The Anatomy of Human Emotion
R. J. Dolan
Introduction
Emotions, uniquely among mental states, are characterized by psychological and somatic referents. The former embody the subjectivity of all psychological states. The latter are evident in objectively measurable stereotyped behavioural patterns of facial expression, comportment, and states of autonomic arousal. These include unique patterns of response associated with discrete emotional states, as for example seen in the primary emotions of fear, anger, or disgust often thought of as emotion proper. Emotional states are also unique among psychological states in exerting global effects on virtually all aspects of cognition including attention, perception, and memory. Emotion also exerts biasing influences on high level cognition including the decision-making processes that guide extended behaviour. An informed neurobiological account of emotion needs to incorporate how these wide ranging effects are mediated.
Although much of what we can infer about emotional processing in the human brain is derived from clinic-pathological correlations, the advent of high resolution, non-invasive functional neuroimaging techniques such as functional magnetic resonance imaging (fMRI) and positron emission tomography (PET) has
greatly expanded this knowledge base. This is particularly the case for emotion, as opposed to other areas of cognition, where normative studies have provided a much richer account of the underlying neurobiology than that available on the basis of observations from pathology as in classical neuropsychology.
greatly expanded this knowledge base. This is particularly the case for emotion, as opposed to other areas of cognition, where normative studies have provided a much richer account of the underlying neurobiology than that available on the basis of observations from pathology as in classical neuropsychology.
Emotion has historically been considered to reflect the product of activity within the limbic system of the brain. The general utility of the concept of a limbic-based emotional system is limited by a lack of a consensus as to its precise anatomical extent and boundaries, coupled with knowledge that emotion-related brain activity is, to a considerable degree, configured by behavioural context. What this means is that brain regions engaged by, for example, an emotion of fear associated with seeing a snake can have both distinct and common features with an emotion of fear associated with a fearful recollection. Consequently, within this framework emotional states are not unique to any single brain region but are expressed in widespread patterns of brain activity, including activity within early sensory cortices, shaped by the emotion eliciting context. This perspective emphasizes a global propagation of emotional signals as opposed to a perspective of circumscribed limbic-mediated emotion-related activity.
The amygdala and emotion
The above considerations aside, the structure most closely affiliated with emotional processing is the amygdala. This structure is an anatomically and functionally heterogeneous, bilateral, collection of nuclei located in anterior medial temporal cortex. The importance of the amygdala in emotional control was first highlighted by reports that rhesus monkeys with bilateral temporal lobe ablations no longer show appropriate fear or anger responses.(1) The role of the amygdala in emotion has been subsequently extended by findings that humans with lesions to this structure have impaired emotional recognition, particularly for fear, and no longer acquire Pavlovian conditioned responses(2) (see below). Finally, functional neuroimaging findings show activation of amygdala in responses to face stimuli that depict a range of emotions, particularly fear but also other primary emotions.(3, 4)
The importance of the amygdala in emotion derives in part from its extensive anatomical connections with all sensory processing cortices, as well as hippocampus, basal ganglia, cingulate cortex and the homeostatic regulatory regions of hypothalamus and brain stem.(5) This widespread anatomical connectivity means that this structure can access information processing in multiple brain regions and, in turn, can exert diffuse modulatory influences, including influences on effector autonomic and motor output systems. In this way activation of the amygdala by a sensory based emotional stimulus influences widespread brain regions including those that mediate homeostatic regulatory responses as expressed in altered autonomic state, such as change in heart rate, blood pressure and respiration.
Learning predictive emotional responses
A central role for emotion is to index value, specifically whether present or future sensory events or states of the environment that are likely to be associated with reward or punishment. From this perspective, all emotions are to a greater or lesser degree valanced. For example, an emotion of joy signals a likelihood of reward while an emotion of fear signals a likelihood of punishment. The fact that signals that predict such emotional occurrences are to some degree arbitrary means that the brain must have some means of associating sensory cues with potential emotional outcomes, an ability that seems crucial for adaptive behaviour.
Associative learning provides a phylogenetically highly conserved means to predict future events of value, such as the likelihood of food or danger, on the basis of predictive sensory cues. The amygdala plays a crucial role in mediating this form of emotional learning as evidenced by deficits seen with animal lesion data and learning-related effects seen in human functional neuroimaging experiments.(6, 7) In its simplest form, Pavlovian conditioning is expressed when a previously neutral sensory stimulus (the conditioned stimulus, or CS+) acquires emotional predictive significance through pairing with a biologically salient reinforcer (the unconditioned stimulus, or UCS). With conditioning, the predictive stimulus (CS+) comes to elicit behaviour previously associated with the UCS, but in the absence of UCS presentation. There is a wealth of animal and human data which now shows that the amygdala has a key role in this form of associative learning, for both appetitive and aversive outcomes.
