Figure 27-1. Lateral (A) and posterior (B) views of the brainstem showing the inferior (dark green and red), middle (light green), and superior (blue) cerebellar peduncles. The inferior peduncle is composed of juxtarestiform (dark green) and restiform (red) bodies. Superior view (C) showing the positions and relationships of the three cerebellar peduncles in a brain specimen. Cranial nerves are identified by Roman numerals.
Table 27-1 Synopsis of Selected Afferent and Efferent Fibers of the Cerebellum as Contained in or Associated with the Cerebellar Peduncles
−, uncrossed; X, crossed; (−), some uncrossed; (X), some crossed.
The basilar pons, which is located inferior to the exiting roots of the trigeminal nerve, is continuous into the middle cerebellar peduncle (brachium pontis), which is located superior to the exiting roots of the fifth cranial nerve (Figs. 27-1A–C and 27-2). These exiting roots represent the boundary between the basilar pons and the middle cerebellar peduncle. This large peduncle mainly conveys pontocerebellar fibers arising from the pontine nuclei of the basilar pons into the cerebellum.
Figure 27-2. Axial magnetic resonance image of the cerebellum and pons showing the relationship between the root of the trigeminal nerve and the basilar pons (inferior to the root) and the middle cerebellar peduncle (superior to the root). Compare with Figure 27-1.
The superior cerebellar peduncle (brachium conjunctivum) sweeps rostrally out of the cerebellum and penetrates into the midbrain just caudal to the exit of the trochlear nerve (Fig. 27-1A–C). Within the midbrain, these fibers cross the midline as the decussation of the superior cerebellar peduncle at the level of the inferior colliculus. This bundle contains predominantly cerebellar efferent fibers that originate from neurons of the cerebellar nuclei and distribute to the diencephalon and brainstem.
Cerebellar Lobes, Lobules, and Zones
At the most general level, it is common to divide the cerebellum into a narrow midline vermis and expansive lateral hemispheres (Figs. 27-3 and 27-4 [see pp. 374-375]). The cerebellum is further divided into anterior, posterior, and flocculonodular lobes by the primary and posterolateral fissures, respectively. The lobes of the cerebellum are composed of yet smaller divisions called lobules (Figs. 27-3 and 27-5 [see p. 375]). The lobules of the vermis are identified by Roman numerals I to X; the corresponding lateral (hemisphere) portion of each vermis lobule is identified by the same Roman numeral but with the prefix H (Figs. 27-3 and 27-5). Vermis lobules II to X have hemisphere portions HII to HX (Fig. 27-5); lobule I does not have a hemisphere part in humans. The anterior lobe comprises lobules I to V and HII to HV, and the posterior lobe, lobules VI to IX and HVI to HIX. The flocculonodular lobe consists of the nodulus (lobule X) and its hemisphere counterpart, the flocculus (lobule HX). In turn, each lobule consists of a series of individual ridges of cortex called folia (singular, folium).
Figure 27-3. Unfolded view (see upper right) of the cerebellar cortex showing lobes, lobules (by name and number), and main fissures (printed in blue). The lobules of the hemisphere are designated by the prefix H, to show which lobule of the hemisphere is continuous with its corresponding (designated by the Roman numeral) vermal lobule.
Figure 27-4. Rostral (A, superior aspect), caudal (B, inferior aspect), anterior or ventral (C, with brainstem removed), and medial sagittal (D) views of the cerebellum compared with corresponding views of the cerebellum in magnetic resonance imaging (E to J). In rostral views (A and E, J), the folia of the anterior lobe can characteristically be followed across the midline. The tonsil and its close relationship with the medulla are seen in inferior views (B and G, H); the peduncles and the lobes are clearly evident in anterior (ventral) views (C and H, I). The medial sagittal views (D and J) reveal the relationship of the cerebellum to the fourth ventricle and brainstem.
Figure 27-5. An unfolded view of the cerebellum showing medial (gray), intermediate (green), and lateral (blue) zones on the right and the nuclei with which each zone is functionally associated on the left in the corresponding color. The general areas of cortex and nuclei served by the cerebellar arteries are also indicated on the left. Roman numerals indicate lobules of the vermis; numerals preceded by H indicate the corresponding lobules of the hemisphere.
The individual folia are continuous from one hemisphere to the other, across the midline on the superior cerebellar surface (Fig. 27-4A, E, F). This pattern, obvious on the superior cerebellar surface, is disrupted on the inferior surface by the enlargement of the lateral parts of the cerebellum and consequent infolding of the midline area (Fig. 27-4B, G, I).
Superimposed on the lobes and lobules of the cerebellum are rostrocaudally oriented cortical zones that are defined on the basis of their connections. There are three principal zones on each side: the medial (vermal), intermediate (paravermal), and lateral (hemisphere) zones (Fig. 27-5). On the basis of their afferent and efferent connections, these three larger cortical zones can be subdivided further into nine smaller zones. In general, these zone patterns are the basis for the modules discussed later in this chapter. The clinical deficits that result from a cerebellar lesion depend mainly on which of the three principal zones is involved; consequently, the three-zone terminology is used in this chapter.
