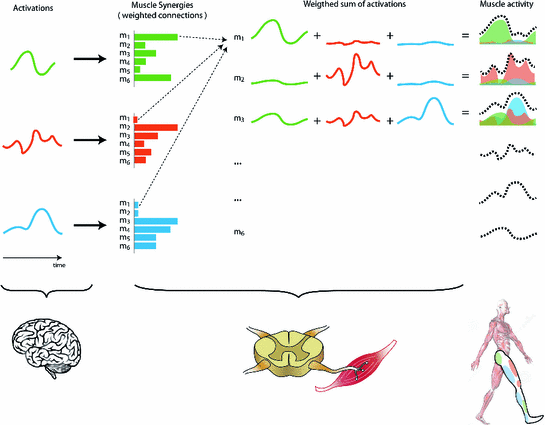
Fig. 2
Under the muscle synergies hypothesis, muscle activities result from a combination of a few activation signals mediated by muscle synergies. Functionally, muscle synergies assume a similar role of spinal interneurons, which translate supraspinal commands into
-motoneurons recruitment, by means of appropriate-weighted connections

Muscle synergies not only describe which muscles work in coordination, but also “how” they work together. As shown in Fig. 2, each synergy describes the extent to which each muscle contributes to the synergy it belongs to. Under the proposed analogy, the synergy would specify the intensity of each instrument within the same group of musicians (e.g., 3 out of 6 violins should play louder than the others). This concept is expressed mathematically as weights. Synergies represent the ensemble of weighted connections between activations and muscles, resembling the role of spinal interneurons that translate supraspinal commands into
-motoneurons recruitment.

A growing number of studies have provided evidence for the synergistic hypothesis, showing that the muscle patterns can be reconstructed by a number of synergies much smaller than the number of muscles, in a variety of species, behaviors, and tasks. In particular, studies have been conducted with frogs [12, 17, 21, 30, 58, 66], cats [61, 62] , monkeys [50, 51], and healthy human subjects while walking [15, 25, 38, 42], running [11], reaching [18, 20, 47], fingerspelling [43, 69], standing during postural perturbations [63, 64], cycling [4, 23, 35, 37, 68], catching [1], and generating isometric force [6, 9, 26, 54]. Moreover, s few studies have provided theoretical interpretations of the synergy hypothesis ffff. A number of reviews on muscle synergy decomposition have been published recently [2, 8, 19, 27, 44].
1.3 Muscle Synergies as a Novel Assessment Tool of Neurologic Pathologies
Disorders of the CNS such as stroke and spinal cord injuries (SCI) are characterized by several motor deficits resulting from inappropriate muscle activity and coordination. Among these deficits, patients with stroke show stereotyped muscle activation patterns due to the loss of independent control. The analysis of muscle synergies, combined with behavioral and biomechanical measures, is expected to highlight these modifications and provide a better understanding of the deficits that result from the impaired nervous system [57]. Since the design of present and future motor interventions strongly relies on the scientific understanding of the neural function and plasticity, muscle synergy analysis could be exploited to improve our understanding of the motor recovery process and to design new effective therapeutic approaches [59]. For these reasons, some groups have recently started looking at muscle synergies organization in upper and lower extremities of patients with SCI and stroke.
1.3.1 Muscle Synergies in SCI Subjects
So far, just few studies have been conducted to investigate the effect of SCI on muscle synergies [41]. Ivanenko and colleagues [40] studied lower limb muscle synergies extracted in 11 SCI subjects with a thoracic lesion around T8, characterized by different sensory and motor impairments, during treadmill walking at different speeds and different levels of body weight support. Results showed that with respect to the healthy people, temporal activations were preserved, while the structure of muscle synergies was altered (see Fig. 3): in particular, the synergies of the less impaired subjects were more similar to the healthy ones than those of the most affected subjects.
Another study extended these findings investigating a population of 8 SCI patients presenting incomplete lesions at different levels of the spinal cord (from C4 to T10), during overground walking [31]. The number, composition, and activation of muscle synergies were altered with respect to healthy controls. In particular, the patients showed fewer muscle synergies and altered activations. Remarkably, the alteration of muscle synergies reflected an altered muscle coordination, which could limit safe and effective walking.
A further study showed how the organization of muscle synergies was also altered in upper limb, during different grasping movements [70].
In summary, the few investigations of muscle synergies in SCI subjects suggest that the plasticity induced by the lesion and by the training after the lesion leads to a reorganization of the connections of the interneuronal networks in order to modify and create new muscle synergies while the temporal organization of the activation coefficients remains preserved [40, 41]. The reorganization of muscle synergies occurs both in upper and lower extremities [40, 70], while in some cases, it is also possible to observe alteration of their number and activations [31].
