A review of the effect of treadmill training on CP population by Willoughby et al. (2009) showed augmented speed of over-ground walking measured during a 10 m walking test (10 mWT) and improvements in gross motor skills (evaluated by means of Gross Motor Function Measure—GMFM). Moreover, walking endurance (measured with the 6 min walking test—6MWT) obtained some mild increase only in the more impaired group of subjects (with Gross Motor Function Classification System—GMFCS III or VI) [9]. The review by Damiano et al. (2009) confirmed the results highlighting that many studies noted positive, yet small, effects with a significant increase in self-selected gait speed after BWSTT.
Nevertheless, insufficient evidence is found for CP patients exercised with treadmill training, [10], and some drawbacks that can be highlighted include that the trunk and the lower limbs are difficult to control during exercise; thus BWSTT demands high physical effort especially for severely impaired subjects, [11]. In addition, treadmill training is conducted on artificial walking surface and neuromuscular feedback and sensation are different compared with over-ground walking, [8].
In this framework robotics is emerging as a leading technology for motor rehabilitation of subjects with neurological impairments and, in particular, for recovery of walking. In fact, Robot-Assisted Gait Training (RAGT) has some possible promising advantages with respect to traditional training or BWSTT including the fact that it is intensive, controlled, repetitive, and provided with goal-oriented tasks that are known to be related to the cortical organization and motor learning process, [12]. These are particularly important for the pediatric population that could obtain better results thanks to their greater neuroplasticity. Moreover, it can be performed in a safe manner and allows hand-free operation by therapists, which in traditional therapy has a high physical burden. Finally, robotic rehabilitation is often delivered in conjunction with virtual reality (VR) resulting in cognitive engaging tasks that stimulate the subject’s active participation, [13].
2.1 Clinical Results
Interesting results were obtained with RAGT on 999 stroke adult subjects, [14] and on SCI, [15], assessing significant improvement after RAGT, and also with respect to traditional treatment and BWSTT. Only a few studies assessed the positive effect of robot-assisted lower limb training on the pediatric population, [16].
Till date, few robotic devices are available for the pediatric population and, among them, two are primarily used: Lokomat (Hocoma AG, Switzerland) and Gait trainer GT I (RehaStim, Germany), [16]. These robots follow two different training principles: the first principle provides training with a driven orthosis that guides the lower limb in a sequence of gait cycles on a treadmill. The second uses the end-effector paradigm: the lower extremities are fixed on two moving plates that are moved in a sequence similar to the gait cycle. During the training, both provide the body weight support allowing the fruition of training for subjects with different levels of impairment.
Some positive results on the CP population are reported in the literature. Borggraefe et al. (2010) showed positive effects with 12 sessions of training with Lokomat and described improvements in standing and walking ability (dimensions D and E of the GMFM, respectively) in 20 children with bilateral CP, which were maintained after a period of 6 months, [17, 18]. The authors also reported a dose-dependence efficacy of the intervention as the improvements of the task specifically trained (walking) measured in dimension E of GMFM are positively correlated with higher distance and time walked. Lokomat has also been used by Meyer-Heim et al. (2009), who obtained some significant improvements in terms of 10 m walking test (10 mWT) and dimension D of GMFM-66 after 12–20 sessions of training 22 CP children, [12].
