CLINICAL CASE | Acoustic Neuroma
A 40-year-old woman notes that she has been having difficulty understanding what people are saying when they stand on her left side. She also finds she hears better with the phone over her right, not left, ear. On examination, when a vibrating tuning fork is held at a distance from her left or right ear, she hears better with the right ear. When the tuning fork is placed on the mastoid process, thus eliminating air conduction, the same pattern of hearing ability persists, better on the right than left side. For either side, when placed on either mastoid process, the tuning fork sounds softer than when held near the ear. She is also observed to have a mild gait instability and mild flattening of the left nasolabial fold.
Figure 8–1A1 is an MRI, with gadolinium, showing a lesion in the left internal auditory canal, which is indicative of an acoustic neuroma. Figure 8–1 A2 shows an MRI from approximately the same level from a healthy person.
Based on your reading of this chapter, answer the following questions.
1. Explain why this patient has the following three signs: unilateral hearing loss, gait instability, and mild flattening of the left nasolabial fold.
2. What is the significance of preservation of the left hearing impairment whether the tuning fork is held at a distance from her ear or when it contacts the mastoid process?
Key neurological signs and corresponding damaged brain structures Unilateral hearing lossAn acoustic neuroma—typically a Schwann cell tumor, or schwannoma—preferentially impairs the function of the auditory division of the eighth cranial nerve. As the tumor grows, it expands the internal auditory canal, through which the nerve passes en route to the periphery (Figure 8–1B1-3). The eighth nerve peripheral auditory structures, and cochlear nuclei are the only sites where lesions produce a unilateral impairment. Central auditory system lesions do not produce deafness in one ear because of the numerous opportunities for auditory information to decussate.
Flattening of the nasolabial foldThe facial nerve joins with the eighth nerve to exit through the internal auditory canal (Figure 8–1B1). As a consequence, facial nerve function can also be compromised with acoustic neuromas. The facial nerve, as we will see in Chapter 11, innervates the muscles of facial expression, unilaterally. A clear sign of weakness of these facial muscles is the flattening of the skin fold that extends from the nose to the lateral edge of the mouth; sometimes this is termed a smile line. In addition to the muscles of the lower face, eighth nerve damage also can weaken other facial muscles. Eighth nerve functions are discussed in Chapter 11.
Gait instabilityThis can be produced either by compromised function of the vestibular division of the eighth nerve or by compression of the pons and cerebellum by the expanding tumor. This patient does not report vertigo, a sign of vestibular dysfunction. Gait instability is a common sign of cerebellar dysfunction (Chapter 13). Ataxia is a form of incoordination associated with cerebellar disease or damage. The gait instability can be due to lower extremity ataxia. Note on the MRI that the tumor is displacing the pons and cerebellum, making pontocerebellar dysfunction a likely explanation for the instability.
Air versus bone conductionAs discussed in this chapter (also see Figure 8–3A), sound is conducted to the inner ear via the tympanic membrane and middle ear ossicles. This is the optimal conduction route. Alternatively, sound vibrations can activate the inner ear directly (ie, vibrate the basilar membrane) by conduction through the bone. Under normal conditions, air conduction is much better than bone conduction and, in consequence, sounds are heard better through the air, not bone. The patient shows this normal pattern. This is expected because her problem is not impairment of the middle ear ossicles, but rather conduction of neural signals to the brain.
FIGURE 8–1
Acoustic neuroma. A. MRI. A1. MRI from a patient, with the contrast material gadolinium, which produces better delineation of the tumor from surrounding tissues showing the tumor. A2. MRI from a healthy person. B. Inner surface of the skull in the region of the cerebellopontine angle with the brain stem and cerebellum removed to show the cranial nerves and associated foramina through which they exit the cranial cavity. B1. Normal. B2. Acoustic neuroma at an early stage when it is small and not displacing the brain stem. B3. Acoustic neuroma at a later stage when it displaces the pons and cerebellum and can also affect the functions of nearby cranial nerves, as shown in the figure. These include: (1) facial somatic sensation and corneal reflex because of fifth nerve involvement; (2) taste, because of sensory fibers in the seventh nerve; (3) eye muscle control because of the sixth nerve; (4) facial muscle control because of the seventh nerve; and (5) oral-pharyngeal sensory functions of glossopharyngeal nerve. Further, greater expansion into the pons can lead to corticospinal tract impairments, because this motor path is located in the ventral pons, and more severe cerebellar motor impairments. (A1, Courtesy of Dr. Frank Gaillard, Radiopaedia.com. A2, Courtesy of Dr. Joy Hirsch, Columbia University.)

