CHAPTER 2
TECHNICAL ASPECTS OF AMBULATORY ELECTROENCEPHALOGRAPHY
J. ANDREW EHRENBERG, BSC, R. EEG T., CNIM and CHARLES M. EPSTEIN, MD
INTRODUCTION
Ambulatory electroencephalography (aEEG) refers to portable electroencephalography units, capable of prolonged periods of multichannel recording in an outpatient setting that encompasses normal daily life (1). While routine and sleep deprived EEGs are typically 20 to 30 minutes in duration with some ability to capture abnormalities, a normal routine EEG does not exclude a diagnosis of epilepsy (2). Even when interictal abnormalities are present in the routine scalp EEG, long-term monitoring can be beneficial to provide a greater diagnostic yield (3).
While the utility and applicability of aEEG are well described in other portions of this book, this chapter deals with the technical aspects of aEEG. Ambulatory EEG is used as an adjunct to an epilepsy diagnosis; it may also be used to guide treatment in both adult and pediatric patients. Since aEEG will be recorded predominantly outside the controlled environment of a clinical neurophysiology laboratory, it is essential to provide the best initial technical setup possible, because the interpretation and clinical correlation assumes that all technical considerations are optimized during the entirety of the EEG recording time. While prolonged aEEG recordings outside of the laboratory environment (e.g., at home) are a clinically useful tool for a neurologist, these same features make a strong argument for requiring a fundamental understanding of the technological limitations and the underlying concepts of recording even more essential. Computer-assisted ambulatory EEG (CAA-EEG) uses complex computerized microprocessors and electronic components that are designed to provide prolonged EEG recording outside of a sheltered laboratory or hospital environment. Normally, this would allow technical issues to be immediately recognized and eliminated by qualified personnel. During aEEG recording, important technological challenges may arise.
Throughout the history of EEG development, digital technology has replaced and made significant advances over the older analog systems that were initially utilized (4). It is in the field of aEEG monitoring where advances in technology and miniaturization of its components and enhanced memory have made the greatest impact. In fact, modern aEEG systems currently in use bear only a vague similarity to the initial 4-channel systems in the past. Prior to the incorporation of digital aEEG technology, the initial method of acquisition was recording to a cassette tape medium. This method would allow aEEG in addition to the audio equivalent of analog paper EEG. Many do not realize that in general, EEG utilizes some of the essential technological features that are necessary for recording music through translation and reproduction of analog signals. Similar to the initial trends in music development in the 1980s, the desire for a portable format led to the use of portable cassette tape players for music that were subsequently adapted to the same technology to begin aEEG. Similar to the development of portable music devices, the industry evolved from being recorded and played on cassette tape to storage on digital media (e.g., compact disc, MP3 players); CAA-EEG systems also evolved to storage on digital media. While the specifications vary widely from vendor to vendor, most CAA-EEG systems share some common features. It is important to obtain the individual specifications of an aEEG machine that will meet the needs intended for clinical use that is anticipated. Just as the diagnostic question prompting aEEG in the first place must be specific enough to prove useful, so too must the specifications of the machine for the intended use (5).
In this chapter, we explore the technical aspects of CAA-EEG recording. This will include the basic technology of a computer-based EEG system concentrating on the technical aspects relative to aEEG and speculation about the technological changes that could impact devices used for ambulatory EEG monitoring in the near future.
BASIC TECHNICAL ASPECTS OF AEEG
In discussing the technical aspects of aEEG, it is essential to understand the general technical properties that apply to neurodiagnostic procedures that can impact integrity of recording by producing artifact. Functionally, these properties that may produce artifact can be divided into four principal sources during aEEG recording (Figure 2.1):
1. The bioelectrical activity and recording electrodes
2. The differential amplifier
FIGURE 2.1 Basic technical components of an aEEG system.
3. Digitization and storage of the analog electrical waveforms
4. Reconstruction and display of activity for review
Bioelectrical Activity and Electrodes
Electrode artifact is the most common type of artifact limiting the quality of aEEG monitoring. As an integral clinical neurophysiological study utilized for nearly a century, the long-term EEG and aEEG remain best suited for the evaluation of epilepsy. The basic principles used by Neminsky (6) and later Hans Berger (7) have not significantly changed over the years and still lie at the foundation for most electrophysiological modalities within the field of clinical neurophysiology.