Two randomized controlled trials studied the difference between robot-based therapy and conventional therapy with Lokomat (20 sessions), [19] and Gait Trainer (10 sessions over 2 weeks), [11]. Druzbiki et al. (2013), [19] recruited 52 CP subjects that underwent 20 sessions of 45 min each with either Lokomat or traditional physiotherapy (N
26 each). They observed only few improvements (not statistically significant) in spatiotemporal parameters and kinematics of gait analysis both in the study group and in the control group. Differently, Smania et al. (2011), [11] analyzed the results of 18 diplegic and tetraplegic CP trained with 10 sessions of 40 min each. Nine children of the study group underwent 30 min of RAGT with Gait Trainer
10 min of traditional training while nine of the control group had 40 min of traditional training. Results assessed significant improvements in terms of 10 mWT and 6 min walking test (6 MWT) only in the study group, while no groups gained significant improvement in an index of activities of daily living (Functional Independence Measure for Children—WeeFIM). The controversial results of these studies suggest that the RAGT therapy seems to be ineffective in modifying motor strategy consolidated in chronic disorders like cerebral palsy, thus the gait analysis cannot highlight modification in the kinematics pattern. However, improving muscular strength or reduction of energy expenditure could intervene in obtaining the overall effect of augment speed or endurance during walking observed in other studies, [11, 18] sustainably. The results remain uncertain, thus they should be regarded as preliminary with further studies necessary in RAGT in the CP population.


2.2 Rehabilitative Factors
2.2.1 Subject-Specific Responsiveness to RAGT
Recently, some evidence on pediatric treadmill training suggests a possible heterogeneity in the response to task-specific therapies in children, [20]. In particular, some studies assessed possible different outcomes following training for subjects with different impairment levels at baseline [9, 18, 20].
Borggraefe et al. (2010) observed that patients with moderate to severe cerebral palsy achieved less improvements after the robotic training compared to mildly affected patients. Schroeder et al. (2014) suggest that gross motor function at baseline can be considered as an independent determinant of improvement in GMFM-66 total score and GMFM-E score, meaning that patients with higher motor abilities at baseline improved more during RAGT than patients with lower gross motor abilities at baseline, [20]. These results are in line with findings by Hanna et al. (2008), who observed that CP patients with GMFCS levels of I and II exhibit a higher potential to gain motor function over time compared to severely affected patients using developmental curves of GMFM-66, [21].
However, a different trend was highlighted in a review for BWSTT: greater benefits were gained by children with more severe functional involvement (GMFCS III and IV), [9].
This inconsistency might be related to the heterogeneity of the studies analyzed (the first two related to the use of RAGT and the third one to BWSTT) and the use of different outcome measures to analyze data. It should be noticed that even if children with severe walking impairment (GMFCS IV) may be expected to obtain only reduced changes in functional abilities after training, they may obtain other potential benefits that can have an enormous impact on the children’s health and well-being, [9].
Schroeder et al. (2014) provided a wider evaluation of the patient-specific responsiveness to RAGT, [20], considering also other factors that could influence the recovery (age, gender, etiology, and add-on botulinum toxin therapy). In their study they recruited 83 children (aged between 4 and 18 years) with various developmental disorders (bilateral spastic CP, unilateral CP, ataxic CP, hereditary spastic paraparesis, and genetic syndrome). The patients underwent 12 sessions of training within 3 weeks with Lokomat and were evaluated before and after the training by means of GMFM, obtaining some improvements in GMFM-66 and GMFM-E. The correlation between the results obtained and the other factors considered revealed that age seems to have an inverse effect on the improvement of standing abilities (GMFM-D), while no correlation was found between GMFM, gender, etiology, or previous intervention with botulinum toxin treatment.
2.2.2 Effects of Enhanced Active Contribution on Clinical Outcomes
Active participation of the subjects involved in training is recognized as one of the more important determinants of positive outcome, [22].
Some research groups investigate it analyzing the muscular activity during robot-assisted locomotion. Two studies assessed that the EMG activity of quadriceps and hamstrings is reduced with robot-assisted training with respect to therapist-assisted treadmill training [23, 24], but this difference is reduced if during RAGT subjects were vocally encouraged to maximize their effort, [24]. Schuler et al. (2013) observed that muscular activity follows a more physiological activation timing with respect to training on treadmill without orthosis, [25]. The reduced active contribution during robot-assisted training could be one of the responsible facts for the controversial results described in the literature, as it is recognized as a principle factor in eliciting performance improvements, [22].