How the brain updates predictions of emotional outcomes
While contingencies acquired on the basis of associative learning provide a basis for generation of predictions of future event of value in response to sensory cues, this form of learning lacks flexibility in optimizing future behaviour. For example, the value of future states associated with predictive cues may change in the absence of subsequent pairing with these cues. Thus, a cue that is associated with a particular food that is valued when a person is hungry has diminished relevance when the person is sated with that same food. Consequently, it is important for optimal adaptive behaviour to be able to maintain an updated representation of the current value of such sensory-predictive cues that does not slavishly depend on new learning in relation to that cue.
Reinforcer devaluation is a standard experimental methodology for examining how value representations accessed by predictive cues are updated. As indicated, in the case of food, its value can be decreased through what is termed sensory-specific satiety. In this type of manipulation, the reward value of a food eaten to satiety is reduced (devalued) relative to foods that are not eaten to satiety. In humans, functional neuroimaging measured brain responses elicited by predictive stimuli (such as a CS+), that have been subject to devaluation, are associated with significant response decrements in the OFC paralleling the behavioural effects of satiation.(8) This response pattern within OFC indicates that this region is involved in representing reward value of predictive stimuli in a flexible manner, observations that also accord with extensive evidence from animal lesion data.(9, 10)
The observation that neural responses evoked by a food predictive conditioned stimulus (a CS+) in OFC are directly modulated by hunger states can inform an understanding of the behavioural impact of pathologies that impact on orbital-frontal cortex, especially the feeding abnormalities observed in both the Kluver-Bucy syndrome and fronto-temporal dementias. Patients with these conditions frequently show increased appetite, indiscriminate eating, food cramming, and change in food preference, hyperorality, and even attempts to eat non-food items. A dysfunctional network
involving OFC and amygdala would mean that food cues, and other predictive cues, are unable to recruit motivationally appropriate representations of food-based reward value.
involving OFC and amygdala would mean that food cues, and other predictive cues, are unable to recruit motivationally appropriate representations of food-based reward value.
A computational account of emotional learning
Learning to predict reward or danger is a basic and highly conserved form of learning, as embodied in Pavlovian or associative learning. However, to be maximally adaptive, it is important that this form of learning is used not only to predict but also to shape optimal actions. The computational principles that underpin what is now referred to as value learning, involving prediction and optimization of action with respect to likely future outcomes, is more than an abstract issue and speaks to the critical issue of optimal control in decision-making.
One classical solution as to how associative learning is implemented is by means of a signal, referred to as a prediction error, which registers a difference between a predicted and actual outcome. This type of solution to predictive learning has been formalized within what is known as the Rescorla-Wagner learning rule. Temporal difference learning (TD) provides a more sophisticated computational extension of this learning rule that accounts in a precise manner for how an organism learns to make predictions, as well as select optimal actions, in response to states of the environment so as to maximize long-term reward or avoid long-term punishment.(11) As in the case of the Rescorla-Wagner model, when a positive (or negative outcome) is not predicted there is a large prediction error which reduces to zero when this same outcome is fully predicted. The function of the prediction error is to act as a teaching signal that can both update future predictions as well as shape optimal policies or action choices. In temporal difference learning (TD), credit is assigned by means of the difference between temporally successive predictions, rather than between a predictive stimulus and an outcome, such that learning occurs whenever there is a change in prediction over time.
The importance of the above theoretical considerations rests upon empirical observations that TD error-like responses have now been demonstrated in the response pattern of dopamine neurones recorded in monkeys during associative learning.(12) Consequently, in a classical conditioning context where a stimulus is followed by an unexpected reward it can be shown that dopamine neurones respond with a burst of action potentials after actual reward receipt. Over the course of learning, with repeated presentations of a predictive stimulus and reward, dopamine neurones no longer respond to receipt of the reward. In this latter case, the reward is accurately predicted because of the occurrence of the preceding predictive stimulus. What is now observed is a prediction error at the time of the earliest predictor of this reward, for example at time of presentation of a predictive CS stimulus. Prediction error type brain responses have also been shown to occur in the human striatum and orbital-prefrontal cortex during both Pavlovian and Instrumental learning in humans, as measured by fMRI.(13, 14) Indeed, a crucial link between a dopamine prediction error signal, human striate activity and reward-related choice behaviour in humans has also been shown using fMRI techniques. In this latter case, a reward outcome prediction error signal was enhanced by boosting the impact of dopamine using L-dopa (a precursor of dopamine), while a dopaminergic blocker Haloperidol led to an attenuation of a prediction error signal. Crucially, the former manipulation was associated with enhanced reward learning while the latter was associated with impaired reward learning in a manner that indicates that a reward outcome prediction error is involved in shaping optimal behaviour.(15)
How emotion influences memory
The cognitive domain where the modulatory influences of emotion have been best characterized is with respect to episodic memory, the type of memory that underpins autobiographical experience. Emotion enhances episodic memory function as seen in an enhancement for material that encompasses personal autobiographical, picture, and word based-items, an effect best seen in free recall tasks.(16) The critical role played by the amygdala in this modulation is illustrated by functional neuroimaging experiments where amygdala activity during encoding predicts a benefit in later recall of emotional material relative to neutral material.(17) Thus, enhanced amygdala activity at encoding for both positive and negative stimuli is predictive of later episodic memory function, during free recall tasks.
