Introduction
Transcranial direct current stimulation (tDCS) is a noninvasive brain stimulation (NIBS) technique, whereby a subthreshold, continuous electrical current (typically 1–2 mA) is applied to the brain via two or more electrodes, placed over the scalp ( ). The conventional tDCS device consists of a portable, battery-operated machine, connected through conductive cables to two electrodes (an anode and a cathode), that are cushioned in saline-humidified sponge pads ( Fig. 18.1 ).

The device generates a current that passes through the skin, subcutaneous tissue, skull, meninges, cerebrospinal fluid, and finally, brain gray and white matter, with the direction of the electrical current flowing from the anode toward the cathode ( ). In general terms, the neurons under the anode tend to be partially depolarized, increasing their excitability and facilitating synaptic transmission ( ). On the other hand, in the area under the cathode, the neurons tend to hyperpolarize, decreasing their excitability and inhibiting synaptic transmission ( ).
These processes take place, however, with tDCS not directly eliciting action potentials, but rather facilitating or inhibiting (modulating) the already ongoing neuronal activity taking place in the stimulated regions ( ). This biophysical change in the target region also tends to influence other brain areas that are anatomically or functionally connected to it ( ). It is with this underlying rationale that different electrode montages are considered when treating a disorder in which a specific brain region or network is involved (for a detailed description of the biophysical and network mechanisms of tDCS, please see “ Mechanism(s) of action ” section).
tDCS has been intensively researched in diverse neuropsychiatric disorders, with variable degrees of efficacy, but with consistent safety and tolerability profiles ( ; ). In the treatment of major depressive disorder (MDD) in particular, tDCS has been found to be superior to sham, with moderate effect sizes ( ; ; ). Furthermore, pivotal trials have suggested tDCS’ synergistic effects with sertraline ( ), albeit being inferior, as monotherapy, to escitalopram ( ).
Currently, tDCS is not yet placed on the same level of clinical efficacy as other forms of NIBS for treatment-resistant depression (TRD), such as transcranial magnetic stimulation (TMS), which is an approved alternative after treatment failure with at least one antidepressant , or electroconvulsive therapy (ECT), for severe cases of depression, especially with psychotic symptoms ( ).
However, some advantages associated with tDCS, in comparison with other NIBS techniques (i.e., TMS and ECT), are its relatively low cost, portability, easiness of use, and the possibility of home-based treatment ( ). Moreover, tDCS is not associated with many of the most common adverse events observed with antidepressant medications (i.e., appetite, libido, and sleep alterations), nor with the adverse cognitive effects of ECT ( ).
In the treatment of MDD in general, and more specifically in the case of TRD, tDCS displays an augmentative and synergistic potential, both to an existing regimen of antidepressants, and/or to cognitive behavioral therapy (CBT) ( ).
In this chapter, we provide a detailed overview of tDCS as a potentializing intervention in TRD, encompassing recent advancements and interesting lines for future research.
History and development
Animal studies involving basic aspects of direct electrical current stimulation in the brain began in the 1950s, with observations that brief periods of intracerebral or epidural stimulation could produce long-lasting effects on neuronal membrane resting potential, depending on the polarity of the electrodes: the anode was found to increase the rate of neuronal firing, while the cathode led to hyperpolarization of neurons and to the decrease of the neuronal firing rate ( ; ). However, since the 1960s, it has been known that opposite polarization effects might also be observed in individual cortical layers, suggesting that effects of direct current stimulation might depend on other factors besides polarity, such as current direction and neuronal geometry ( ).
Perhaps the first placebo-controlled clinical trial to use noninvasive electrical brain polarization in the treatment of depressed patients was published in 1964, with the observation of clinical improvement in depressive patients receiving active treatment, especially regarding anxiety, agitation, and somatic symptom domains ( ). In this cross-over trial involving 24 inpatients, the electrical current was passed through 2 electrodes (anodes) placed over each eyebrow, while the cathode was placed on one leg, using a protocol of 0.25 mA during 8 h per day, for 12 consecutive days ( ). The protocol used in this trial was based on previous observations of optimal antidepressant response with current amplitudes at a maximum of 0.3 mA; however, antidepressant effects were observed to change in other amplitudes, depending on current density parameters ( ). At the time of the first brain polarization trials for depression, limitations in methodology and incipient neurobiological knowledge related to the disorder became obstacles in the development of the technique, which went gradually into oblivion over the next 40 years ( ).