Virtual reality has been suggested as an effective means to encourage subjects’ motivation and active participation during training, especially in the pediatric population. Evaluation of EMG patterns on nine children with motor impairment and eight healthy subjects showed that there is an increased EMG activity during tasks with virtual realities than during normal walking conditions for both groups, [26]. These results are confirmed by two other studies, [25, 27] that, robot assisted treadmill training showed that the EMG activity of the hip muscles in the swing phase is significantly correlated with the presence of virtual reality, together with the encouragement provided by physiotherapists.
To conclude, RAGT seems to be a promising strategy to provide rehabilitation treatment in children affected by cerebral palsy and its effectiveness may be enhanced in the presence of active participation, promoted by therapists and/or virtual reality.
Definitive conclusions about RAGT cost-effectiveness cannot be drawn. Crucial elements to be considered for future studies include the small sample size, especially on the pediatric population, the absence of a randomized control trial design, and the lack of instrumental evaluations. There is no clear evidence of benefits of the robot-assisted training in cerebral palsy, also with respect to traditional training. Explanation for this might be due to different methods and protocols during intervention/group of patients studied as there are no well-established protocols shared by clinics to provide training. Moreover, it should be considered that there are only few effective assessment methods able to identify possible variation after training in a quantitative and not operator-dependent fashion. Changes to body structure and function (e.g., muscle tone, energy expenditure, muscle strength, bone density) are not often considered although they could be critical to provide a comprehensive evaluation of the training. Finally, some studies suggest that the effects of training are patient-dependent and a lot should still be done to identify specific factors that allow for prediction of the training efficacy and that could provide important indications to clinicians to customize the rehabilitation treatment.
3 Biofeedback as Rehabilitation Tool Using Physiological Sensors
Biofeedback, for use in treatment of children with cerebral palsy, can be defined as the use of sensory feedback through which objective performance observation related to a specific motor task is presented to provide the child with immediate, consistent feedback of performance, [28]. The aim of providing patients with biofeedback during exercise is twofold. First of all, to improve the effectiveness of the rehabilitation treatment, both by allowing patients to adjust their movements according to the feedback of performance and by providing an incentive to exercise. In the second instance, recording the physiological parameters to be fed back to the patient provides quantitative monitoring and documentation of patient progress during treatment. The latter feature is particularly important when the rehabilitation treatment is extensive and prolonged, which is typically the case with patients with CP.
3.1 Underlying Mechanisms
The neurological mechanisms underlying biofeedback training are still not completely clear. One of the primary problems for children with abnormal movement may be inefficient sensory information. Harris postulated that biofeedback devices that provide augmented or exteroceptive sensory information can be used by children with cerebral palsy to better calibrate the proprioceptive information they receive and, therefore, help them to achieve improved motor control, [29]. Biofeedback may enhance neuralplasticity by involving auxiliary sensory inputs, thus making it an appropriate tool for neurorehabilitation.
3.2 Biofeedback Modalities
Modalities of biofeedback are diverse and the appropriate sensors to use in a biofeedback system depend on the motor control mechanism, the training task, and the therapeutic goal. Clinicians may use sensors that detect such parameters as brain waves, muscle activity, reaction forces, joint angles, or positions. In neurofeedback training, surface sensors are placed on selected areas of the scalp to record EEG activity, thus teaching participants to control the frequency content of the EEG signal and gaining self-regulation of brain functions, [30]. For EMG training, surface EMG electrodes are attached to the skin over the muscle(s) being targeted. The goal of the EMG-based biofeedback is generally to provide subjects with enhanced information about their muscle activity to improve basic motor control skills, coordinated recruitment of synergistic muscles, or functional use of an impaired muscle during daily activities, [31]. Force platform biofeedback systems are used to measure the ground reaction forces generated by a body standing or moving on them. These systems are usually employed in protocols aimed at enhancing stance symmetry, steadiness, and dynamic stability, [32]. Inertial measurement units (IMUs) are systems typically based on accelerometers and gyroscopes that have been used to examine and quantify human movement, [33]. Because of their small and unobtrusive dimension, they have been used in several biofeedback protocols during static and dynamic balance training, [34, 35].
