CLINICAL CASE | Hemiballism
A 65-year-old man with a history of hypertension suddenly developed involuntary, violent, ballistic movements of his right arm and leg. The movements primarily involved flexion and rotation of the proximal parts of the limbs. MRI showed a small hemorrhagic lesion in the subthalamic nucleus on the left side (Figure 14–1A).
Answer the following questions based on your reading of this chapter and relevant sections from other chapters.
1. Explain why the aberrant ballistic movements are on the contralateral side.
2. Occlusion of which cerebral artery and branch could produce a lesion such as the one shown in Figure 14–1?
Key neurological signs and corresponding damaged brain structures Subthalamic nucleus circuitryThe subthalamic nucleus is part of the indirect pathway. It receives GABA-ergic inputs from the external segment of the globus pallidus and projects to the internal segment of the globus pallidus. From there, information is directed to the motor thalamus, and then the motor cortex, which controls movements contralaterally, via the corticospinal tract. Additionally, the subthalamic nucleus receives dense glutamatergic inputs from the motor cortex, primarily on the ipsilateral side. Whereas the cortical-basal ganglia circuitry is ipsilateral, it exerts its movement control influence on the contralateral side because the corticospinal tract is predominantly crossed. The nucleus is somatotopically organized; the lesion shown in Figure 14–1A is sufficiently large to affect both its arm and leg areas of the small nucleus.
Because the subthalamic nucleus normally activates an inhibitory structure—the internal segment of the globus pallidus—when it is lesioned it is reasoned that this inhibition is less. Hemiballism is thus thought to be produced by disinhibition; it is a release phenomenon. It is not known why this is reflected in the violent proximal movements, so much a signature of subthalamic nucleus damage.
The largest portion of the subthalamic nucleus is devoted to limb and trunk motor functions. In addition, smaller regions of the nucleus are more important for eye movement control, emotional, and cognitive functions. These regions are parts of the ocular motor, limbic, and cognitive loops of the basal ganglia.
ReferencesBrust JCM. The Practice of Neural Science. New York, NY: McGraw-Hill; 2000.
Kitajima M, Korogi Y, Kakeda S, et al. Human subthalamic nucleus: evaluation with high-resolution MR imaging at 3.0 T. Neuroradiology. 2008;50(8):675-681.
Nishioka H, Taguchi T, Nanri K, Ikeda Y. Transient hemiballism caused by a small lesion of the subthalamic nucleus. J Clin Neurosci. 2008;15(12):1416-1418.
Hamani C, Saint-Cry JA, Fraser J, Kaplitt M, Lozano A. The subthalamic nucleus in the context of movement disorders. Brain. 2004;127:4-20.
FIGURE 14–1
Hemiballism. A. Schematic drawing in the plane of the MRI showing the location of the subthalamic nucleus and lesion. B. MRI from a person with a small hemorrhagic lesion of the left subthalamic nucleus. (Reproduced with permission from Nishioka H, Taguchi T, Nanri K, Ikeda Y. Transient hemiballism caused by a small lesion of the subthalamic nucleus. J Clin Neurosci. 2008;15:1416-1418.)

The basal ganglia are a collection of subcortical nuclei that have captured the fascination of clinicians and scientists for well over a century because of the remarkable range of behavioral dysfunction associated with basal ganglia disease. Movement control deficits are among the key signs, ranging from the paucity and slowing of movement in Parkinson disease and the writhing movements of Huntington disease to the bizarre tics of Tourette syndrome and distorted postures of dystonia. Unmistakingly, these clinical findings indicate that one important set of basal ganglia functions is regulating our motor actions. How do the basal ganglia fit into an overall view of the organization of the motor systems? Unlike the motor cortex and several brain stem nuclei, which have direct connections with the spinal cord and motor neurons, the basal ganglia influence movements by acting on the descending pathways; this is similar to the cerebellum.
In addition to producing movement control deficits, basal ganglia disease can also impair intellectual capacity and affect, pointing to important roles in cognition and emotion. Dementia is an early disabling consequence of Huntington disease and can be present in patients with advanced stages of Parkinson disease. The basal ganglia play important roles in aspects of drug addiction and psychiatric disease.