Beginning around the year 2000, renewed observations involving the application of tDCS in humans began to shed deeper light on the technique’s longer-lasting behavioral and therapeutic effects. In a seminal study by , noninvasive electrical stimulation of the human motor cortex was shown to alter motor-evoked potentials as elicited by single-TMS pulses ( ). In this study, anodal stimulation led to an increases in cortical excitability, while cathodal stimulation led to subsequent decreases in this parameter; the authors also noted that strength and duration of after-effects could be controlled by modifications of current intensity and duration of stimulation ( ).
Later, it was observed that patients with depression commonly display an asymmetrical pattern of functional interhemispheric activity, with a reduction in prefrontal activity of the left hemisphere, and an increase in the right in comparison to heathy individuals ( ; ). This neurobiological model offered a rationale for the most commonly used tDCS montages in the treatment of MDD, whereby the “excitatory” anode is usually placed over the left hemisphere, while the cathode is placed over the right hemisphere ( ).
The first modern RCT involving the use of tDCS in MDD was published in 2006 ( ). In this trial, 10 patients with MDD received 5 days of anodal stimulation (1 mA for 20 min/day) over the left dorsolateral prefrontal cortex (DLPFC) or sham. Results showed four treatment responders in the active group,no responders in the sham group, and no reports of major adverse effects ( ).
These positive initial results gradually led to dozens of trials involving the use of tDCS for MDD, with varying individual degrees of efficacy, but with overall moderate effect sizes versus placebo. Additionally, consistent patient tolerability and general safety allowed for the continued research of this NIBS modality, as shall be described below.
Mechanisms of action
The exact mechanism of action of tDCS is still to be fully elucidated. At the cellular level, tDCS is believed to induce a change in neuronal membrane potentials, affecting the probability and timing of spikes (neural firing rates) ( ; ). In vitro experiments using rat motor cortex brain slices indicated that anodal stimulation produces partial depolarization of the neuronal soma, with the cathode producing somatic hyperpolarization, respectively increasing or decreasing the probability of triggering action potentials ( ).
Initial neurophysiological studies with tDCS applied to the motor area also pointed in the same direction. When accessing motor-evoked potentials (MEPs) elicited by simple TMS pulses, researchers showed an increase in amplitude after anodal and a decrease after cathodal stimulation ( ). The latest research, however, suggests that this relationship is more complex and that parameters such as current intensity and duration of stimulation may have a nonlinear relationship with the effects of tDCS on cortical excitability. For instance, a study has shown that the application of both anodal and cathodal tDCS to the motor cortex, with an intensity of 2 mA for 20 min, led to an increase in the amplitude of MEPs, changing the direction of cathodal effects to excitability enhancement ( ).
More recent evidence also expands on classic findings and suggests that tDCS impacts neural functioning also at a network level, with its local effects spreading to other regions anatomically and functionally connected to the target area ( ). The current intensity commonly used in clinical studies in humans (typically 2 mA) is not sufficient to elicit action potentials. Therefore, tDCS would act influencing the ongoing oscillatory dynamics of nodes of the network and in that sense, selectively affecting the “active” neurons, which are receiving synaptic inputs (in contrast to the neurons “at rest” of the inactive networks at the time of stimulation) ( ).
Therefore, for depression, most of the studies that place the anode over the left DLPFC use this region as a “window” to enter large-scale brain networks like the default mode network (DMN), for which the DLPFC is a key hub. The DMN is believed to be involved in self-referential processing, affective cognition, and emotion regulation ( ). Facilitating its endogenous activity and normalizing functional connectivity with key regions within the network (like the subgenual anterior cingulate cortex, sACC) could potentially lead to an improvement in depressive symptoms, as shown after a course of TMS treatment ( ; ).
Moreover, due to the diffuse nature of tDCS, it can stimulate other regions and potentially, deeper structures that are in the path of the current between the electrodes ( ). Although evidence suggests that tDCS may functionally target neuronal dynamics ( ), the arrangement of the electrodes (montage) also plays an important role in the final effect of the stimulation ( ).