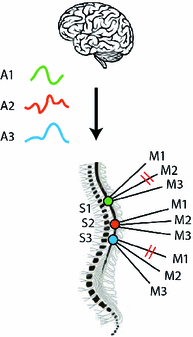
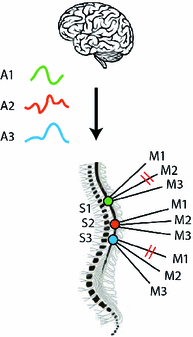
Fig. 3
SCI may disrupt the weighted connection constituted activations and the
-motoneurons connecting each muscle. Despite activations may be preserved and controlled by supraspinal circuits, the synergy vectors will be different (different weights because of the disruption of some interneurons) A: Activation; S: Sinergy; M: Muscle

1.3.2 Muscle Synergies in Post-stroke Subjects
Few more studies investigated muscle synergies in post-stroke subjects. Concerning locomotion, Clark and colleagues [16] analyzed 57 chronic post-stroke subjects, characterized by different levels of impairment, during treadmill walking at different speeds. They found that muscle synergies were generally preserved, but the ability to differentially activate the synergies was compromised in many of the paretic legs. They also observed that fewer synergies were needed to account for the whole muscle activity in the paretic leg relative to non-paretic and healthy control leg. This decrease in the number of synergies was also correlated with degradation of clinical and biomechanical walking performance variables, such as the preferred walking speed, speed modulation, step length asymmetry, and propulsive asymmetry [10, 16]. The fewer number of muscle synergies in the affected limb has been hypothesized to result from the merging of more synergies normally observed in healthy subjects (this ‘merging’ phenomenon will be explained afterward).
Gizzi et al. [28] extended these results by investigating muscle synergies from 32 muscles on 10 patients recently affected by stroke (maximum 20 weeks), while they were walking overground at comfortable speed. Focusing only on lower leg muscles, they observed a preservation of the temporal organization of the synergy activations. By considering lower limb, trunk and upper limb muscles, the synergies, but not the activations, of the affected side were different from those in the unaffected side and of the healthy controls. The results induced the authors to speculate that these changes in muscle synergies could be due to compensatory strategies mainly carried out by the upper and trunk musculature.
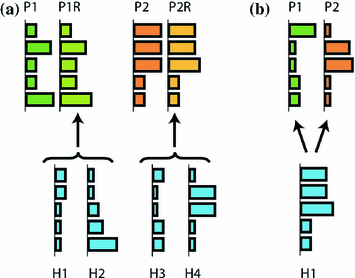
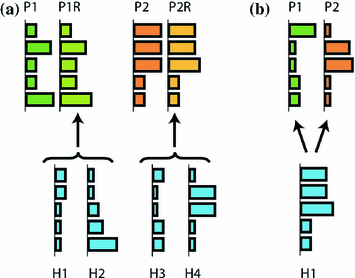
Fig. 4
Merging and fractionation of muscle synergies. a Merging of muscle synergies. Paretic side synergies may be explained as the merging of healthy synergies. For instance, healthy synergies H1 and H2 can merge and form P1R (paretic synergy reconstructed), which is very similar to the original paretic synergy 1 (P1). b Paretic synergies P1 and P2 may be explained as fractions of healthy synergy H1
Cheung et al. [13, 14] evaluated upper limb muscle synergies in a population upto 31 patients affected by (moderate to severe) stroke, while they were executing 3D reaching movements. In case of less impaired subjects, the muscle synergies of the unaffected and affected arm were similar even though the muscle activation patterns were different [13]. However, severe impaired subjects (Fugl-Meyer score
30) showed three distinct behaviors: the preservation, merging, or fractionation of the affected muscle synergies of the affected arm with respect to those of the unaffected (or healthy) arm [14] (see Fig. 4). The merging of muscle synergies (Fig. 4) is the process by which two groups of muscles that are normally independent to each other, become grouped. Under the synergy analogy, we may see this phenomenon as two independent groups of musicians that, at a certain moment (following a certain re-organization process), start to follow the same melody. The fractionation of muscle synergies appears when a group of muscles belonging to one synergy is divided in two groups, each following a different modulation signal.

Roh et al. [55] investigated upper limb muscle synergies in 10 chronic and severe (Fugl-Meyer score
25) stroke survivors, using a submaximal isometric force matching protocol. The activations were modulated in a task-dependent manner in healthy and stroke subjects, while an alteration in synergy composition was observed only in the stroke-affected arm. In particular, the altered recruitment was correlated with the abnormal task performances. In this case, no merging or fractionation was observed.