Although the basal ganglia continue to be among the least understood of all brain structures, their mysteries are now yielding to modern neurobiological techniques for elucidating neurochemistry and connections. For example, the basal ganglia contain virtually all of the major neuroactive agents that have been discovered in the central nervous system. Although the reason for this biochemical diversity remains elusive, such knowledge can be used to treat some forms of basal ganglia disease. Indeed, the discovery that the brains of patients with Parkinson disease are deficient in dopamine quickly led to the development of drug replacement therapy. Knowledge about connections of the basal ganglia with the rest of the brain has led to a major revision of the traditional views of basal ganglia organization and function. Discoveries about basal ganglia circuitry and pathways have even led to therapeutic neurosurgical and neurophysiological procedures.
This chapter first considers the constituents of the basal ganglia and their three-dimensional shapes, partly from a developmental context. Next, their functional organization is surveyed, emphasizing the distinctive roles of the basal ganglia in movement control, cognition, and emotions. (Chapter 16 revisits the basal ganglia in relation to emotions and psychiatric disease.) Finally, this chapter examines the regional anatomy of the basal ganglia using a series of myelin-stained sections and MRI slices through the cerebral hemispheres and brain stem.
The many components of the basal ganglia are best learned, in a general way, from the outset; then their functional and clinical anatomy can be mastered. As we will focus on later in the context of their connections, the components of the basal ganglia can be divided into three categories: input, output, and intrinsic nuclei (Table 14–1). The input nuclei receive afferent connections from brain regions other than the basal ganglia, in particular the cerebral cortex, and in turn project to the intrinsic and output nuclei. There are three input nuclei, merged into a single structure termed the striatum (Figure 14–2): (1) the caudate nucleus, (2) the putamen, and (3) the nucleus accumbens. The functions of the striatum do not correspond precisely to its component anatomical parts. Most of the caudate nucleus participates in eye movement control and cognition, whereas the putamen participates mostly in control of limb and trunk movements. Emotions are mediated by the nucleus accumbens, together with adjoining parts of the caudate nucleus and putamen; the emotional striatum is commonly termed the ventral striatum. Given that the striatum is really a composite of three nuclei, it is not surprising that it has a complex shape.
Input nuclei (striatum)1 |
|
Output nuclei |
|
Intrinsic nuclei |
|
The output nuclei project to regions of the diencephalon and brain stem that are not part of the basal ganglia. There are three nuclei on the output side of the basal ganglia (Table 14–1; Figure 14–2B1, B2): the internal segment of the globus pallidus, associated mostly with the putamen and limb and trunk control; the substantia nigra pars reticulata, primarily involved in cognition and eye movements along with the caudate nucleus, and a portion of the ventral pallidum, important for emotions with the ventral striatum. These nuclei are located deep within the base of the brain; they are shown schematically in Figure 14–2 in relation to a transparent view of the striatum.
The intrinsic nuclei are also located deep within the base of the brain; their connections are largely restricted to the basal ganglia (Table 14–1; Figure 14–2). The basal ganglia have five intrinsic nuclei: the external segment of the globus pallidus, a portion of the ventral pallidum (separate from the output part), the subthalamic nucleus, the substantia nigra pars compacta, and the ventral tegmental area (Figure 14–2). Their connections are closely related to the input and output nuclei.
The Complex Shapes and Fractionation of Basal Ganglia Components Are Understood by How the Basal Ganglia Develop
Learning the numerous components and subdivisions of the basal ganglia is a challenge. Taking a developmental perspective helps to understand two key features of the anatomy of the basal ganglia: the complex three-dimensional shape and fractionation of the components of the basal ganglia into subdivisions. The caudate nucleus develops a C-shape, largely as a consequence of cerebral cortex development. As the cortex expands caudally and inferiorly, forming the occipital and temporal lobes (Figure 14–3A), underlying structures—including the caudate nucleus and lateral ventricle—follow. This expansion and change in shape are produced by the birth and migration of cells along predetermined axes. This imparts a distinctive shape of the caudate nucleus in relation to the shapes of the other two striatal components. The C-shape of the caudate nucleus results in three components: head, body, and tail (Figure 14–3C).
