(1)
Department of Clinical Neurological Sciences, Western University, London, ON, Canada
2.1 The Use of Bipolar Coagulation
2.1.1 The Physical Principles of Bipolar Coagulation
There are general characteristics of epilepsy surgical technique that facilitate the accomplishment of satisfactory cortical resection. These are all aimed at increasing the gentleness of the surgery and thereby reducing potential injury from the surgery while particularly providing protection of the juxtaposed cortex at the same time. They include the appropriate use of bipolar coagulation, irrigation, suction, and cottonoid patties. The importance of these characteristics with respect to the quality of all branches of neurosurgery cannot be overemphasized!
One of the most important components in neurosurgical technique is the appreciation of the benefits of good coagulation. Perhaps even more important is the understanding of what good coagulation is and how to achieve it. Physically, the use of bipolar coagulation is characterized by the passage of electrical current between the two poles of the coagulator, e.g., the two blades of the bipolar coagulation forceps. The route, or routes, of current passage will involve whatever conducting material exists between the blades of the forceps. That is to say, any conductive electrical pathway(s), which exist(s) between the blades of the forceps, irrespective of how far they may be from the shortest route between the blades, will conduct some fraction of the current when the coagulator is activated. These pathways consist of any biological fluids or tissue, e.g., cerebrospinal or extracellular fluid, parenchyma, cells, blood, saline, etc. If there are a number of such pathways, then they are said to be in parallel. That is to say, they are parallel to one another, and, as such, they will all conduct some part of any electrolytic current passing through the area from one blade of the coagulating forceps to the other. In other words, they will share the available current to varying degrees. There are a number of variations, but the primary one consists of the resistances of the various segments of the current pathway. The larger the resistance (i.e., the lower the conductance) of a given segment, the smaller will be the share of current traversing that segment. Conversely, the greater the conductance of a particular pathway, the greater will be the share of current traversing that pathway. Thus, the reader will appreciate that the ideal preparatory achievement when using coagulation is to isolate the tissue that is to be coagulated, to as great an extent as possible, such that as much as possible the current will in fact travel through that targeted tissue, e.g., a blood vessel in most situations. There are a number of aids that can be utilized to achieve this isolation, which can be continually emphasized further on in this section.
If the conducting surfaces of the bipolar forceps are large, then the amount of current flowing between any two points of the pair of forceps, at a given strength of current, is small. This is illustrated in Fig. 2.1a. In this illustration, the current is equally distributed through the entire conducting medium that is in contact with the metal surfaces of the two bipolar forceps. Thus, if a small blood vessel was placed anywhere in the pathway under the circumstances outlined in the figure, only a very small amount of current would travel through the blood vessel, per se. The remainder of the current would travel through the other segments of the conducting medium, providing no help in coagulating the targeted tissue for the coagulation. This wasteful conduction of current is often referred to as the shunting of current, i.e., shunting it away from the primary pathway through which the proposed coagulation is aimed.


Fig. 2.1
Current pathways involving insulated and non-insulated bipolar coagulation forceps. Potential current pathways in the use of bipolar forceps, at a given strength of coagulation, without insulation (a) and (b) those with insulated forceps blades (except for the internal opposing surfaces of the tips). In the latter, the current pathways are more compact and more concentrated in the vicinity of the small non-insulated areas at the tips of the forceps. The amount of the current is proportional to the illustrated thickness of the theoretically depicted pathways. bip.f bipolar forceps, c.p. conducting pathway, ins insulation on forceps, n-ins non-insulated internal tips of the forceps
Figure 2.1b depicts the situation in which the illustrated elements are the same as in Fig. 2.1a except that the blades of the bipolar forceps are insulated except at the tips of the blades. Thus, all the segments of the current pathway travel between the blades and hence are concentrated between the two blade tips, the only parts of the forceps lacking insulation. With large areas of the forceps blades insulated, there is a significant reduction in the large amount of current (as in Fig. 2.1a) that is simply shunted away from the vicinity of the opposing tips, where the coagulation is to take place. Figure 2.1b also exhibits what was noted earlier that any potential bona fide segment of the pathway that is capable of carrying current would do so. In Fig. 2.1a, the current segments traveled the same distances between the non-insulated forceps and hence had equal shares, or fractions, of the current. Figure 2.1b illustrates that the farther away a segmental path is from the conducting metal points, the smaller is the fraction, or share, of the current it accommodates, depicted by thinner lines of the farther away (from the points of the forceps) segments of the pathway. In this situation, all of the current travels between the two small, non-insulated tips of the coagulating forceps, and thus nearly all of the current will follow a pathway through the tissue on either side of which the forceps tips are placed. Further, it can be appreciated that the shunting of current noted in the immediate foregoing can be largely abolished by removing the excess conducting medium around the targeted tissue. This will be addressed further on (vide infra).