Finally, Tropea et al. [67] investigated the effects of the conjunction between natural recovery and intense robot-mediated treatment on muscle synergies in subacute patients (one week to four months after the lesion). Patients underwent 6-week rehabilitative training, performing planar robot-assisted reaching movements. The results suggested that (i) muscle synergies underlying shoulder control can reflect the functional deficit induced by the pathology, (ii) muscle synergies can be modified by the rehabilitative treatment, and (iii) the improvement of motor performance was achieved in conjunction with a slight, even though not statistically significant, restoring of the coordination of the activity of shoulder muscle groups.
Overall, all observational studies of muscle synergies in stroke subjects showed that the organization of muscle synergies depends on the level of impairment and maybe on the onset of the cerebrovascular accident. In particular, in severe impaired chronic stroke subjects, in the lower and upper limb, it is possible to observe the preservation, merging or fractionation of muscle synergies. In subacute or mildly impaired stroke subjects, it is possible to observe the preservation of muscle synergies. In both lower and upper limb, the activation coefficients of muscle synergies are generally preserved, and, in the upper limb, the synergies dominated by the activation of shoulder muscles are generally altered.
The merging and fractionation may be interpreted as a possible compensatory or adaptive reorganization of brainstem and spinal control [14], and the degree of merging seems to be related to the degree of motor and functional deficit. The merging of upper limb muscle synergies can be ascribable to the higher muscle co-contraction in the affected side, analogously to what was found in the lower limb [16]. While the merging was related to the severity of the impairment, the fractionation emerged only after years from the initial stroke lesion, but whether it is an adaptive process triggered in response to the motor impairment following stroke remains an open issue [14].
Muscle synergies can be modified by the motor reorganization following a rehabilitative process [67]. In particular, modifications involving a reorganization of muscles within the same muscle synergy seem to occur in a short-time period, while the fractionation process of merged synergies seems to require more time [14].
1.4 Muscle Synergy Analysis in the Clinical Setting
In this section, we will provide a step-by-step guide to those practitioners willing to understand how to perform synergy analysis in a typical clinical setting. We will give examples based on previous experiments during cycling movements. Most of the information included in this section can be found in [4], from which data are extracted and adapted.
The basic experimental data that a researchers or clinician needs in order to perform synergy analysis is muscle activity. This is usually done by non-invasive electromyography (EMG) analysis which is, the technique of recording the superficial electrical muscle activity by means of electrodes placed on the skin surface. The recorded EMG signals should be consequently processed and then used as input to special algorithms responsible for extracting the synergies and their corresponding activations. The analysis of synergies ends with the interpretation of results. Each of these four steps (shown in Fig. 5) entails several aspects that should be taken into account to extract meaningful information. In the following subsection, we will bring the reader throughout this process.


Fig. 5
General experimental procedure to analyze muscle synergies
STEP 1. Recording EMG
The first step in the experimental design is defining which muscles should be recorded. As the analysis of muscle synergies intends to unveil the coordination strategies over a large set of muscles, it is important to record the muscular activity of as many muscles as possible. It is recommended to at least record those that play an important role in the motor task or behavior. Participants should be instructed to refrain from intense physical activities for at least 2 days before testing [4], to avoid biasing the results due to fatigue.
The positioning of the electrodes on the skin strongly influences the quality of the EMG signal: badly positioned or oriented electrodes can produce significant variations in the signal quality and/or reliability. The SENIAM project [32] developed a set of recommendations about the placement of sEMG electrodes on the different muscle groups. After selecting the muscles, the researchers should therefore follow these recommendations for sEMG recording procedures: shave the places where the electrodes are placed; clean the skin with alcohol to minimize impedance; allow the alcohol to vaporize in order to dry the skin before placing the electrodes. After that, the bipolar electrodes (with a 2-cm interelectrode distance) should be fastened to the specific locations, according to the respective sensor placement procedure in SENIAM. In the case of electrodes connected by cable to the EMG acquisition system, it is recommended to wrap the electrodes with bandages to ensure that the wires do not impede the subject’s movement and also to avoid movement-induced artifacts. Finally, care should be taken to ensure that the reference electrode is placed as far away as possible from the recorded muscles and on electrically neutral tissue (say over a bony prominence) and that it makes very good electrical contact with the skin.
To ensure the correct placement of the electrodes, some preliminary tests to check for cross talk and cable-induced noise should be performed. If needed, electrodes should be repositioned [4].
Depending on the motor behavior or task recorded, some additional events could be detected to segment the data into individual cycles. For instance, in the case of cycling, the crank angle should be also measured, and synchronized with the EMG recordings.