FIGURE 14–3
Development of the basal ganglia. A. Lateral views of the developing brain and head at different prenatal ages (A1–A4) and term. Schematic diagrams of the cerebral hemisphere, lateral ventricle, and striatum accompany each age. The fibers in A5 (right) are of the internal capsule. B. Brain sections through 50-day embryo (B1) and 7-month embryo (B2). Schematic ascending and descending axons in the internal capsule are shown in B2. C. The striatum in relation to the ventricular system in the mature brain. The striatum consists of the caudate nucleus, putamen, and nucleus accumbens. Only the caudate nucleus has a C–shape, which is similar to that of the lateral ventricle. The nucleus accumbens is located ventromedially primarily on the medial striatal surface.

A second developmental process contributes to formation of some of the basal ganglia subdivisions. Development of axon projection to and from the cerebral cortex in the internal capsule divides many basal ganglia components into separate nuclei, thereby increasing the complexity of the nomenclature. Figure 14–3B, schematic coronal sections through the developing forebrain, shows a single developing striatum (Figure 14–3B1) and separation of the caudate nucleus (head and body) and the putamen by the internal capsule. Three developing axon projections within the internal capsule are key, those from (1) the thalamus to the cortex (ie, to layer 4); (2) the cortex (layer 6) back to the thalamus; and (3) the cortex (layer 5) to the striatum, brain stem, and spinal cord. For the striatum, these internal capsule axons incompletely divide the striatum into the three components, leaving behind cell bridges (Figure 14–3C). Throughout its entire course, the caudate nucleus is medial to the internal capsule, and the putamen, lateral. Figure 14–4A shows the course of the corticospinal tract in the internal capsule; its path can be followed between the head of the caudate and the putamen. The nucleus accumbens is largely that portion of the striatum rostral and inferior to the anterior limb of the internal capsule. The tail of the caudate nucleus is separated from the putamen by additional projection fibers.
The internal capsule also separates the internal segment of the globus pallidus and the substantia nigra pars reticulata, similar to the striatal cell bridges, shown in maturity in Figure 14–3. In humans, cells of the substantia nigra pars reticulata and internal segment of the globus pallidus are scattered between each other within the internal capsule (see Figure 14–15). In addition to being part of the same structure but separated by the internal capsule, the morphology, neurotransmitter content, and connections of neurons in the internal segment of the globus pallidus and the substantia nigra pars reticulata are similar.
Although not understood from a simple developmental perspective, the anterior commissure separates the external segment of the globus pallidus from the ventral pallidum. However, many neurons in the ventral pallidum have connections and a neurochemistry similar to the globus pallidus external segment—like that of an intrinsic nucleus—and others, especially in the more caudal ventral pallidum, have properties like the internal segment of the globus pallidus, an output nucleus.
Direct and Indirect Pathways Form Common Circuits Throughout All Functional Divisions of the Basal Ganglia
The general organization of the basal ganglia from input to output is shown in Figure 14–5. Like the cerebellum, a basic circuit describes the basal ganglia, irrespective of motor, cognitive, or emotional function. As described, the basal ganglia are divided into input, output, and intrinsic nuclei (Figure 14–5A). Beginning with all areas of the cerebral cortex, information passes through the input nuclei and then the output nuclei. Connections with the thalamus target several nuclei that, in turn, project to different areas of the frontal lobe. These differential projections confer the distinctive functions of the basal ganglia (discussed below). Connections with the brain stem motor control centers target the pedunculopontine nucleus, important in gait control, and the superior colliculus, for saccadic eye movements. As an example of the flow of information through the basal ganglia, follow the path in Figure 14–5B, from the frontal lobe, to the putamen, globus pallidus internal segment, thalamus, and back to the cerebral cortex (to primary motor cortex).
FIGURE 14–5
Direct and indirect paths of the basal ganglia. A. Block diagram. The input nuclei are the components of the striatum; they receive input from all cortical areas. The output nuclei are the globus pallidus internal segment, the substantia nigra pars reticulata, and part of the ventral pallidum. Blue shading is the direct path. Green shading shows the indirect path. Whereas the basal ganglia receive input from all cortical areas, the return path from the thalamus is directed only to the frontal lobe. Note, dopaminergic cell groups innervate both the cerebral cortex and the striatum. Connections to the brain stem are directed to the superior colliculus, for eye movement control, and the pedunculopontine nucleus, for gait control. B. Circuitry of the direct path. Follow the path from cortex: (1) back to cortex; and (2) to brain stem. Note how both paths eventually end in the spinal cord. Inset shows the indirect path that terminates in the internal globus pallidus. Motor pathways to the spinal cord are also shown.