Not only might the number of pathways be of importance in coagulation, but the composition of the conducting pathway will also influence the amount of current carried by the potential segments of the pathway. If the composition in a single pathway, for example, is a simple electrolyte solution such as saline, then the current will simply travel through the solution as a homogeneous electrolytic current, which is the same at any point or segment within the pathway, because the resistance is uniform throughout the saline solution. [An example of this is the function of a kitchen toaster, i.e., all of the heating coils are the same thin, equally resistant wires. Thus, the heat generated by the total matrix of wires is homogeneous throughout.] In such a circumstance, if the current is gradually raised, then a level will be reached where the current is sufficiently strong to create enough thermal energy to bring to the boiling point the immediate environs between the tips of the forceps, i.e., this is reflected in bubbles at the tips of the forceps. In a given current pathway, the amount of current required for this phenomenon to occur is related, in a positive correlation, to the resistance of the pathway. The generation of the bubbles may produce sufficient amplitudes of mechanical perturbations (vibrations) in that the experienced surgeon can physically appreciate the mechanical, vibratory transmissions up through the handles of the forceps! (This is usually not appreciated until a few seconds after the onset of the current, especially in using lower current strengths, which will be discussed in later sections, e.g., Sects. 2.1.6 and 2.1.7.)
Nearly all biological tissues have electrolytic components, as well as individual intrinsic resistances to the conduction of electrolytic current; the resistances are dependent upon the characteristics of the conducting quality of their physical makeups. In keeping with an earlier similar explanation, when there is a combination of biological tissues between the bipolar forceps during the process of coagulation, then each tissue will conduct a share of the coagulating current; each share will be inversely related to its intrinsic resistance. If within a given single current pathway there are components with varying resistances, then these components are said to be in series along this pathway. If the components have different resistances, then the heat generated within a given segment will vary depending upon the resistance of the component; the greater the resistance of a given component, the greater will be the heat generated by a given current traveling through it. For example, components containing protein, e.g., tissue such as blood, blood vessels, meninges, and brain parenchyma, have a much greater resistance to current flow than do solutions that are primarily electrolytic, such as saline and pure extracellular fluid (including cerebrospinal fluid). Because of their increased resistances, the flow of current through these protein-containing components will result in the generation of greater quantities of heat than in those components consisting primarily of a simple electrolyte. Contrariwise, the pathways, which have smaller resistances, will conduct greater amounts of the total current. This is depicted in Fig. 2.2.


Fig. 2.2
Differential resistances of tissues within a theoretically single segmental current pathway between the bipolar coagulation forceps. (a) Exaggerated separation of the forceps blades, exhibiting a theoretic single pathway, through which current is flowing, which consists of varying components, with different resistances, within the pathway. (b) The inferior part of the diagram depicts the heat generation in the different components of the current pathway. bip.f. bipolar forceps blades, bl blood, bl.v (blv) blood vessel, br.p. (brp) brain parenchyma, csf, cerebrospinal fluid, ecf. extracellular fluid, R resistance, S saline, R blv, R bl, R brp, R ecf, R s, and R csf represent the resistances of blood vessel, blood, brain parenchyma, extracellular fluid, saline, and cerebrospinal fluid, respectively, where R blv > R bl ≈ R brp > > R ecf ≈ R s ≈ R csf (see text)
Figure 2.2a depicts a theoretically pure single current pathway with a composition of a variety of potential tissues and fluids through which the current passes. Rough approximations of the resistances of the various fluids and tissues are depicted by the height of the resistance symbols. Figure 2.2b is simply a reflection of the generation of heat as a result of the current flowing through the various resistances.
Given the foregoing discussions, the ideal situation for the achievement of satisfactory coagulation is when the pathway through which the electrolytic current passes contains primarily the tissue targeted for the coagulation. Taken a step further, the absolute ideal environment of a current pathway, then, would consist of targeted tissue being the only significant coagulable component in the pathway; more often than not, this would consist of a bleeding point. Thus, in this situation there would be no excess blood or excess saline or other electrolytic fluid irrigation in the current pathway, under which circumstances the proteinaceous blood vessel can be broken down and destroyed, leaving behind the residual coagulum of that tissue. To again reiterate an earlier notation, if saline irrigation can be utilized to dilute, or clear, the pathway of potentially coagulable substances, except that which is to be coagulated, then the quality of the coagulation event is optimized!