Since biofeedback therapy always involves a monitoring instrument capable of providing accurate physiological information, new and innovative sensor technology is particularly important in order to provide participants with a significant, accurate, and low-latency clue, thus improving the training outcome.
3.3 Early Studies
Starting from the 1970s, scientific studies investigated the effects of biofeedback therapy on the treatment of motor deficits in cerebral palsy. In an early work, Wooldridge and Russell, [36] tested a mercury-switch device to provide 12 cerebral palsied children with auditory and visual information regarding the spatial position of the head. It was concluded that the head position trainer was effective in the development of head control and position awareness in children with cerebral palsy. Postural control was also investigated in another early study, [37], where a pressure switch that activated a videocassette recorder was placed in the seat insert of five children with spastic diplegia or quadriplegia with inadequate trunk control. Based on the amount of time they exerted pressure against the switch, the study showed that children improved their sitting posture by voluntarily extending their trunk. In one pioneer study, Nash et al. [38] used the gain of the tonic stretch reflex of the gastrocnemius muscle derived from the level of EMG activity while the child’s joint was rotated by the operator, to control video games. The protocol was tested on three spastic diplegic children with normal intelligence and aimed at facilitating the control of the reflex sensitivity, thus reducing spasticity. They reported that the range of voluntary joint rotation increased significantly, but that only one subject had a significant reduction in spasticity. They also highlighted that the protocol made the training interesting and enjoyable for all the children.
3.4 State of the Art
Early approaches have several limitations that prevent long-term use in children. In the first place, the types of cues used to convey the information to the subject were relatively simple, usually employing analog, digital, or binary values. A common form of performance information employed response-contingency systems, in which a desirable event such as the operation of a television set occurs as long as the required activity occurs, [28, 36]. Such feedback requires attention and can be distracting to the child and to other children nearby, preventing its use, for instance, during school. To have a significant effect on brain plasticity, it seems desirable to have the child training for several hours a day during daily activities. To address this limitation, Bloom and colleagues, [39] developed a wearable device that provided the subject with a mechanical tactile stimulation. The device was based on a mechanical vibrating stimulator attached to the skin, which provided the patient with a vibration proportional to the activity of the most impaired muscle and it was tested on 11 cerebral palsy children during daily activity. Results, based on parental questionnaires and Goal Attainment Scale assessments, showed significant clinical improvements in all the children who completed the study.
Another limitation of early studies is the reduced information available to the patient, typically limited to one or two channels so as not to overwhelm him during movement execution. Therefore, an effective task-oriented biofeedback system requires synergistic feedback of multiple channels that characterize the task performance without overwhelming a patient’s perception and cognitive ability. Bolek et al. [40] developed multiple-site performance-contingent feedback to treat motor dysfunction in two patients with cerebral palsy. Specifically, they conveyed information from four muscles of the lower limb to train postural stability while sitting. Right and left gluteusmedius were targeted to remain above threshold, while the right and left gluteus maximus were required to be below threshold. When this constellation of muscle groups was on target, a reward was activated. Failure to maintain any muscle at the therapeutic threshold terminated the reward. The aim of this approach was to internalize the correct muscle pattern recruitment rather than individual muscle activity. Improvement, expressed in percent of time the threshold was met, was reported for both the participants.