The auditory system mediates hearing, a sensory experience that is as broad as the sound spectrum itself. From signals of impending danger, like a car horn, to the pleasing sounds that fill a concert hall, much of our daily behavior is determined by the sounds around us. The auditory system is also our principal communication portal, allowing us to understand speech. This system, like the somatic sensory and visual systems, has a topographic organization determined by the peripheral receptive sheet. And similar to the other systems, the auditory system consists of multiple parallel pathways that engage multiple cortical regions, either directly or via complex corticocortical networks. Each auditory pathway is hierarchically organized and has the connections and properties to mediate different aspects of hearing.
The complexity of the auditory pathways derives from the particular properties of natural sounds, with their diverse frequency characteristics, multiple sources of origin, and large dynamic ranges. However, an added measure of complexity is imposed on the human auditory system by the demands of producing and understanding speech. Although the physical characteristics of a spoken word may be simpler than many sounds that are not part of our lexicon, the linguistic quality of the stimulus engages unique cortical areas. This chapter first considers the general functional organization of the auditory system. Then it examines key levels through the brain stem and thalamus, where auditory information is processed. Finally, the complex connections of the auditory and speech centers of the cerebral cortex are examined.
The process of hearing begins on the body surface, as sounds are conducted by the auricle and external auditory meatus to the tympanic membrane. Mechanical displacement of the tympanic membrane, produced by changes in sound pressure waves, is transmitted to the inner ear by tiny bones termed the middle ear ossicles (see Figure 8–3). The inner ear transductive machinery is located within the temporal bone in a coiled structure called the cochlea. This is the location of the auditory receptors, termed hair cells because they each have a bundle of hair-like stereocilia on their apical surface. Each auditory receptor is sensitive to a limited frequency range of sounds. Hair cells in the human cochlea are not mitotically replaced, and their numbers decline throughout life. This reduction can be exacerbated by conditions such as ear infections, exposure to loud sounds or drugs with ototoxic properties.
A topographic relationship exists between the location of a hair cell in the cochlea and the sound frequency to which the receptor is most sensitive. As discussed later, from the base of the cochlea to the apex, the frequency to which a hair cell is maximally sensitive changes systematically from high frequencies to low frequencies. This differential frequency sensitivity of hair cells along the length of the cochlea is the basis of the tonotopic organization of the auditory receptive sheet. Many of the components of the auditory system are tonotopically organized. The topographic relationship between the receptor sheet and the central nervous system is similar to that of the somatic sensory and visual systems, where the subcortical nuclei and cortical areas have a somatotopic or retinotopic organization. In each of these cases, the topographic organization of the central representations is determined by the spatial organization of the peripheral receptive sheet. An important difference exists, however. The receptor sheets of the somatic sensory and visual systems are spatial maps representing stimulus location (eg, hand versus foot, central versus peripheral vision). The cochlea represents the frequency of sounds. Localizing where a sound originates is determined by central nervous system auditory neurons, computed on the basis of the timing, loudness, and spectral characteristics of sounds (see below).
Hair cells are innervated by the distal processes of bipolar primary sensory neurons located in the spiral ganglion. The central processes of the bipolar neurons form the cochlear division of the vestibulocochlear nerve (cranial nerve VIII). These axons project to the ipsilateral cochlear nuclei (Figure 8–2), which are located in the rostral medulla. The cochlear nuclei consist of the ventral cochlear nucleus, which has anterior and posterior subdivisions, and the dorsal cochlear nucleus. Neurons in these three components have distinct connections with the rest of the auditory system and give rise to parallel auditory pathways that serve different aspects of hearing. A key function of the ventral cochlear nucleus is horizontal localization of sound. In addition, some neurons in the posteroventral division contribute to a system of connections that regulate hair cell sensitivity. The ventral cochlear nucleus projects to the superior olivary complex, a cluster of nuclei in the caudal pons. Most neurons in the superior olivary complex project via an ascending pathway called the lateral lemniscus to the inferior colliculus, located in the midbrain. The projection from the ventral cochlear nucleus to the inferior colliculus is bilateral, reflecting the importance of binaural mechanisms for horizontal (side-to-side) localization of sounds. The dorsal cochlear nucleus is thought to play a role in identifying sound source elevation as well as identifying complex spectral characteristics of sounds. It projects directly to the contralateral inferior colliculus, also via the lateral lemniscus. The inferior colliculus is the site of convergence of all lower brain stem auditory nuclei. It is tonotopically organized and contains a spatial map of the location of sounds.