There are functionally and clinically important connections with the intrinsic nuclei. The external segment of the globus pallidus and the subthalamic nucleus are part of a basal ganglia circuit that receives input from other basal ganglia nuclei and in turn projects back (Figure 14–5A). The substantia nigra pars compacta and the ventral tegmental area contain dopaminergic neurons that project to the striatum, as well as to portions of the cortex (Figure 14–5A). Dopamine has a neuromodulatory action on striatal neurons. There are many different dopamine receptor subtypes and, depending on the particular subtype present on the postsynaptic neuron, dopamine either depolarizes or hyperpolarizes striatal neurons.
Whereas the basal ganglia have a daunting complexity, there is a logic to the connections that helps explain their overall actions. A pair of complementary circuits—termed the direct and indirect pathways—have opposing actions on their downstream structures. The connections from the striatum to the output nuclei and then to the thalamus and brain stem, described above, comprise the direct path (Figure 14–5A, B), which promotes the actions of the basal ganglia. By contrast, connections from the striatum to three intrinsic nuclei—the external segment of the globus pallidus, part of the ventral pallidum, and the subthalamic nucleus—comprise the indirect path (Figure 14–5A, B inset), which inhibits the actions of the basal ganglia. In Figure 14–5B (inset) follow the path from the striatum, globus pallidus external segment, subthalamic nucleus, and then globus pallidus internal segment. For the components of the basal ganglia that control limb and trunk movements, eye movements, and facial muscles, the direct path enables these actions and the indirect path puts the brakes on. Box 14–1 shows schematically how neuronal activity changes in the direct and indirect paths as information from the cortex is processed from one structure to the next. Aberrant direct path functions in many movement disorders appear to drive excessive muscle tone, tics, and habitual behaviors, and aberrant indirect path functions produce debilitating akinesia, bradykinesia, and rigidity in Parkinson disease. It is thought that similar facilitatory and suppressive actions influence the nonmotor functions of the basal ganglia as well. We consider below how the complementary actions of the direct and indirect paths occur, in the context of the diversity of neurotransmitter actions in the basal ganglia.
Box 14–1 Knowledge of the Intrinsic Circuitry of the Basal Ganglia Helps to Explain Hypokinetic and Hyperkinetic Signs
Knowledge of dysfunction in the direct and indirect pathways in the skeletomotor loop (Figures 14–5, 14–6, 14–7) is helping to explain the mechanisms of disordered movement control in basal ganglia disease and to develop more effective therapies. As discussed earlier, the direct path promotes movements, and the indirect path inhibits movements. Projection neurons of the putamen in the direct path synapse on neurons in the internal segment of the globus pallidus, which project to the ventrolateral and ventral anterior nuclei of the thalamus. This circuit contains two inhibitory neurons, in the putamen and globus pallidus. Thus, a brief period of cortical excitation of the putamen (see neural responses in boxes marked cerebral cortex and striatum; Figure 14–7A) is transformed into an inhibitory message (pause in neural activity) in the internal segment of the globus pallidus because striatal neurons are inhibitory. However, because the output of the internal segment of the globus pallidus is also inhibitory, the amount of inhibition of the thalamus from the internal segment of the globus pallidus is reduced. Inhibition of an inhibitory signal is termed disinhibition; functionally, this double negative is equivalent to excitation. The thalamic response shown is transiently released from inhibition and fires a burst of action potentials. In a motor behavior such as reaching for a glass of water, neurons in premotor areas, as well as corticospinal tract neurons in primary motor cortex, are thought to be excited by the actions of the direct path.
The indirect path has the opposite effect on the thalamus and cerebral cortex as the direct path. Putamen neurons of the indirect path, which are inhibitory because they contain GABA, project to the external segment of the globus pallidus. Excitation of the striatal neurons inhibits the external segment of the globus pallidus (pause in action potentials). Because the output of the external segment of the globus pallidus is inhibitory, indirect path neurons of the putamen disinhibit the subthalamic nucleus (burst of action potentials). This disinhibition will excite the internal segment of the globus pallidus and substantia nigra pars reticulata (which are both inhibitory) and thereby increase the strength of the inhibitory output signal directed to the thalamus.