One more shortcoming of earlier biofeedback approaches was that the information presented often took the form of lines or bars on a computer screen or simple beeps. These were neither intuitive nor attention grabbing. Motivation and attention are two key factors for biofeedback training. The success of therapies aimed at inducing neuroplasticity is strictly related to the amount of time spent on active training. As a result, the training task should be attractive and motivating to keep the subject attentive for several repetitions of the task. This feature is particularly important when working with children who get tired and distracted easily, [38]. Multimedia-based technology uses computerized graphics and animation, together with sound and haptic stimulation, to immerse the subject in a constructed virtual reality (VR), [41]; thus it can be exploited to design biofeedback cues with the required features. Novel VR-based biofeedback systems have the potential to promote sustained attention, self-confidence, and motivation of participants during the repetitive task therapy. Therefore, there is widespread interest in using VR in rehabilitation of children with cerebral palsy, to address upper extremity, [42, 43] and lower extremity motor functions, [44]. Yoo and colleagues, [43] investigated the effectiveness of a combined EMG biofeedback and VR intervention system to improve muscle imbalance between triceps and biceps during reaching movements in three children with spastic cerebral palsy. Results reported an improvement in the muscle imbalance ratio between triceps and biceps compared to a traditional EMG-based biofeedback training. Another case report, [45] investigated the effects of VR therapy on cortical reorganization and associated motor function in an 8-year-old children with hemiparetic cerebral palsy. After VR therapy, the altered activations disappeared and the contralateral primary sensorimotor cortex was activated. This neuroplastic change was associated with enhanced functional motor skills, which were not possible before the intervention.
An important feature of these novel systems is that virtual applications that are Internet-deliverable pave the way for possible home-based rehabilitation, which has the potential to reduce the costs associated with long periods of hospitalization or traveling long distances, [46]. Interactive technologies also provide children with movement disorders with the chance to be involved without being judged because of their disability [47]. In this framework, Golomb and colleagues, [48] carried out a 3-month proof-of-concept pilot study on three adolescents with severe hemiplegic cerebral palsy, where they tested a VR video-game telerehabilitation system using a sensing glove fitted to the plegic hand, [49]. Based on several outcome measures, such as occupational therapy assessments, fingers’ range of motion (ROM), dual-energy x-ray absorptiometry (DXA), and peripheral quantitative computed tomography (pQCT) of the plegic forearm bone health, functional magnetic resonance imaging (fMRI) of hand grip task, the study reported improved hand function and forearm bone health for patients who practiced regularly. To address the need for technologies that facilitate children’s acquisition of play experiences, another group tested the effectiveness of an affordable home-based musical play system (the movement-to-music system (MTM)) on children with severe physical disabilities, who are typically limited to play and create music, [50]. The results, based on parental interviews, showed that the MTM technology had the potential to improve children’s body functions and enhance their participation in family activities. Another study developed a low-cost VR therapy system based on commercially-available game consoles (Sony PlayStation 2 equipped with an EyeToy video camera) to elicit practice of targeted neuromotor movements in five children with hemiplegia. The evaluation, based on the Quality of Upper Extremity Skills Test and on caregivers and parents questionnaires, showed that the system successfully elicited targeted neuromotor movements of the hemiplegic limb, [51].
The use of biofeedback techniques looks well suited for rehabilitation of children with cerebral palsy as a natural part of their daily activities. Findings indeed report a positive effect in motor rehabilitation, with improvements in motor control, spatial orientation skills, mobility, and an increase in motivation to practice even for children with severe grades of disabilities. However, even if at present studies report a general positive effect, there is scarcity of evidence of a strong beneficial effect, especially when it comes to VR studies. Indeed, studies that address the use of VR for rehabilitation of children with cerebral palsy are few, and the level of evidence is primarily limited to experimental and pilot studies with small samples. The large variation in outcome measures makes it hard to compare and integrate the results. In some studies, the assessment is based on qualitative interview, while there may be the need for more sensitive outcome measures that have the capacity to capture small motor changes. To conclude, the results show that the use of biofeedback and VR-based biofeedback in rehabilitation of children with cerebral palsy is a highly promising area in which further research is encouraged. In particular, further efforts to develop sensitive outcome measures and a common vocabulary within this research field is needed.