2.1.2 The Separation of the Blades of the Bipolar Coagulating Forceps
Section 2.1.1 was directed purely at the consideration of the environment between bipolar blades during the use of coagulation. This section is simply a brief consideration regarding the distance between the blades of the forceps during coagulation, and as such it is somewhat redundant. I have chosen to accept the redundancy as a number of overlapping features lead to satisfactory coagulation. No surgeon is unaware that if the blades of the forceps touch one another, then there is no resistance to the flow of current between the blades. Thus, quite irrespective of what other pathways might exist, all of the current will flow directly from one blade to the other, leaving none to traverse the tissue targeted for coagulation, which, of course, has resistance. In this case, the current is purely an electrical current between the two forceps blades, i.e., there is no, or insignificantly little, intervening electrolytic current passing through tissue.
The ideal separation will be the smallest that can be maintained without the blades actually contacting one another on the one hand but yet on the other hand as close together as possible in order to include primarily the bulk of the tissue to be coagulated. This ideal separation will provide a pathway through which the majority of the current traverses this tissue, thereby minimizing the amount of current necessary to achieve the coagulation. The minimization occurs through optimally reducing the amount of current that is shunted through pathways, which does not contribute to the coagulation of the tissue in question. This is depicted diagrammatically in Fig. 2.3. Figure 2.3a demonstrates forceps blades that are too far apart and, additionally, an excess of (conducting) fluid between them. In Fig. 2.3b, the excess fluid has been largely removed, but the blades remain too far apart. As a result, there will still be some wasteful shunting of current on either side and around the blood vessel. Figure 2.3c illustrates the ideal—the removal of all the excess fluid and the marked increased reduction of the separation of the forceps blades. Both of these alterations reduce the amount of current required for the achievement of a simple satisfactory coagulation.


Fig. 2.3
The effects of separation of forceps in bipolar coagulation. Diagrams demonstrating the advantages of reducing the separation of bipolar coagulating forceps blades to a minimum; (a) illustrates forceps blades that are too far apart and with an excess of fluid between the blades and around the blood vessel; (b) is the same diagram as (a), but with the excess fluid having been removed (thus a reduction of unnecessary shunting current); (c) shows the blade separation reduced to a minimum thus reducing the distance of the current pathway between the blades and insuring that within the pathway the primary composition is the targeted tissue (e.g., blood vessel, parenchyma, etc.). bip.f. bipolar forceps, sal saline (see text)
Blood flowing within a blood vessel will carry away varying amounts of heat surrounding it. If it is a bleeding vessel that is being coagulated, then the separation of the blades of the bipolar forceps should be small enough to obliterate the flow, thereby providing static blood inside the vessel, which is much more easily coagulated than flowing blood (vide infra).
2.1.3 Saline Irrigation
In keeping with the tenets of the foregoing, the ideal coagulating pathway consists of pure electrolytic components with the only exception being that of the component to be coagulated. As noted in the foregoing, saline irrigation, or irrigation with some other equivalent electrolyte, aids in achieving this by primarily washing out other components, whose coagulation would be simply unnecessarily shunting current away from the tissue being coagulated. The primary other component in most instances is blood. If there is blood along the pathway, then it will absorb current and heat and will elevate the requirement for the necessary current to coagulate that at which the coagulation is being aimed. Thus, adequate saline irrigation will keep the pathway an electrolytic current pathway, except for what is being coagulated. Its use, of course, is only helpful if an excessive amount of the saline (electrolytic) solution is not left in the environment of the current pathway.
In summary, gentle saline irrigation of the site of coagulation facilitates the removal of proteinaceous-containing substances that may unnecessarily raise the required strength of current to achieve the satisfactory coagulation of the tissue to be coagulated; its effect is by virtue of providing a better quality environment for the conduction of electrolytic current. This abolishes the case of high current and increased amounts of blood that leads to a coagulum on the forceps, which is associated with all the aggravations well known to the neurosurgeon!
I would be remiss in not acknowledging the irrigating bipolar forceps. For the surgeon working alone or without an experienced assistant, there is no adequate substitute for a good pair of irrigating bipolar forceps with the irrigation regulated to just provide the appropriate saline moisture at the end of the forceps during the course of significant requirements of ongoing coagulation.
