The biology of ageing
Alan H. Bittles
Introduction
Although old age is readily recognizable, methods to define and measure the underlying biological processes are much less amenable to study. For this reason, life expectancy has been widely used as a surrogate measure of ageing, as well as to monitor economic progress at national and regional levels. It is generally acknowledged that lifespan is a constitutional feature of the human phenotype, and twin studies have indicated that 25-33 per cent of the variance in human longevity is genetic in origin.(1,2) External factors including lifestyle can also exert a major influence, as illustrated by the current mean life expectancies of 79 and 86 years for males and females in Japan, whereas the comparable figures for Botswana are 35 and 33 years, respectively.
The importance of genetic inheritance as a determinant of extended survival has been illustrated by population level studies in Okinawa, an island prefecture of southern Japan with a very high prevalence of long-lived individuals. On the island, the mortality rates of the male and female siblings of centenarians were approximately half those of birth cohort-matched, non-centenarian siblings.(3) These findings parallel an earlier study of the family of Jeanne Calment, who died in France in 1997 aged 122 years. Of her 55 relatives, 24 per cent had lived to >80 years compared to just 2 per cent of a matched control group.(4) However, it remains unclear whether the enhanced lifespan of individuals who exhibit above average longevity is due to a slowing of the overall ageing process or is primarily associated with resistance to major life-threatening pathologies.
The concept of an ‘allostatic load’, potentially involving the neuroendocrine, sympathetic nervous, immune and cardiovascular systems, and metabolic pathways, has been advanced to describe the lifetime costs of adapting to physical and psychological stresses. According to this hypothesis, while the actions of biological mediators of stress can be initially beneficial to health, chronic stimulation results in regulatory imbalance and subsequent pathophysiological changes.(5) Empirical studies have indicated increased physiological dysregulation and functional decline at >70 years of age, which would imply that predicted global increases in the numbers of older persons will be accompanied by disproportionately larger groups of individuals with major age-related pathologies.
Theories of ageing
While initially popular, it became apparent that single, ‘magic bullet’ causes of ageing were inappropriate to complex biological species, and with this recognition earlier organ- and system-based theories have gradually been discounted. Conversely, the observation that ageing appears to be initiated at different ages and can proceed at different rates in individual members of a species provides presumptive evidence for the interaction of multiple genetic and non-genetic influences. Two main groups of theories have been formulated, genomic and stochastic, each subdivided into a number of discrete topic headings.
Genomic theories of ageing
Genomic theories premise that ageing is primarily associated with changes in the genetic constitution of the organism. Support for genomic theories stems largely from the characteristic life expectancies of mammalian and non-mammalian species, and theories proposing a primarily genetic basis for ageing were greatly strengthened by the demonstration that human diploid cells exhibited a highly reproducible lifespan when cultured in the laboratory. Although strong evolutionary advantages can be envisaged for genetic control of developmental changes up to and including reproductive adulthood, the existence of genes uniquely encoding ageing seems improbable since few free-living animals or humans have ever succeeded in attaining the maximum lifespan of their species.
(a) Information transfer
The ability to synthesize functional proteins is dependent on the fidelity of genetic information encoded in the DNA, its unimpaired transcription from DNA to RNA, and translation into peptides and proteins. As each of these processes is subject to inaccuracy, and during the life course of an organism the sequence of information transfer steps is continuously operational, the error potential is large. With increasing chronological age the probability of errors increases, resulting in the accumulation of deleterious mutations late in life.(6) Since a number of the proteins synthesized may be involved as surveillance enzymes to maintain the accuracy of the entire system, feedback mechanisms could lead to its collapse, resulting in a phenomenon initially termed error catastrophe.
(b) Somatic mutation
With the demonstration of an inverse correlation between the lifespan of mammalian species and the incidence of chromosome abnormalities, age-related physiological changes were originally ascribed to accumulated mutations in the nuclear DNA (nDNA) of somatic cells. Findings of this nature could, however, be explicable in terms of the ability of an organism to tolerate DNA damage via the repair of damaged molecules, with more than 130 human DNA repair genes identified.(7) The capacity of nDNA to resist attack by endogenous reactive species and environmental agents is therefore considerable, which casts doubt on the general applicability of the theory.
(c) Epigenetic mechanisms
Epigenetic errors, i.e. errors in the control of gene expression rather than mutations in DNA or protein, have been proposed as major primary causal factors in senescence.(8) In promoter regions of genes, hypermethylation silences a gene whereas the hypomethylation of previously methylated sequences permits their expression. The pattern of DNA methylation is established during development and is cell type-specific, and changes in methylation can occur both during ageing and in cancer cells. The advantage of epigenetic models of ageing is their lack of requirement for the evolutionary preservation of genes encoding ageing, which in former generations would seldom have been expressed.