Dopamine excites striatal neurons of the direct path and inhibits striatal neurons of the indirect path. Despite these different actions on striatal neurons, the effect of dopamine on either path is to reduce the inhibitory output of the basal ganglia, thereby reducing inhibition of the thalamus. This effect promotes movement generation by the thalamocortical circuits.
The power of this model is that it helps to explain the mechanisms of some hypokinetic and hyperkinetic signs seen in basal ganglia disease. Dopamine is deficient in Parkinson disease, which produces hypokinetic signs. Reduced striatal dopamine in Parkinson disease would be expected to diminish the excitatory effects of the direct path on cortical motor areas and enhance the inhibitory effects of the indirect path (Figure 14–7C1). Together these effects would drastically reduce the thalamic signals to the cortex. For the premotor and motor cortical areas, this would reduce cortical outflow along the corticospinal and corticobulbar tracts and reduce production of motor behaviors (ie, hypokinesia).
In hyperkinetic disorders, the opposite changes take place (Figure 14–7C2): There are enhanced excitatory effects of the indirect path on the cortex. (Note that the output of the substantia nigra pars compactor may be normal.) In Huntington disease, recent studies suggest that striatal neurons of the indirect path, which contain both GABA and enkephalin, are lost (low neural response). This cell loss would result in greater thalamic outflow to the cortex by decreasing striatal inhibition of the external segment of the globus pallidus. Hemiballism, another hyperkinetic disorder, is produced by a subthalamic nucleus lesion. This nucleus normally exerts an excitatory action on the internal segment of the globus pallidus. When the subthalamic nucleus becomes lesioned, the internal segment of the globus pallidus would be expected to inhibit the thalamus less (thin dashed line), thereby increasing outflow to the cerebral cortex.
FIGURE 14–6
The neurotransmitters of the basal ganglia are shown in relation to the organization of basal ganglia circuits. Neurons in the striatum that contain GABA, substance P, and dynorphin (purple) give rise to the direct path, projecting to the internal segment of the globus pallidus. Neurons that contain GABA and enkephalin (green) give rise to the indirect path and project to the external segment of the globus pallidus. GABA, γ-aminobutyric acid; GPe, external segment of the globus pallidus; GPi, internal segment of the globus pallidus; SNc, substantia nigra pars compacta; SNr, substantia nigra pars reticulata; STN, subthalamic nucleus; VP, ventral pallidum; VTA, ventral tegmental area.

FIGURE 14–7
Functional basal ganglia circuits in health and disease. Summary of the direct (A) and indirect (B) paths of the healthy basal ganglia. Filled neuronal cell bodies and terminals indicate inhibitory actions, and open cell bodies indicate excitatory actions. Schematic action potential records are shown by each structure. The vertical line is an action potential; the horizontal line is the baseline. Neural activity for each circuit can be followed, beginning with a phasic excitatory input from the cortex and the resulting phasic change in the thalamus. Changes in activity in the diseased circuits are shown for hypokinetic (C1) and hyperkinetic (C2) neurological signs. The thickness of the lines indicates relative changes in the number of neurons and strength of connections. Thicker means stronger connections and more activity; thinner means fewer and weaker connections. Schematic neural responses are also shown. Unlike A and B, which are the neural responses to discrete cortical input signals, the responses in C1 and C2 reflect changes in continuous activation patterns produced by disease. These paths follow only tonic changes in neural activation, because phasic changes are not well characterized. GPe, external segment of the globus pallidus; GPi, internal segment of the globus pallidus; SNc, substantia nigra pars compacta; SNr, substantia nigra pars reticulata; STN, subthala-mic nucleus.