2.1.4 Suction
Little needs to be documented with respect to the importance of suction, other than as it pertains to optimizing the physical quality of the coagulating current. It obviously must be gentle enough not to damage the tissue immediately surrounding the point of suction, e.g., also one of the strategies to reduce postoperative lesions arising from the surgery. However, from the physical aspect, the suction is important in reducing any excess electrolyte solution, which has been utilized to cleanse and to keep moisturized the area to be coagulated. As noted in the foregoing, this applies also to other tissue, the coagulation of which is not germane to the desired tissue to be coagulated. Leaving excess saline fluid around this area, if it contacts both blades of the forceps, will electrically provide in parallel pathways (see Figs. 2.1 and 2.3a), which will shunt current through it without making any contribution to the desired coagulation. Since the electrolytic (saline) pathways have less resistance than that which involves the point to be coagulated (which will be protein in content), the former may conduct the majority of the current, and the latter will receive insufficient current to effect the appropriate coagulation in much the same way as noted in the foregoing section which dealt with the separation of the blades of the coagulating forceps. The removal of this excess saline has the same effect as narrowing the separation of widely separated forceps blades, i.e., it minimizes the loss of current through noncontributory pathways (illustrated in Fig. 2.3a, c). Once again, the importance of the gentleness of the application of the suction cannot be overemphasized.
In summary, during normal epilepsy surgical resection, the pressure of the suction should be lowered such that it will not damage tissue to which it might be applied, when the outlet hole on the handle of the suction cannula is uncovered. Given this as the starting point, then the regulation of the force of suction may be controlled by gently rolling the thumb over the outlet hole. At the same time, any unwanted potentially injurious event from too much suction can be prevented instantly by rolling the thumb off the hole.
2.1.5 Cottonoid Patties
The use of cotton patties in any neurosurgical procedure has many attributes. One of these is its protective effect during the use of higher levels of suction without traumatizing the underlying brain parenchyma. For example, there are unusual cases when the suction must be raised to high levels, e.g., high enough to damage brain tissue if applied directly to it. A typical such case involves the bleeding that may occur during the removal of an arteriovenous malformation and which can be sufficient to preclude satisfactory vision of the underlying operative field. The safety to underlying brain parenchyma can be achieved through the use of cottonoid patties. The use of a cottonoid greatly increases the efficiency of removing only the blood, of providing easier visual access to the bleeding point(s), and of significantly reducing the potential of damaging brain parenchyma or small fragile vascular vessels.
In addition to the foregoing, the use of suction applied to cotton patties allows the safe application of pressure for the occlusion of the flow of blood from bleeding vessels. The object is to achieve a bloodless field in which to conduct optimal coagulation. Bleeding arteries, if sufficiently large, may be impossible to coagulate without significantly reducing or abolishing its flow at the point from which it is escaping. In such a situation, if the flow is not stopped or at least significantly reduced, it may simply carry away enough or all of the localized heat generated by the coagulation current, thus precluding a heat-generating coagulum from forming in the vessel’s lumen and halting the flow of blood. As noted earlier, once the blood is static, it can be easily coagulated with the result of a significantly sized coagulum.
Under this circumstance, I have witnessed surgeons attempting to control the situation by either increasing the force of suction, in order to remove the blood, or the strength of the coagulation current, in order to provide enough heat to effect coagulation, or both. Both of these require a change in at least one of the technical essentials at this point in the surgery. Both of these potential changes may eventually aid in achieving coagulation of the bleeding vessel. However, I believe very strongly (!) that both of these are not only completely unnecessary but require more time (adjusting the required alterations at the time and then readjusting them back again when the bleeding is controlled) and are also much less likely to achieve a satisfactory coagulation. This can usually be rectified by either significantly reducing or obliterating the blood flow through the very safe use of pressure, applied by the suction cannula on a cottonoid patty over the bleeding arterial vessel. This is demonstrated in Fig. 2.4. In Fig. 2.4a, an appropriately sized cottonoid patty is placed over the end of the bleeding vessel, and Fig. 2.4b simply demonstrates the suction cannula being pressed down with sufficient force to collapse the lumen of the vessel, thus stopping any flow of blood. In response to the occasional neurosurgical resident or fellow saying “but won’t your way of dealing with the bleeding vessel take more time?” (i.e., the very few seconds longer!), I have no concern about saying Yes. The reason for this lack of concern will become clear when seeing the section on current strength (vide infra, Sect. 2.1.6).