(d) Mitochondrial decline
Mitochondria are subcellular organelles responsible for aerobic energy production in humans and many other species. The mitochondrial genome of ˜16.5 kb is characterized by its extremely compact organization, with no protective histones, a lack of excision or recombinational repair mechanisms, and a virtual absence of introns, all of which make it highly susceptible to mutation. Mitochondrial DNA (mtDNA) plays a central role in mitochondrial propagation and the maintenance of cellular respiration, but a majority of proteins involved in the regulation of mtDNA transcription, translation and replication, and the mitochondrial respiratory chain, are encoded in the nuclear genome. This design requires the operation of a highly coordinated mechanism for the expression of the nuclear and mitochondrial genomes. The central role of mitochondria in energy production means that defects may be of major metabolic significance, and the demonstration of increased levels of mtDNA deletions and base-substitutions in aged human neurones, heart, and skeletal muscle suggest a causative role for mtDNA mutations in ageing.(9)
(e) Telomere loss
Telomeres are specialized structures located at the terminus of the DNA helix and critical to the maintenance of DNA stability and replication. The enzyme telomerase which is responsible for telomere synthesis is active during early embryonic and foetal development but its activity is down-regulated in all human somatic cells before birth. As human diploid fibroblasts in culture were shown to progressively lose telomeres, it was hypothesized that telomere length could act as a predictor of the potential in vitro lifespan achievable by a cell strain.(10) Humans have a common telomere profile found on lymphocytes, amniocytes, and fibroblasts which appears to be preserved throughout life. However, the rate of telomere loss with ageing varies between chromosomes and there is evidence that, in addition to the common human telomere profile, each person exhibits an individual profile.(11) Besides ageing, telomere loss has been implicated in a wide range of disease states, including heart disease, stroke, infection, long-term chronic stress, and obesity. Given the apparent relationship between telomere loss and both ageing and age-related pathologies, pharmacological activation of telomerase has been proposed as a potential treatment for chronic or degenerative diseases.(12) As tumour tissue and transformed cells constitutively produce telomerase, any therapeutic intervention of this nature would require careful monitoring.
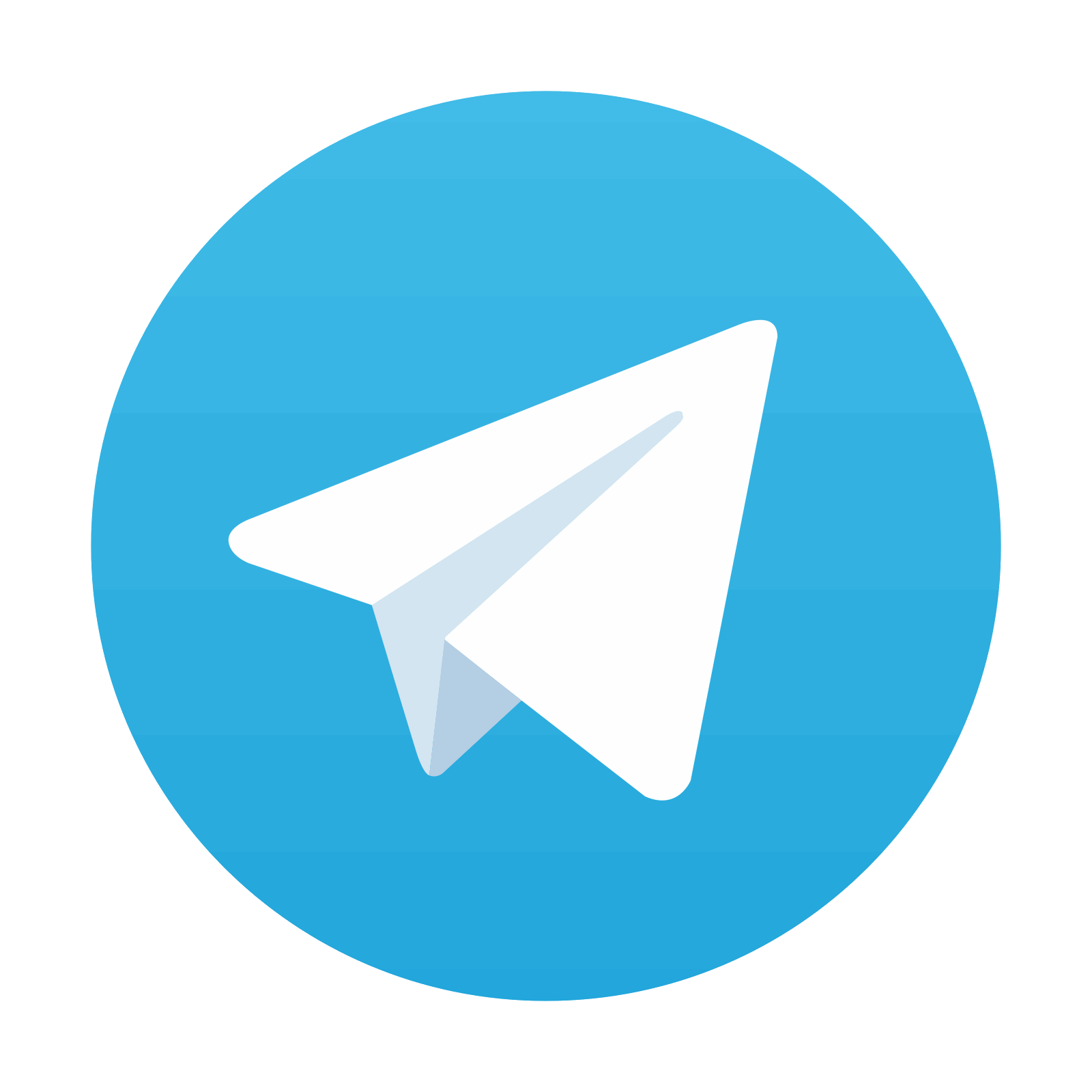
Stay updated, free articles. Join our Telegram channel

Full access? Get Clinical Tree
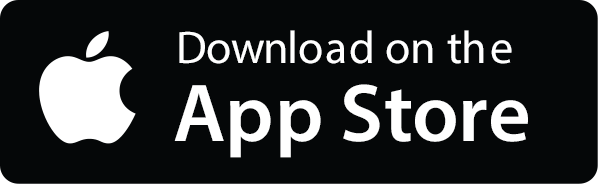
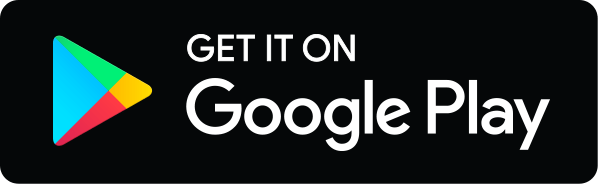