4 Noninvasive Brain Stimulation for Cerebral Palsy Rehabilitation
Noninvasive brain stimulation is growing as a very active research line because of its possibilities to enhance cognition, motor performance, rehabilitation after brain damage, and treatment of different psychopathologies. Basically, brain activity can be modulated by manipulation of neuron resting potential, rendering cells more prone to be activated if depolarized or reducing the probability of firing if hyperpolarized. Both effects, activation and inhibition, are reached by passing through the scalp a magnetic pulse or a weak electrical current, [52]. Transcranial Magnetic Stimulation (TMS) is based in a stimulator device that generates high intensity electrical pulses into a coil, superimposed above the scalp, to induce magnetic fields that easily pass the skull and modify actual electrical currents inducing activation or inhibition of cells. If the magnetic pulse is strong enough firing of neurons can also be induced. There are two main types of magnetic stimulation: single pulse (TMS) and repetitive transcranial magnetic stimulation (rTMS), [53]. Transcranial Direct Current Stimulation (tDCS) is based in an electrical device, battery powered, that delivers continuous current to a pair of electrodes situated above the scalp. The device contains specific software for programming the experimental setup and for maintaining a constant current intensity in function of variation on skull impedance. Electrodes are positioned to direct current flow between them, in such a way that tissue under anode is expected to become depolarized while brain tissue under cathode is said to be hyperpolarized. Electrical intensity is weak enough, between 0 and 2 mA, to ensure that no neuronal firing is triggered, [53].
Both techniques are being actively investigated for, directly or indirectly, enhancing neurorehabilitation. Thus, different reports show better performance on learning tasks (motor, sensory, or cognitive) of healthy participants as well of patients with brain damage (stroke, TBI, Alzheimer, Parkinson, epilepsy, amyotrophic lateral sclerosis, cerebral palsy, etc.), [52, 54]. Specifically the problem of spasticity has been targeted in various reports, mainly focused on stroke patients but also in cerebral palsy children. Two approaches can be indentified in the literature: direct modulation of spasticity by direct modulation of brain activity or modulation of typical motor training programs. Primary motor cortex, M1, sends out projections directly to the spinal cord, where it modulates spinal interneurons and reflex. Damage to M1 can result in spasticity because the absence, or reduction, of high-order motor commands imply the reduction of spinal inhibitory processes and a consequent overactivation of muscles. Thus, noninvasive M1 stimulation should increase spinal inhibition and spasticity. Working with this hypothesis has shown temporal reductions in upper or lower limb spasticity in stroke patients by stimulation itself but also by combination with physiotherapy or other functional motor tasks [55–57]. In 2007 Valle et al. [58], stimulated for 5 days the primary motor cortex of 17 CP children from 5 to 18 years old. 5 Hz stimulation, but not sham or 1 Hz, produced modest benefit in some, but not all, measures taken, namely upper limb joints range of motion. The more important information about these reports rely in safety data as no side effects were noted and no convulsions were generated in well-medicated patients diagnosed of epilepsy.