The long-term effects of tDCS are believed to be due to synaptic plasticity modulated by LTP- (long-term potentiation) and LTD- (long-term depression)-like mechanisms ( ). Pharmacological studies in humans ( ) and animal electrophysiology experiments ( ; ) corroborate this hypothesis, although the exact biophysical mechanism involved is still unknown ( ).
Clinical efficacy of tDCS for TRD patients
The clinical efficacy of tDCS for the treatment of depression has been extensively investigated in the past two decades. The first RCT of tDCS for depression was conducted by Fregni and colleagues in 2006 ( ). In this pilot trial, the authors evaluated the effectiveness of tDCS (1 mA, 20 min, 5 consecutive sessions) compared to a sham intervention in 10 adult patients with a diagnosis of depression, and found a significant improvement in depressive symptoms in the active group ( ). Afterwards, a larger RCT ( n = 40) with antidepressant-free patients was conducted also showing clinical improvement in the active tDCS arm (2 mA, 20 min, 10 sessions) ( ).
However, a few subsequent RCTs were conducted with medicated and treatment-resistant patient samples, failing to show any significant difference between active and sham tDCS for depression improvement. For instance, Blumberger et al. recruited 24 adult patients with treatment-resistant depression (defined as failure to achieve remission or inability to tolerate two trials of an antidepressant medication from separate classes) and applied 15 tDCS sessions (one treatment per weekday), delivered at 2 mA for 20 min ( ). Both the continuous outcome (depression scale improvement) and remission rates did not differ between active and sham tDCS after the 3 weeks of treatment ( ). Palm et al. also conducted a trial with 22 treatment-resistant subjects (TRD defined with the same criteria used by ) that were taking antidepressants in a stable regimen ( ). The authors applied a cross-over design (10 active and 10 sham sessions) with tDCS intensity of 1 mA or 2 mA for 20 min ( ). The first 10 patients were treated with 1 mA and the other 12 with 2 mA. Again, the authors failed to show any meaningful therapeutic effects of tDCS compared to sham for TRD patients, even applying both stimulation intensities ( ).
Later, Brunoni and colleagues performed the SELECT-TDCS study, a factorial clinical trial evaluating the combination of tDCS and sertraline (50 mg/day) compared to tDCS, sertraline, or sham alone ( ). Overall, the authors found that the efficacy of tDCS as monotherapy was similar to the antidepressant drug, while the combined group (tDCS + sertraline ) presented superior depression improvement compared to the other arms ( ). Interestingly, secondary analyses suggested that treatment resistance (more than one failed antidepressant trial) was a predictor variable for a lower treatment response ( ). TRD was also associated with lower tDCS efficacy in all predictor models conducted by a subsequent individual patient data meta-analysis of six randomized controlled trials ( n = 289 patients), in which tDCS effectiveness for depression was assessed ( ). Moreover, based on the previous findings of Blumberger et al. and Palm et al., a guideline on the therapeutic use of tDCS considered the technique “probably ineffective” for TRD patients ( ).
More recently, Brunoni and colleagues conducted the ELECT-tDCS , a noninferiority trial comparing the effects of tDCS and escitalopram for depression and showed that tDCS treatment was inferior to the antidepressant drug (20 mg/day), although superior to sham ( ). This was the largest tDCS trial for depression to date, with 245 participants, of which 30% of the included patients in the tDCS group were resistant to at least 1 antidepressant treatment in the current episode, or with > 4 treatment failures over the patient’s lifetime ( ). Secondary analyses of this study also showed that TRD patients presented a negative association with depression improvement, when comparing active tDCS to sham intervention ( ).
Results from an individual patient data (IPD) meta-analysis also confirmed the tDCS effects presenting a negative association with TRD patients ( ). Nonetheless, two recent meta-analyses that showed tDCS having a moderate effect for depression (n = 25, Hedges’ g = 0.46, 95% confidence interval (CI): 0.22–0.70 and n = 9, β = 0.31, 95% CI: 0.15– 0.47, respectively) ( ; ) were not able to identify this negative association. Some hypotheses for these new findings suggest that the results of the first IPD meta-analysis were probably driven by the large study of Brunoni et al. conducted in 2013 ( SELECT-TDCS ), with a low-treatment–resistant sample showing clinical efficacy of tDCS ( ), whereas other studies with smaller sample sizes and higher treatment resistance showed no effects of tDCS ( ; ; ).