FIGURE 8–2
Organization of the auditory system. A. Dorsal view of brain stem, illustrating the organization of major components of the auditory system. B. Organization of the auditory system revealed in cross section at different levels through the brain stem and in coronal section through the diencephalon and cerebral hemispheres. The inset shows schematically the locations of the auditory and speech-related areas of the cerebral cortex. Wernicke’s area, for understanding speech, is located in the superior temporal gyrus. Broca’s area, for articulating speech, is located in the inferior frontal gyrus. Heschl’s gyri are located within the lateral sulcus and cannot be seen on the surface.

In sequence, the next segment of the ascending auditory pathway is the medial geniculate nucleus, the thalamic auditory relay nucleus. The medial geniculate nucleus projects to the primary auditory cortex, located within the lateral sulcus (also called the Sylvian fissure) on the superior surface of the temporal lobe. The primary auditory cortex contains multiple tonotopically organized territories, all located on Heschl’s gyri (Figure 8–2B, inset; see Figure 8–8). The primary cortex forms a central core surrounded by multiple secondary auditory areas that form a belt around the primary core. Neurons in the primary core are activated by simple tones, whereas those in the surrounding belt of secondary areas are better activated by complex sounds. Several higher-order auditory areas adjoin the secondary areas on the superior and lateral surfaces of the temporal lobe in the superior temporal gyrus and sulcus (Figure 8–2B). This is where several areas are located that are important for understanding speech (see below).
There is logic to the organization of the projections from the primary cortex, much like that of the visual system’s what and where (or how) pathways (see Figure 7–15). There is a ventral stream that originates anteriorly and projects to the ventral portion of the frontal lobe, including Broca’s area. This path may be analogous to the “what” path and is thought to be important in identifying the source of speech, such as a bark from a dog or meow from a cat. It is also a path involved in analyzing the linguistic meaning of sounds. There is a dorsal stream that originates caudally and projects to the parietal lobe and, from there, preferentially to the dorsolateral prefrontal and premotor cortical areas of the frontal lobe. This path is thought to be more important for spatial localization of the source of sounds and for using sounds for actions.
The auditory pathways contain decussations and commissures—where axons cross the midline—at multiple levels, so that sounds from one ear are processed by both sides of the brain. The bilateral representation of sounds provides a mechanism for sound localization (see below) and enhancing the detection of sounds through summation of converging inputs. Apart from sound localization, what is the clinical significance of this bilateral organization of central auditory connections? Unilateral brain damage does not cause deafness in one ear unless the injury destroys the cochlear nuclei or the entering fascicles of the cochlear nerve. Unilateral deafness is thus a sign of injury to the peripheral auditory organ or the cochlear nerve. As discussed in later sections of the chapter, unilateral damage to central auditory centers produces impairment in localizing and interpreting sounds or linguistic disorders, not deafness.
The membranous labyrinth is a complex sac within the bony labyrinth, cavities in the petrous portion of the temporal bone (Figure 8–3). The membranous labyrinth consists of the auditory sensory organ, the cochlea, and five vestibular sensory organs, the three semicircular canals, and the saccule and utricle (Figure 8–3A). (Another name for the semicircular canals, utricle, and saccule is the vestibular labyrinth.) The morphological complexity of the auditory and vestibular sensory organs rivals that of the eyeball. Vestibular sensory organs mediate our sense of acceleration, such as during takeoff in a jet, and are important in balance and eye movement control. The vestibular system is considered in Chapter 12. Much of the membranous labyrinth is filled with endolymph, an extracellular fluid resembling intracellular fluid in its ionic constituents. Endolymph has a high potassium concentration and low sodium concentration. Perilymph, a fluid resembling extracellular fluid and cerebrospinal fluid, fills the space between the membranous labyrinth and the temporal bone.
FIGURE 8–3
Structure of the human ear. A. The external ear (auricle) focuses sounds into the external auditory meatus. Alternating increasing and decreasing air pressure vibrates the tympanum (ear drum). These vibrations are conducted across the middle ear by the three ear ossicles: malleus, incus, and stapes. Vibration of the stapes stimulates the cochlea. B. Cut-away view of the cochlea, showing the three coiled channels: scala vestibuli, scala media, and scala tympani. C. Expanded view of a section through the cochlear duct, illustrating the organ of Corti. (A, Adapted from Noback CR. The Human Nervous System: Basic Elements of Structure and Function. New York, NY: McGraw-Hill; 1967., C, Adapted from Dallas P. Peripheral mechanisms of hearing. In: Darian-Smith I, ed. Handbook of Physiology. Vol. 3. Sensory Processes. Bethesda, MA: American Physiological Society; 1984:595-637.)