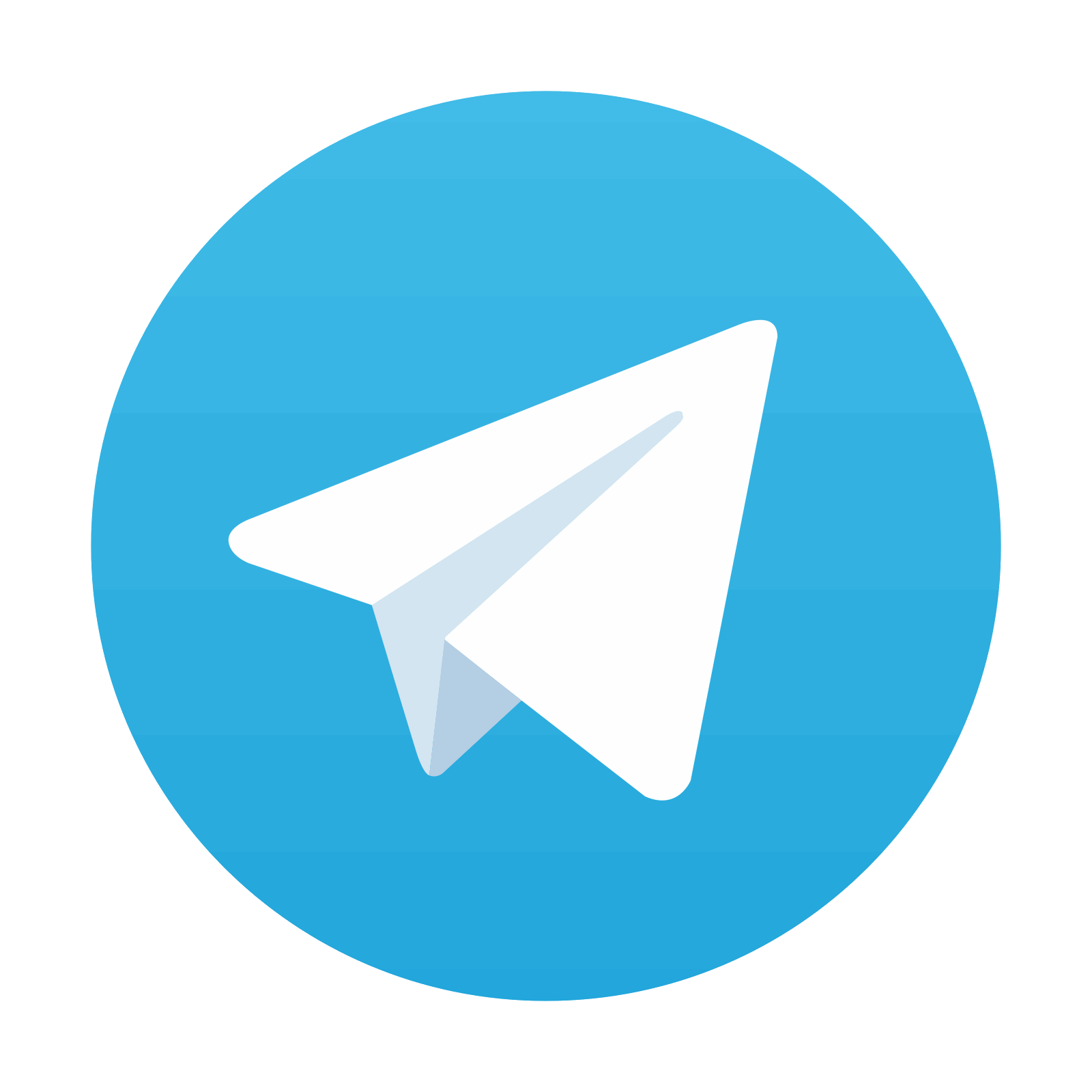
Stay updated, free articles. Join our Telegram channel

Full access? Get Clinical Tree
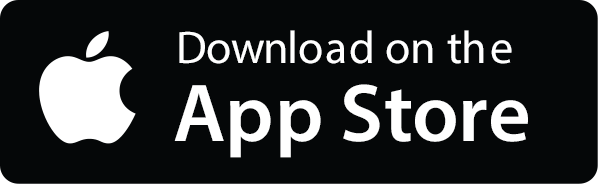
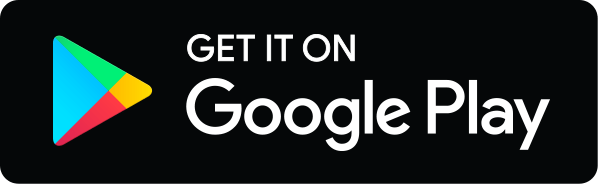