Finally, a very recent guideline focusing on the evaluations of the effects of tDCS for neurological and psychiatric disorders considered anodal tDCS on the left DLPFC definitely effective (Level A) in improving depression in major depressive disorder, even for treatment-resistant patients ( ) . Thus, the findings of the recent studies in the tDCS literature have been supporting the evidence that tDCS effects are not significantly discrepant between resistant and not treatment-resistant depression subjects. Therefore, even though the intervention is not yet approved by the U.S. FDA for depression, and its acute antidepressant effects are currently considered inferior when compared to TMS and ECT ( ), tDCS has been presenting considerable advantages to other NIBS techniques such as portability, easier application and lower cost.
Adverse effects
Several studies have already systematically assessed the safety, tolerability and acceptability of tDCS application for both healthy subjects and patients with neuropsychiatric conditions, enabling more reliable findings regarding these outcomes for tDCS treatment. In general, safety, acceptability, and tolerability are known, respectively, as the potential damage caused by a protocol, the rate of drop-outs for any reasons, and the impact of an adverse effect (AE) that does not cause functional and structural damage ( ). AEs are defined as any undesirable effect associated with the use of a medical intervention and can be classified as mild, moderate, serious or severe. The latter correspond to outcomes such as death, hospitalization, life-threatening events, disability or permanent damage, congenital anomaly/birth defect, or other serious important medical events (i.e., seizures/convulsions that do not result in hospitalization) ( ).
The first study to evaluate AEs related to tDCS application in humans was conducted by Nitsche and colleagues ( ). They reported the findings of approximately 500 participants receiving a tDCS current of 1 mA (35 cm 2 electrodes) for 20 min over the motor cortex, and only mild transient tingling sensations under the electrodes and/or light flashes when the stimulation was switched on or off abruptly were reported ( ). Posteriorly, a similar study by was conducted with data from previous studies of their group to evaluate safety aspects of tDCS over six different cortical areas ( ). Observations from a total of 567 tDCS sessions on 102 participants were summarized. None reported serious AEs, however, 70.6% of the participants reported a mild tingling sensation, 35.3% presented moderate fatigue, 30.4% felt a light itching sensation under the electrodes during the tDCS session, 21.6% reported a slight burning sensation, 15.7% reported pain sensation, and 10.8% presented difficulties in concentrating. Headaches and nausea were less reported (4.9% and 2.9%, respectively) ( ).
Two systematic reviews (SR) were conducted aiming to evaluate the safety, tolerability and acceptability of tDCS in several conditions. The first SR, conducted by , summarized data of 209 studies, of which 56% (117 studies) reported any AE. The most common AEs reported were itching (active vs. sham group: 39.3% vs. 32.9%), tingling (22.2% vs. 18.3%), headache (14.8% vs. 16.2%), burning sensation (8.7% vs. 8.7%), and discomfort (10.4% vs. 10%) ( ). According to the findings, all described AEs were considered mild, and no significant differences were found between active and sham groups. More recently, a study conducted a SR and meta-analysis to investigate acceptability and tolerability of tDCS treatment for several conditions ( ). Regarding acceptability, the authors showed that 6% and 7.2% of participants dropped-out from active and sham groups, respectively. However, almost half of the studies did not report dropouts and only 23.4% of the included experiments presented the reasons for discontinuations. Among the reasons, the most frequent were protocol violation and the presence of AEs. Regarding tolerability, the authors found tingling being the most common AE, followed by skin redness, itching, headache, burning sensation and sleepiness . Nevertheless, several studies did not report AEs and, when described, no systematic method was applied among the studies ( ).
Adverse effects in depression trials
Although the above findings show only mild adverse effects after tDCS application, some points should be underscored for depression trials. Loo et al . reported a case of suicide of a patient with depression the day after receiving an active tDCS session. The authors did not find any clinical changes, associated with the stimulation session, thus the event was not attributed to the tDCS application ( ).