The cochlea is a coiled structure about 30 mm long (Figure 8–3A). The hair cells are located in the organ of Corti, a specialized portion of the cochlear duct that rests on the basilar membrane (Figure 8–3C). Hair cells of the organ of Corti are covered by the tectorial membrane (Figure 8–3C). The basilar membrane, hair cells, and tectorial membrane collectively form the basic auditory transductive apparatus. Two kinds of hair cells are found in the organ of Corti, and their names reflect their position with respect to the axis of the coiled cochlea: inner and outer hair cells. Inner hair cells are arranged in a single row, whereas outer hair cells are arranged in three or four rows. Although there are fewer inner than outer hair cells (approximately 3500 vs 12,000), the inner hair cells are responsible for frequency and other fine discriminations in hearing. This is because most of the axons in the cochlear division of cranial nerve VIII innervate the inner hair cells. Each inner hair cell is innervated by as many as 10 auditory nerve fibers, and each auditory fiber contacts only a single, or at most a few, inner hair cells. This is a high-resolution system, like that of the innervation of the fingertips and the fovea. By contrast, only a small fraction of auditory nerve fibers innervates the outer hair cell population. Each fiber branches to contact multiple outer hair cells. Research has shown that outer hair cells are important as efferent structures, modulating the sensitivity of the organ of Corti (see the section on the olivocochlear system, below).
The organ of Corti transduces sounds into neural signals. This organ is mechanically coupled to the external environment by the tympanic membrane and the middle ear ossicles (malleus, incus, and stapes), the smallest bones of the body (Figure 8–3A). Pressure changes in the external auditory meatus, resulting from sound waves, cause the tympanic membrane to vibrate. The middle ear ossicles—the malleus, incus, and stapes—conduct the external pressure changes from the tympanic membrane to the scala vestibuli of the inner ear (Figure 8–3B). These pressure changes are conducted from the scala vestibuli through the fluid to the other compartments of the cochlea, the scala media to the scala tympani (Figure 8–3B). Pressure changes resulting from sounds set up a traveling wave along the compliant basilar membrane (Figure 8–3C), on which the hair cells and their support structures rest. Because the hair cells have hair bundles that are embedded in the less compliant tectorial membrane, the traveling wave results in shearing forces between the two membranes. These shearing forces cause the hair bundles to bend, resulting in a membrane conductance change in the hair cells.
Hearing thus depends on movement of the basilar membrane produced by sounds. Outer hair cells can enhance this movement, thereby amplifying the signal generated by the organ of Corti in response to sound. They do so by changing their length in response to sounds (see section on the olivocochlear system, below). This results in a small additional displacement of the basilar membrane that increases the mechanical oscillation produced by changes in sound pressure on the tympanic membrane.
The traveling wave on the basilar membrane, established by changes in sound pressure impinging on the ear resulting from sounds, is extraordinarily complex. High-frequency sounds generate a wave on the basilar membrane with a peak amplitude close to the base of the cochlea; consequently, these sounds preferentially activate the basal hair cells. As the frequency of the sound source decreases, the location of the peak amplitude of the wave on the basilar membrane shifts continuously toward the cochlear apex. This results in the preferential low-frequency activation of hair cells that are located closer to the cochlear apex. Although the mechanical properties of the basilar membrane are a key determinant of the auditory tuning of hair cells and the tonotopic organization of the organ of Corti, other factors play important roles. For example, the length of the hair bundle varies with position within the cochlea. The bundles act as miniature tuning forks: The shorter bundles are tuned to high frequencies (and are located on hair cells at the cochlear base), whereas the longer bundles are tuned to low frequencies (and are located on hair cells at the apex). The electrical membrane characteristics of hair cells also contribute to frequency tuning. As is discussed in the next section, the tonotopic organization underlies the topography of connections in the central auditory pathways.
The cochlear nuclei, located in the rostral medulla, comprise the ventral cochlear nucleus, which has anterior and posterior subdivisions, and the dorsal cochlear nucleus (Figure 8–4C). The dorsal and ventral cochlear nuclei are each tonotopically organized and have distinctive functions. The ventral cochlear nucleus is important for horizontal sound localization. In addition, some of the neurons in the posteroventral component engage a system for regulating hair cell sensitivity. The ventral cochlear nucleus projects bilaterally to the superior olivary complex. Whereas we know much about the physiological characteristics of neurons in the dorsal cochlear nucleus—many process the spectral characteristics of sounds—its perceptional functions are not as well understood. The dorsal cochlear nucleus is thought to be important for vertical sound localization, which depends on spectral information (see next section), and for analyzing complex sounds. It projects directly to the contralateral inferior colliculus, bypassing the superior olivary complex.