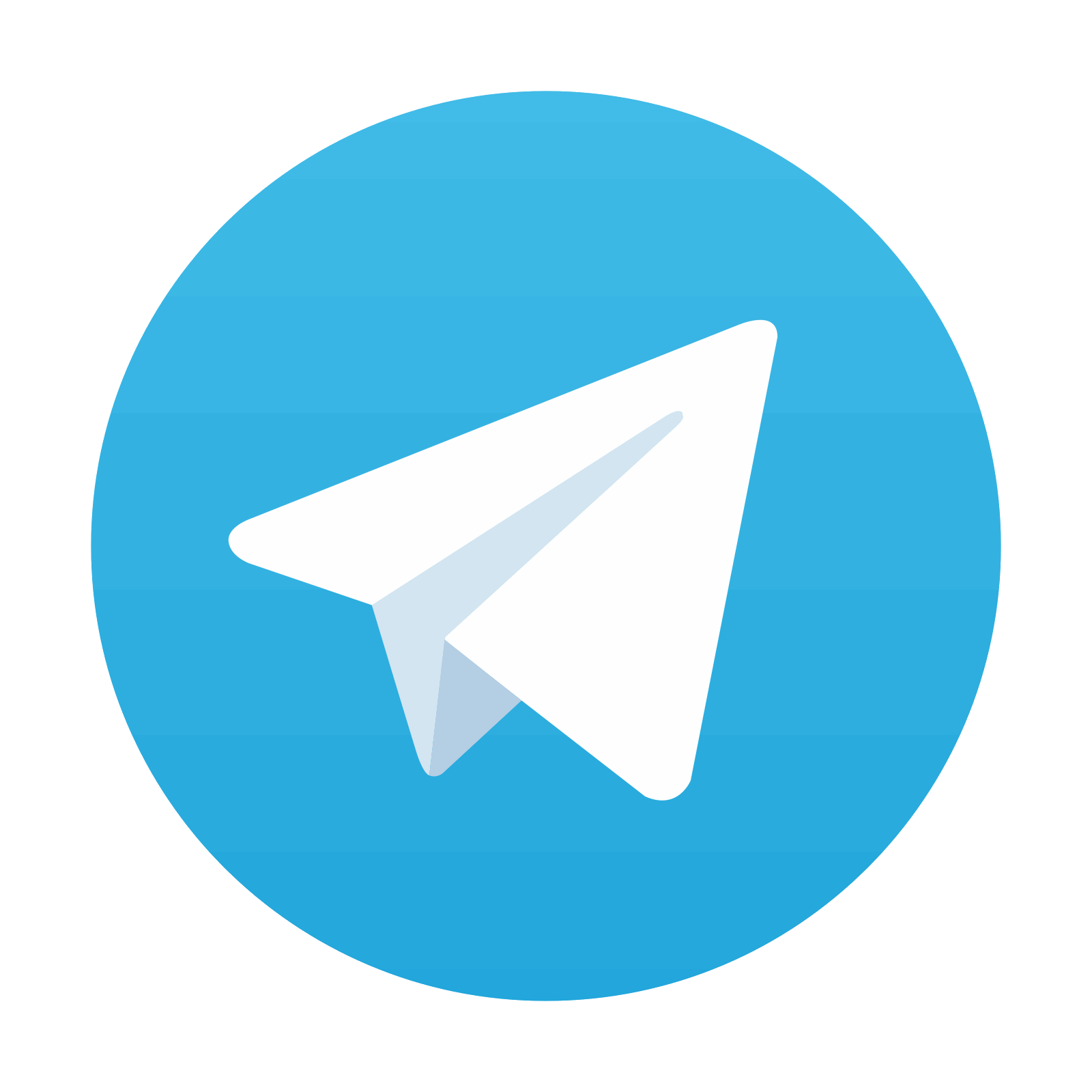
Stay updated, free articles. Join our Telegram channel

Full access? Get Clinical Tree
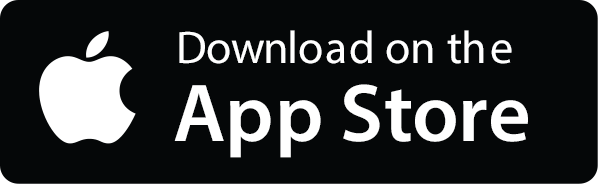
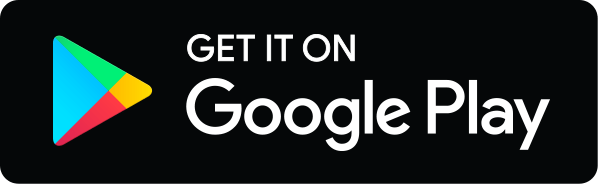