Episodes of mania and hypomania have also been reported in studies investigating the effects of tDCS for unipolar and bipolar depression ( ). For instance, the largest study of tDCS for unipolar depression ( n = 245) reported two patients presenting episodes of new-onset mania after the beginning of the tDCS protocol ( ). Moreover, a recent meta-analysis showed that patients with unipolar depression present an increased risk of treatment-emergent mania when compared to the sham group. However, they could not predict whether these symptoms were caused by the tDCS application or due to a natural history of the condition ( ).
In turn, most antidepressant treatments carry a risk of inducing treatment-emergent mania, and it is still unclear whether tDCS treatment presents a higher risk when compared to other treatments. Other AEs, such as behavioral or cognitive effects were never reported in the literature of tDCS for depression ( ; ). Although some clinical trials of tDCS for unipolar depression patients have already reported (hypo)mania switch episodes, they were not yet directly associated with the tDCS application ( ). Thus, the low rate of reported adverse effects during depression treatment and other conditions makes tDCS a safe and well-tolerable intervention. However, before tDCS application, a few relative contraindications must be evaluated, such as skin problems (dermatitis, psoriasis or eczema), metallic implants in the head (under or in between the electrodes), subjects with neurological history (neurosurgery, epilepsy, malformation), and special populations (children and elderly) must be carefully considered ( ).
Summary and future directions
Contrary to other NIBS modalities (e.g., rTMS, theta-burst stimulation-TBS, deep TMS), tDCS has not yet received FDA clearance for the treatment of MDD. Nevertheless, tDCS has been extensively studied in all three important regulatory aspects (efficacy, safety and tolerability), and besides being deemed very safe and tolerable, its effectiveness as a treatment for MDD has been recently considered “definitely effective” in improving depression in MDD (anodal tDCS to the left DLPFC, level A) ( ) . Furthermore, it should be noted that in a previous meta-analysis, even though absolute effect sizes for TMS modalities were superior to those of tDCS, their confidence intervals overlapped ( ), raising questions as to which of these two NIBS interventions is superior to the other, and also, to what extent ( ). This is also complemented by the results of a recent umbrella review showing tDCS with “high” level of evidence for depression, together with high frequency rTMS ( ).
On the other hand, regardless of the question of superiority among different NIBS modalities, tDCS, unlike TMS and ECT, is portable and may be safely and effectively performed at home ( ). Home-based tDCS, with remote monitoring by mental health professionals, may also allow for longer continuation and maintenance phases in the treatment of MDD, as transportation and logistical burdens would be diminished ( ). This approach has already been performed for the treatment of MDD in a pilot trial (n = 34), presenting significant improvement in mood scores from baseline to the end of the acute phase and a low rate of discontinuation ( ) and in a case series (n = 5), with promising results ( ). However, despite these encouraging findings, it should be noted that these trial and case series lacked a control group, and only patients with adequate computer literacy were selected, which limits its generalizability. Therefore, although home-based tDCS seems a promising approach, trials with larger and broader MDD samples are needed in order to reach more definite conclusions.
Besides the investigation of home-based alternatives, the ongoing development of other strategies to improve tDCS delivery and antidepressant efficacy is deemed extremely necessary . In this regard, three approaches are worth mentioning: (1) the individualization of current dosing parameters, (2) the combination of tDCS with cognitive-behavioral interventions, and (3) the study of biomarkers and clinical predictors associated with tDCS response.
Currently, tDCS is generally delivered within fixed dosing parameters, which might help explain both within- and between-study variabilities regarding stimulation effects ( ; ). The electric field that effectively reaches the brain can be substantially different across subjects due to individual characteristics, such as size and shape of the head, skull thickness and other parameters of brain anatomy ( ). However, recent developments based on individuals’ functional or structural scans and computational modeling may allow additional investigations clarifying the correlations between electric field strength delivered by tDCS to specific brain regions of interest (ROIs), the interaction mechanism between this field with the neurons and clinical response. For instance, the simulated electrical field strength in the anterior cingulate cortex (ACC) and left DLPFC has been found to be inversely associated with negative affect and depression scores, unveiling a potential mechanism of action related to tDCS ( ). Despite the limitations and complexity involved in computational modeling ( ), this methodology might ultimately provide grounds for individualized current dosing and electrode montages in future tDCS protocols ( ).