Finally, at the end of each trial, data have to be stored to allow for further offline processing.
STEP 2. Processing the EMG
A correct placement of the electrodes does not imply that the recorded EMG signals are appropriate for synergy extraction. Many disturbances may affect such signals. Thus, the investigator should inspect and preprocess the dataset in order to minimize their influence on the analysis. The idea is to remove all the features of the raw data that do not relate to muscle activation. Although it is impossible to guarantee a perfect removal of all these undesired features without affecting the signal, a series of methodologies can be adopted to minimize them.
The typical disturbances that affect EMG signals can be classified as follows [53]:
Filtering is the process of removing the disturbances described above by applying appropriate mathematical techniques, generically called filters. While a detailed description of these techniques is out of the scope of this chapter, it is worth spending some words on the frequency ranges that should be particularly taken care of.
1.
Inherent noise in the electronics equipment. This source of noise is originated from the electronics equipment used for detecting, amplifying, and recording the signals. The usage of high-quality electronics can reduce this disturbance.
2.
Ambient noise. This noise component arises from the electromagnetic radiations in the ambient where the recordings are conducted. In particular, its main cause is the radiation from the electrical power line, which can be 50 or 60 Hz, depending on the country.
3.
Motion artifacts. These disturbances derive either from movements of the cables connecting the electrodes and the amplifier, or from the interface between the electrode and the skin. Thus, they result in low-frequency fluctuations in the EMG signals.
4.
Inherent randomness of the signal. Motor units are characterized by a quasi-random firing rate in the range between 0–20 Hz. This behavior affects the amplitude of the EMG signal, which in this frequency region can be considered unrelated to the task at hand, and therefore unwanted.
In order to facilitate the explanation of the filtering process, we will refer to Fig. 6, which depicts the step-by-step processing on a real EMG signal acquired during a cycling task from the vastus lateralis muscle of a healthy subject.
The bandwidth of EMG signals is 0-400 Hz, indicating that the main information of muscle activity is included in this range of frequencies. Thus all the frequencies above 400 Hz should be eliminated by the so-called low-pass filter. Motion artifacts, characterized by slow fluctuations in the raw original signal (see Fig. 6a), can be eliminated by filtering the low frequencies of the signal, typically setting a high-pass filter with cut-off frequency around 10 Hz [22].
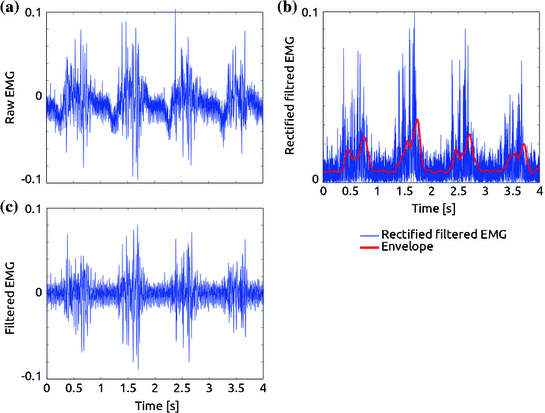
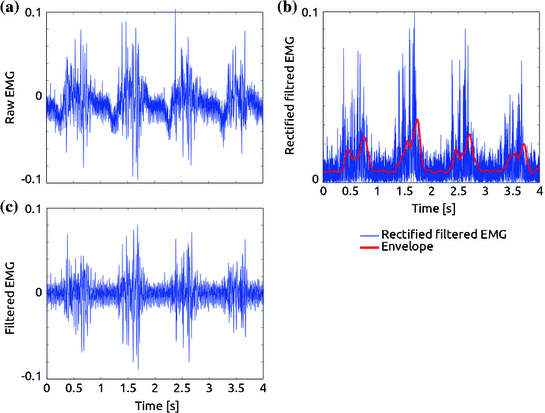
Fig. 6
Processing of a surface EMG signal acquired during a cycling task from the vastus lateralis muscle of a healthy subject. The raw signal (a) is corrupted by motion artifacts (low-frequency fluctuations) and high-frequency noise (not clearly visible in figure). By applying a low-pass and high-pass filters at, respectively, 400 and 20 Hz, these disturbances are removed (b). The signal is then rectified (c, blue line), and the envelope is extracted by applying a low-pass filter with cut-off frequency of 5 Hz (c, red line)
Ambient noise can be almost totally eliminated with modern electronics technology. If required, although not suggested [53], a “notch” filter could be applied to eliminate selectively the frequency of the power line (50 or 60 Hz). Figure 6b shows an example of filtered EMG signal.