The bioelectrical fluctuations of the brain, used to determine normal and abnormal physiological function, are recorded using conductive electrodes. The voltage changes in the brain are attenuated, and to some degree, filtered by the skull and scalp (8,9). As such, the cortically generated activity at the scalp’s surface is significantly smaller than environmental noise. Conductive gel or paste creates an ionic medium between the scalp and the metal of the electrode, creating an “electrical double layer” (Figure 2.2). Most commercially available conductive paste has some adhesive properties and is usually sufficient for routine EEGs in a clinical environment where the patient is not moving and any technical issues can be observed and corrected.
As with any types of EEG chosen for clinical use, electrodes composed of silver/silver chloride and gold are preferred (Table 2.1). The use of disposable, single use electrodes represents the best means of providing infection control in clinical practice (10). The initial impedances of the electrodes should be maintained below 10 kΩ (11), balancing minimal skin abrasion with attention to the least interelectrode impedance that can be achieved. This is essential as skin breakdown is a real concern during prolonged aEEG recording sessions where no upkeep or repair can be performed on a regular basis. Impedances should routinely be recorded at the beginning and at the end of the recording session so that the interpreting physician can assess this in the interpretation of the recording.
FIGURE 2.2 The electrical double-layer outlining the separation and resistance to transmitting electrical charge.
TABLE 2.1 Relative Reactivity Based Upon Metallic Substance Conducting an Electrical Charge
SUBSTANCE | CONDUCTIVITY |
Silver/silver chloride (most stable) | 6.17 × 107 |
Copper | 5.80 × 107 |
Gold (inert, less half-cell) | 4.10 × 107 |
Seawater | 4 |
Rubber | 10−15 |
The conductive and adhesive properties of adhesive pastes are short lived and are therefore insufficient for prolonged recording times. It is important to fix the electrodes to the scalp with some form of approved long-acting skin adhesive such as collodion to ensure recording integrity is maintained throughout the aEEG monitoring session, which is then secured by a Kerlix gauze head-wrap. The extra time taken to ensure adequate interelectrode distances and the added care in how they are applied and then secured to the scalp will help to ensure a technically successful aEEG recording. The interpreting electroencephalographer is best served by becoming familiar with the policies and procedures of performance, the technologists involved with conducting the recording, and technical adequacy or lack thereof of the system utilized in the laboratory during the period of connecting, processing, and annotating the aEEG. It is vitally important that the standard practice of measuring and marking electrode derivations on the scalp for electrode application is understood and consistently adhered to for optimal technical recording. A cartoon of the electrode–scalp interface is illustrated in Figure 2.3 and the actual setup in Figure 2.4.
The limited portability of the early aEEG systems and the limited internal digital memory storage that was previously available resulted in initial 16-channel CAA-EEG recordings. When additional physiological or polygraphic channels were required, the additional information came at the cost of sacrificing the number of channels utilized for EEG. With the technological evolution of digital systems, modern aEEG systems are now easily capable of accommodating 21 channels of recording with some systems able to accommodate up to 32 channels including additional input for extracerebral direct current channels for physiological monitoring (e.g., sleep monitoring). Digital systems, particularly in aEEG, commonly utilize a “system reference” or “machine reference” (4). These inactive reference sites usually reflect the proprietary selection of a midline vertex location due to the central position that is less prone to movement and myogenic artifact. Locations in the vertex midline (such as FCz or CPz) may be used, but care must be taken to avoid a salt bridge with nearby electrodes that “cancel” the bioactive signal (12).
FIGURE 2.3 Cartoon of the initial electrode application and scalp interface.
FIGURE 2.4 Preparation and application of electrodes in a mannequin and human. (Courtesy of Lindsay Ireland, 2011, with permission.)