Knowledge of Basal Ganglia Connections and Neurotransmitters Provides Insight Into Their Function in Health and Disease
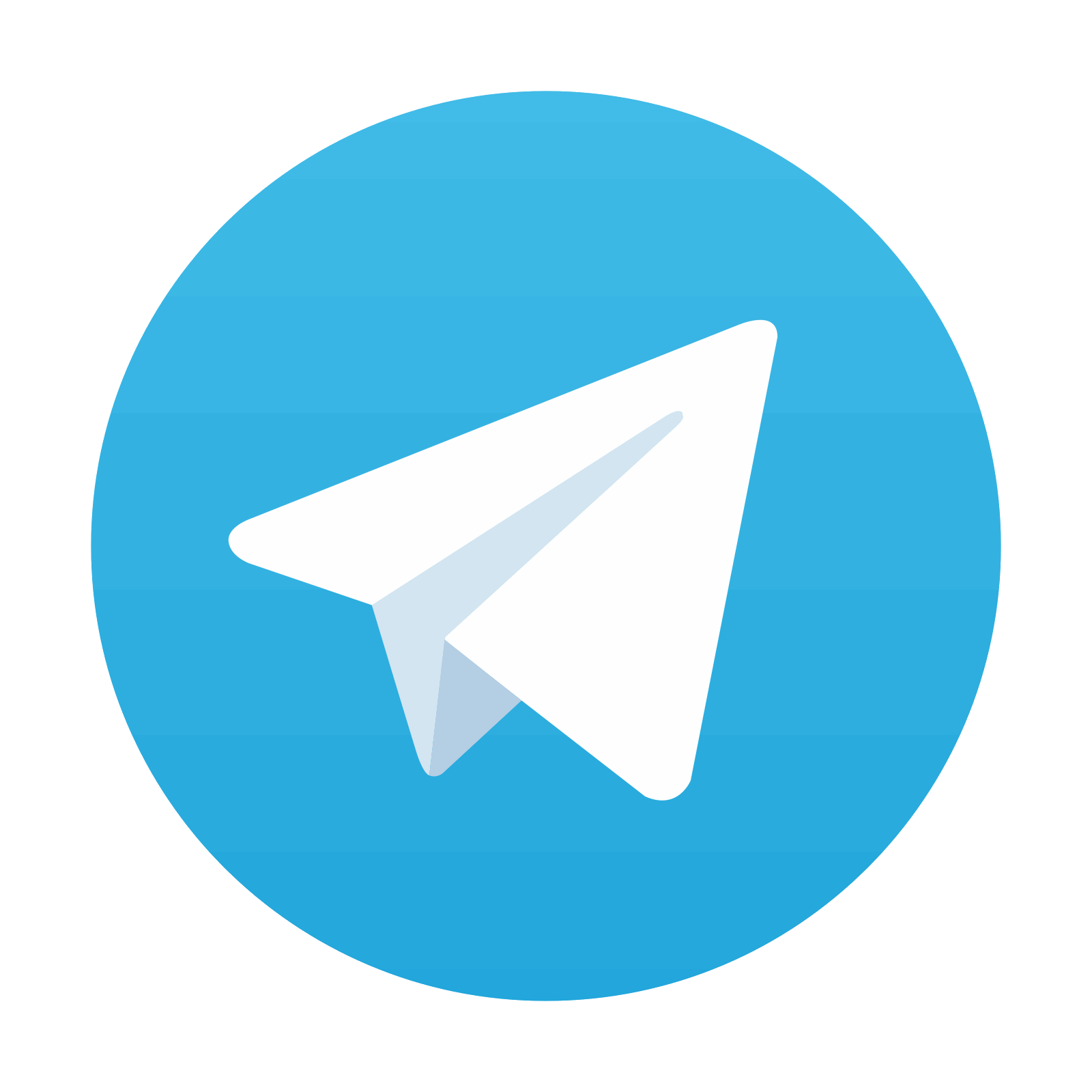
Stay updated, free articles. Join our Telegram channel

Full access? Get Clinical Tree
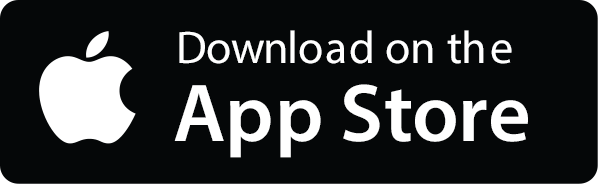
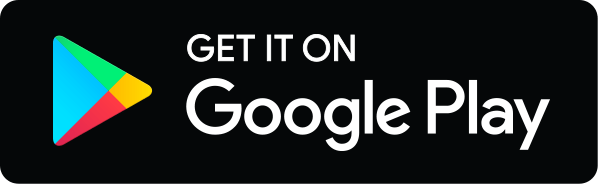