Fig. 2.4
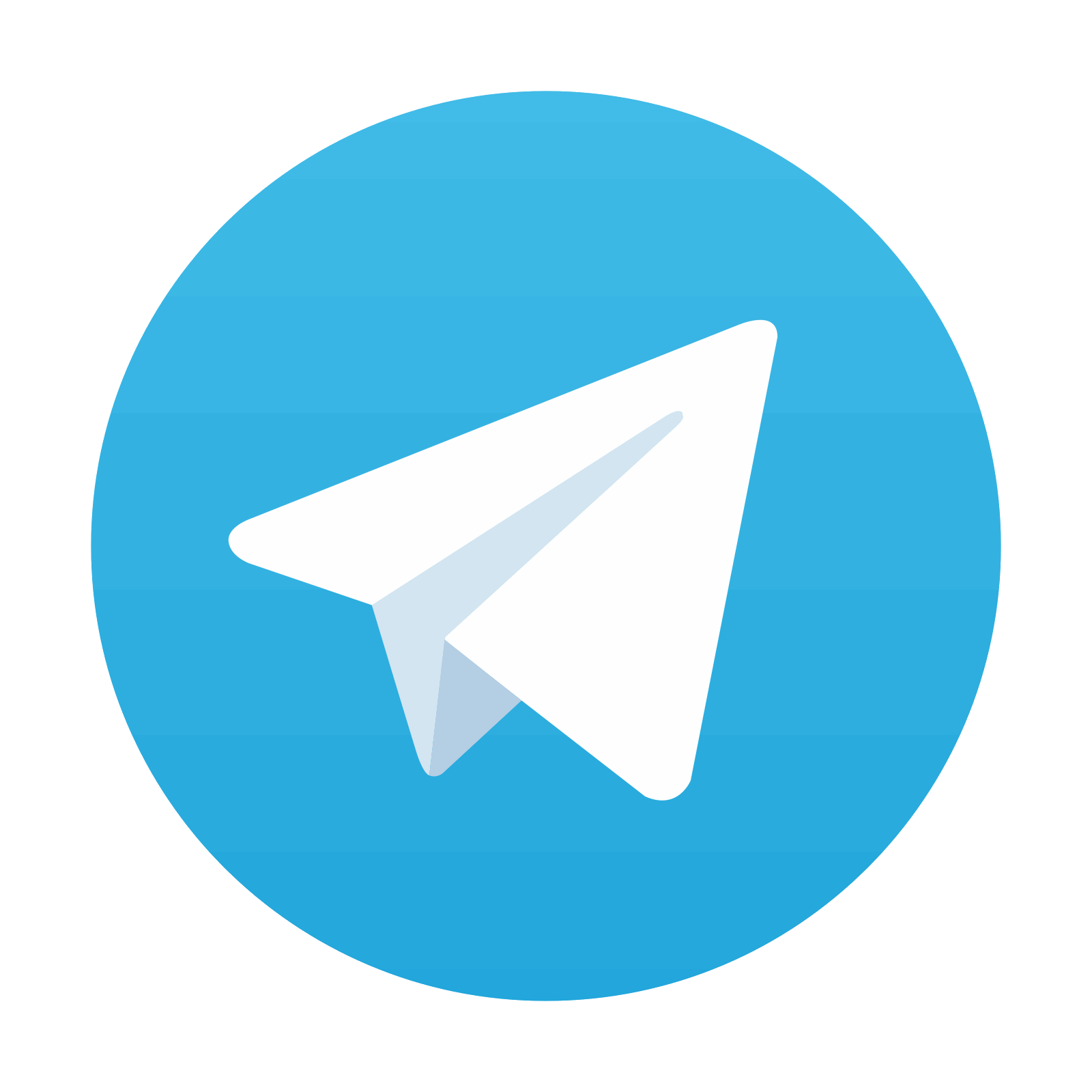
The effect of flowing blood on the quality of coagulation. This illustrates the obliteration of the flow of blood through a bleeding vessel by the application of sufficient pressure applied to the vessel through the use of a suction cannula on a cottonoid patty. (a) The application of an appropriately