Differential Amplifiers
The discovery that neural activity could be induced by external artificial electrical stimulation marked the start of modern electrophysiology in 1791 (13). Thirty years later, a method to record changes in electrical current through deflection by a needle led to discovery of the “galvanometer” that formed the basis for recording electrophysiological potentials, ultimately resulting in the first human “electrogram” of the heart (2). Spontaneous electrical rhythms of the mammalian brain were first demonstrated in 1870 by the physiologist, Richard Caton (14). Refining recording techniques gave rise to the string galvanometer following the application to recording the heart rhythms with the electrocardiograph in 1902. Hans Berger subsequently used Einthoven’s string galvanometer to study the electrical mechanisms of the brain, which would later become the method of monitoring brain signals that would revolutionize clinical neurology and neuropsychology research. Today, technology has evolved to implement the use of a differential amplifier with new software designs and smaller compact recording devices that are amenable to aEEG (Figure 2.5
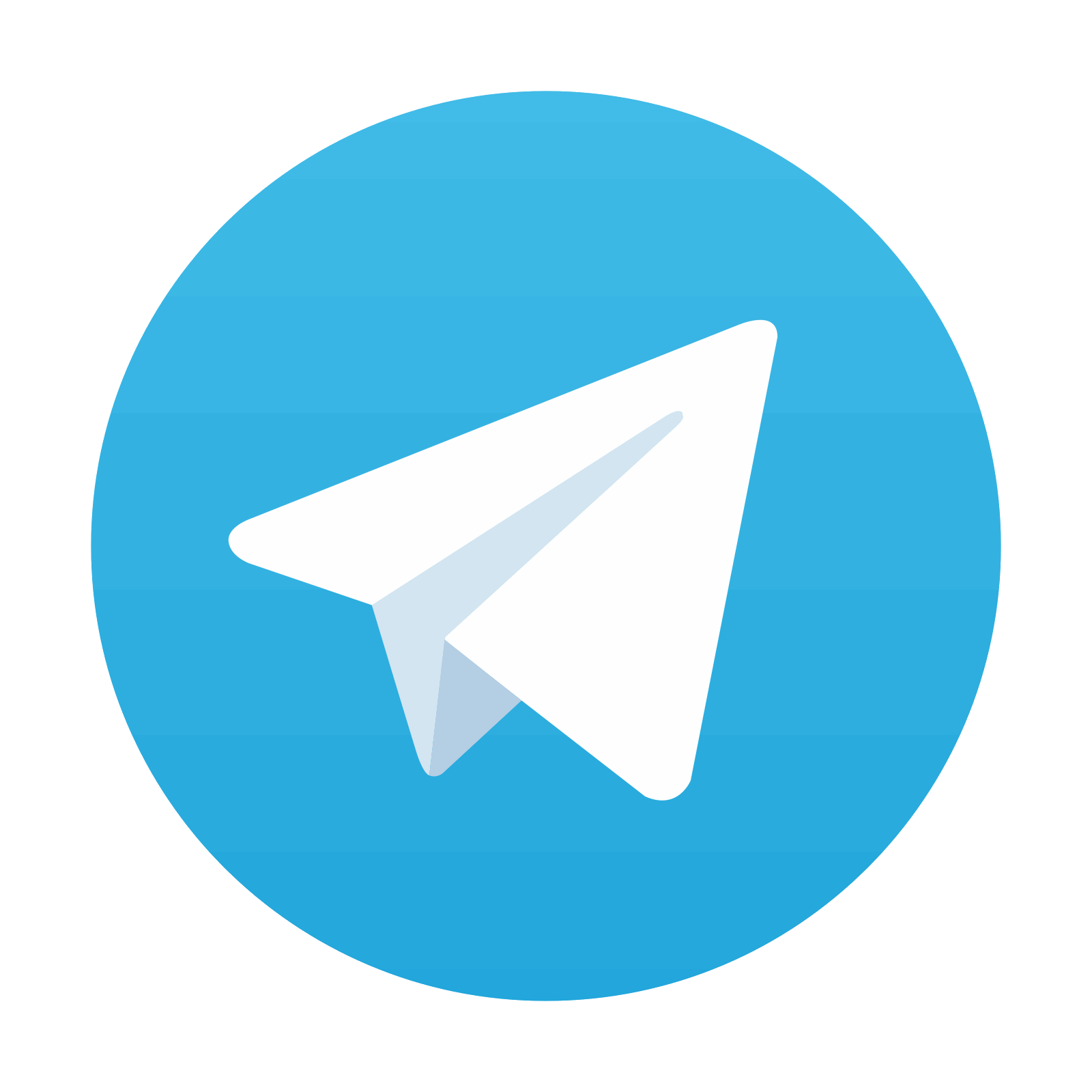
Stay updated, free articles. Join our Telegram channel

Full access? Get Clinical Tree
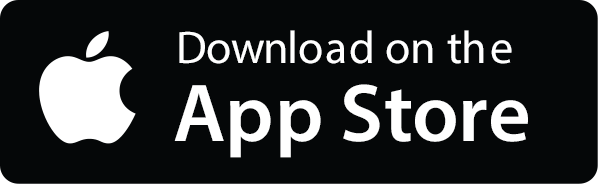
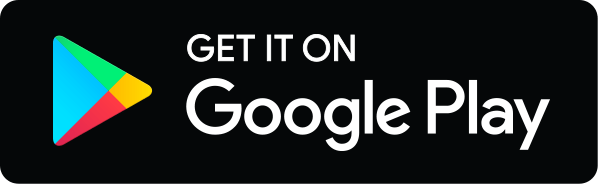