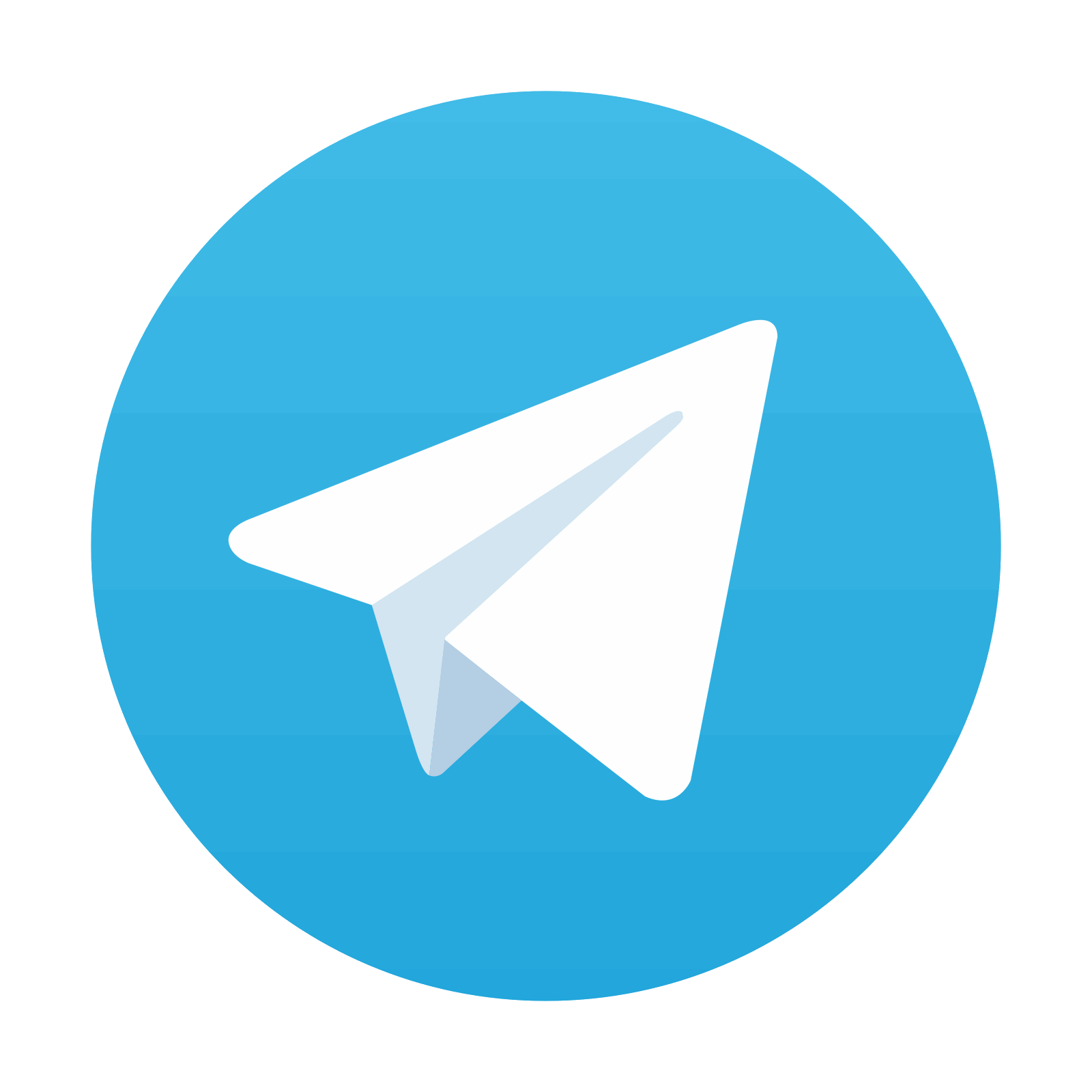
Stay updated, free articles. Join our Telegram channel

Full access? Get Clinical Tree
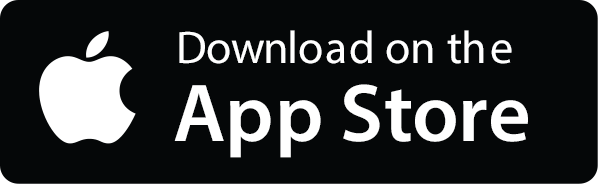
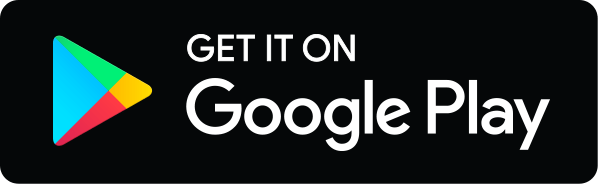