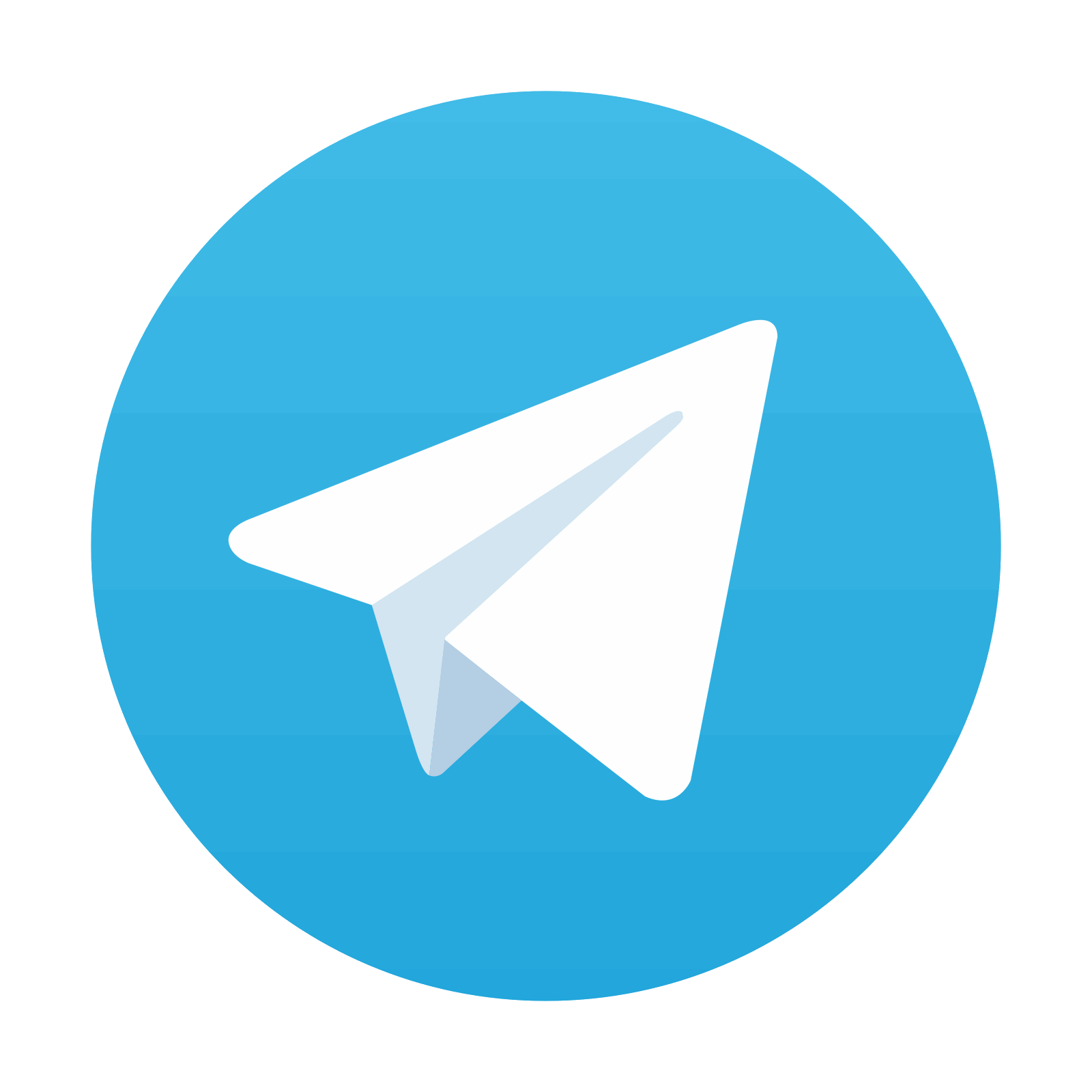
Stay updated, free articles. Join our Telegram channel

Full access? Get Clinical Tree
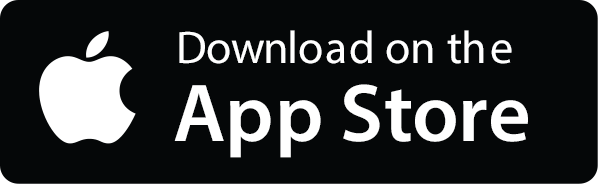
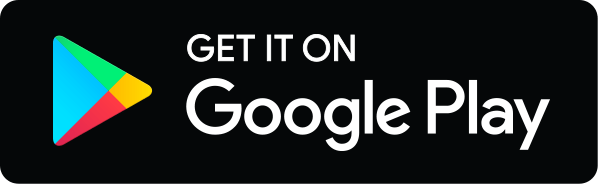