Secondly, the combination of tDCS with cognitive and/or behavioral interventions is also very appealing, as tDCS targets brain areas that are also activated by the latter. This can potentially enhance clinical results through a process known as “target engagement,” in which it is speculated that the effects of tDCS is greatly influenced by the functional state of the targeted cortical region ( ; ). For instance, both tDCS and CBT have been observed to engage the prefrontal cortex and ACC ( ), brain areas which have been previously associated with maladaptive rumination and brooding in depressive states ( ). In a recent pilot study, patients with TRD received active tDCS (20 min, 2 mA applied to the left DLPFC) on eight consecutive days, and were randomly assigned to a mindfulness-based cognitive therapy (MBCT) or relaxation, immediately after the tDCS sessions ( ). In this study, patients who received the combination of tDCS and MBCT displayed better maintenance of clinical improvement ( ). Other previous studies involving the combination of tDCS and cognitive control training in a small sample of patients with MDD have shown mixed results ( ; ). An ongoing multicenter trial evaluating the efficacy of tDCS associated with group CBT ( ) should help shed more light on the probable synergistic effects of NIBS and psychotherapy, in the context of “target engagement” and “dynamic duos” ( ; ).
Finally, the growing use in psychiatric trials of techniques like functional magnetic resonance image (fMRI), single-photon emission computed tomography (SPECT), positron emission tomography (PET), electroencephalography (EEG) and serum levels of several hormones to measure biological markers ( ) may help to clarify the mechanisms of action of tDCS, identify “optimal” parameters of stimulation and eventually guide patient selection more prone to respond to treatment ( ). Among the potential biomarkers that have already been explored, we can highlight baseline resting-state EEG oscillatory activity ( ), polymorphisms in brain-derived neurotrophic factor (BDNF) ( ) and the 5-HTTLPR serotonin transporters ( ), and brain volumes in specific ROIs (for instance, larger gray matter volumes in the left DLPFC at baseline have been associated with better tDCS antidepressant response) ( ). Furthermore, tDCS responders have been found to display greater improvements in dysphoria and retardation symptoms, as compared to nonresponders, offering a more nuanced understanding of what could be expected from tDCS in treatment-resistant samples ( ).
Moreover, the accumulation of big data and the use of a machine learning (ML) approach can also assist in clarifying the gaps in knowledge about the predictive role of biological and clinical characteristics that are associated with better tDCS response in TRD, potentially allowing the identification of preclinical changes which could guide adjustments in stimulation parameters or treatment regimen ( ). To the best of our knowledge, there is no published research using ML to predict tDCS response based on clinical samples ( ).
Conclusions
TDCS is a NIBS intervention that delivers subthreshold electrical currents to neuronal populations, usually via two electrodes, inducing a shift in resting membrane potential, either toward depolarization or hyperpolarization, depending on stimulation parameters.
TDCS has been increasingly investigated in diverse neuropsychiatric disorders, presenting itself as a safe and feasible intervention. The results of recent studies have shown tDCS montages consisting of the positive electrode (anode) over the left dorsolateral prefrontal cortex (DLPFC) and the negative electrode (cathode) over the right DLPFC to be moderately and definitely effective for MDD (level A).
Interestingly, the first trials and reviews with TRD samples showed a negative association with tDCS therapeutic effects. However, recent meta-analyses did not present TRD as a possible predictor of negative response for tDCS. Moreover, a recent guideline considered tDCS as an effective treatment for depression, even for treatment-resistant patients.
Recent studies showed home-use tDCS, individualized dosing, tDCS associated with cognitive and behavioral interventions, the discovery of biomarkers and use of machine learning and big data, as being future directions to help diminish existing knowledge gaps in this most interesting of NIBS modalities.