sized patty over the bleeding point. (b) The application of sufficient force of the suction cannula on the patty to occlude the bleeding foramen
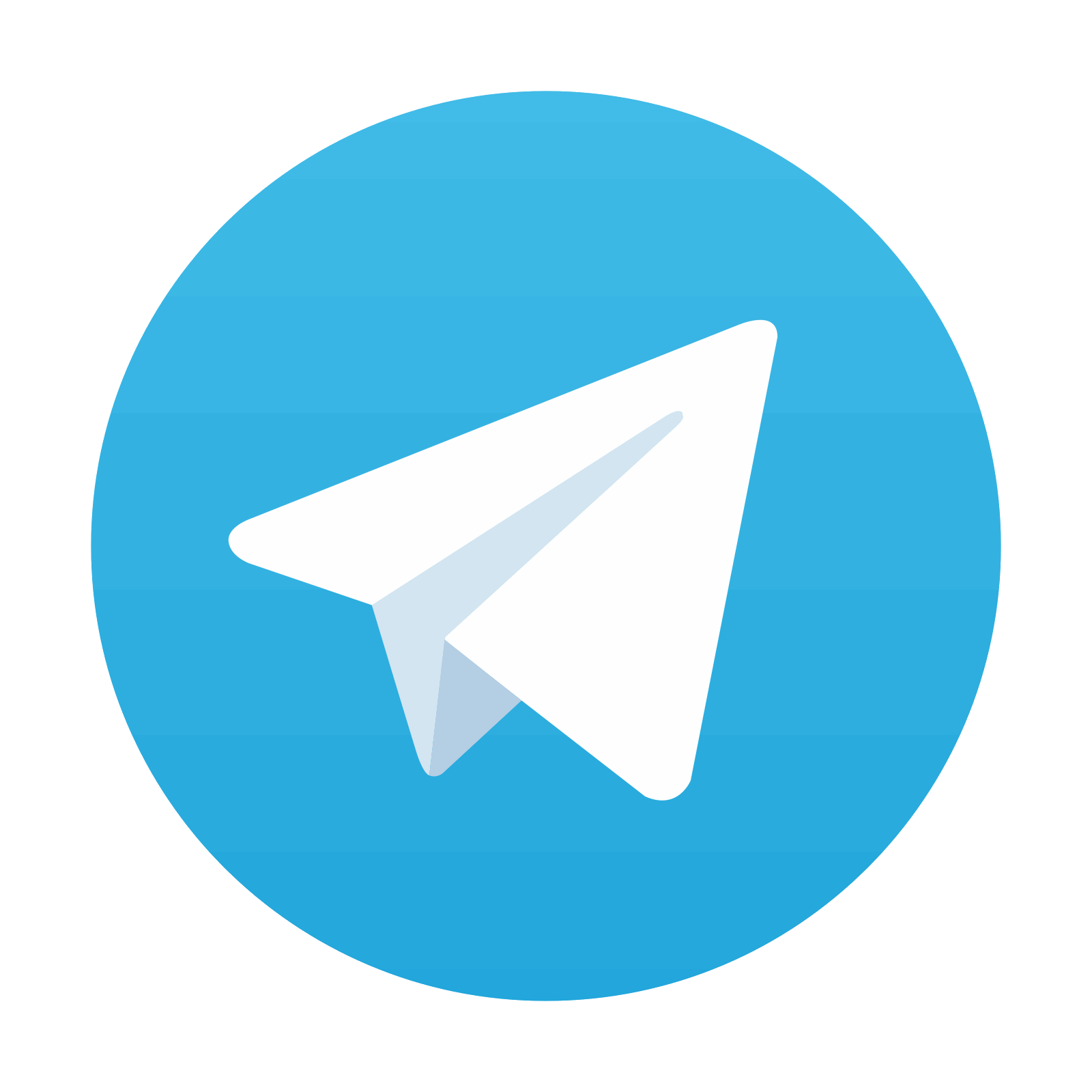
Stay updated, free articles. Join our Telegram channel

Full access? Get Clinical Tree
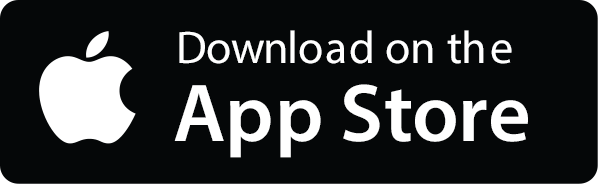
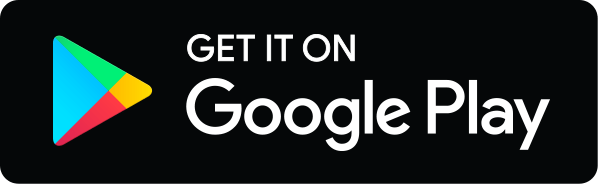