References
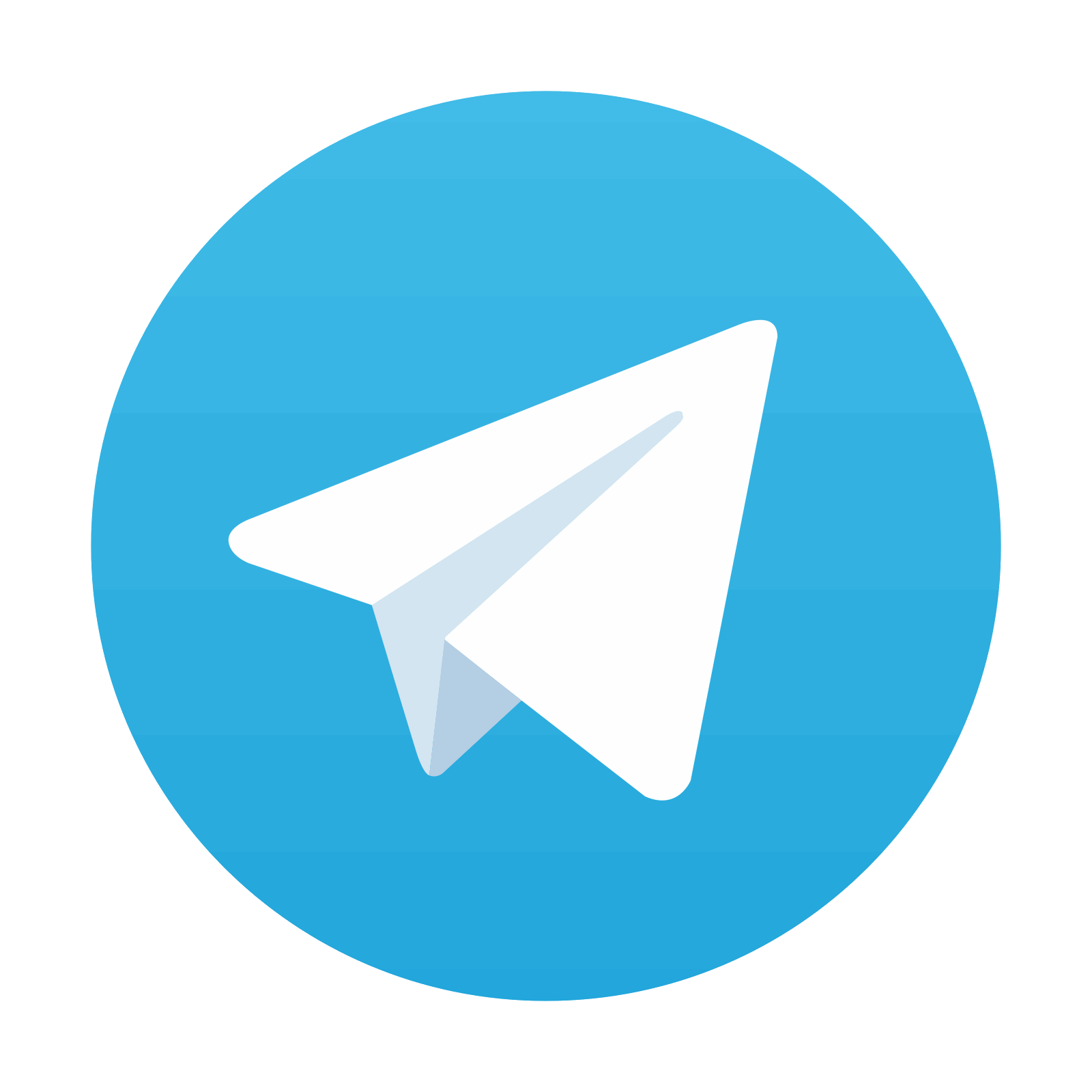
Stay updated, free articles. Join our Telegram channel

Full access? Get Clinical Tree
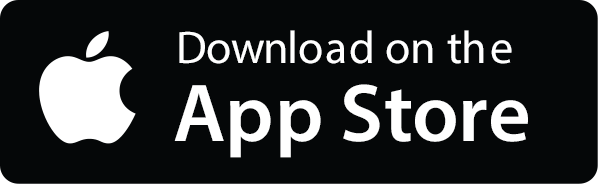
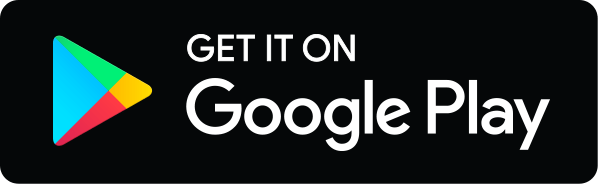