The medial (vermal) zone is a narrow strip of cortex adjacent to the midline that extends throughout anterior and posterior lobes and includes the nodulus (Figs. 27-4A, B and 27-5). This zone is widest in lobule VI and tapers rostrally and caudally. The intermediate (paravermal) zone lies adjacent to the medial zone and extends throughout anterior and posterior lobes but has little representation in the flocculonodular lobe (Fig. 27-5). The lateral (hemisphere) zone occupies by far the largest part of the cerebellar cortex. It includes large portions of anterior and posterior lobes and the flocculus (Figs. 27-3 and 27-5).
Cerebellar Nuclei
The four pairs of cerebellar nuclei are located within the white matter core of the cerebellum and are accessed easily by fibers traveling to and from the overlying cortex (Figs. 27-5 and 27-6). The fastigial (medial cerebellar) nucleus lies immediately adjacent to the midline and is functionally related to the overlying medial zone of the cerebellar cortex. Lateral to the fastigial nucleus are the two interposed nuclei: the globose (posterior interposed) nucleus and the emboliform (anterior interposed) nucleus. These nuclei are functionally related to the intermediate zone of the cortex. Lateral to the emboliform nucleus is the dentate (lateral cerebellar) nucleus, which appears as a large, undulating sheet of cells shaped like a partially crumpled paper bag. Its opening, the hilus, is directed anteromedially (Fig. 27-6). This nucleus is functionally related to the lateral zone of the cortex; its large size correlates with the large size of this cortical zone.
Figure 27-6. The cerebellar nuclei in cross section, drawn from a slide. The color coding of each nucleus corresponds to its appropriate zone in Figure 27-5.
Most of the signals that leave the cerebellum do so via axons that arise in the cerebellar nuclei; the remainder travel on fibers that originate in the cerebellar cortex. Collectively, axons that arise in the cerebellar nuclei constitute cerebellar efferent projections (e.g., cerebellothalamic fibers). These axons originate from cells in the cerebellar nuclei and use one of the excitatory neurotransmitters, glutamate or aspartate, and thus function to activate their targets. The juxtarestiform body contains fibers that arise from the cerebellar cortex, form the cerebellar corticovestibular projection, use the inhibitory neurotransmitter GABA, and inhibit their targets. The fastigial nuclei generally project bilaterally to the brainstem through the juxtarestiform bodies. Fibers originating in the dentate, emboliform, and globose nuclei exit the cerebellum via the superior cerebellar peduncle and cross in its decussation.
Some neurons in each cerebellar nucleus send axons or axon collaterals into the overlying cortex, where they terminate in the granular layer as mossy fibers. These axons are called nucleocortical fibers, and they exert an excitatory influence on the cerebellar cortex.
Blood Supply to Cerebellar Structures
The blood supply to the cerebellar cortex, nuclei, and peduncles is via the posterior inferior cerebellar artery (PICA), anterior inferior cerebellar artery (AICA), and superior cerebellar artery (Figs. 27-5 and 27-7 [see p. 376]). The PICA originates from the vertebral artery and supplies the posterolateral medulla (including the restiform body), the choroid plexus of the fourth ventricle, and caudomedial regions of the inferior cerebellar surface (including the vermis) (Figs. 27-5 and 27-7). Caudal parts of the middle cerebellar peduncle, the choroid plexus extending out of the foramen of Luschka, and the caudolateral portions of the inferior cerebellar surface are served by the AICA. This vessel may also supply caudal parts of the dentate nucleus (Fig. 27-5). The entire superior surface of the cerebellum, most of the cerebellar nuclei, the rostral parts of the middle cerebellar peduncle, and the superior cerebellar peduncle are served by the superior cerebellar artery (Fig. 27-7).
Figure 27-7. Origin and course of arteries serving the cerebellum as seen in lateral aspect.
CEREBELLAR CORTEX
On histologic examination, each folium of the cerebellum has a superficial cellular layer, the cerebellar cortex, and a core of myelinated fibers traveling to (afferent) or from (efferent) the overlying cortex. The cortex consists of a Purkinje cell layer insinuated between a cell-dense inner region immediately adjacent to the white matter core, the granule cell layer, and an outer pale and relatively cell sparse portion, the molecular layer (Figs. 27-8 and 27-9 [see p. 377]).
Figure 27-8. Midsagittal view (A) of the cerebellum showing the main fissures and lobules and a detail (B) of the cortex showing the cortical layers as seen in a neutral red–stained section. Arrows point to representative Purkinje cell bodies.
Figure 27-9. Cell types and synaptic relations in the cerebellar cortex in transverse and sagittal planes. Note the structure of the cerebellar glomerulus (lower left) and the interaction of parallel and climbing fibers (upper right) with the dendritic processes of Purkinje cells. Compare with Figure 27-10. Because of their regional specificity, the unipolar brush cells are not shown (see Fig. 27-11).
Purkinje Cell Layer
The large (40 to 65 µm in diameter) somata of Purkinje cells form a single layer at the interface of the granular and molecular layers (Figs. 27-8 to 27-10 [see p. 378]). Each Purkinje cell gives rise to an elaborate dendritic tree that radiates into the molecular layer. This dendritic tree is shaped like a fan with its wide flattened aspect oriented perpendicular to the long axis of the folium (Figs. 27-9 and 27-10). The “trunk” of the tree is a single primary dendrite, which gives rise to several secondary dendrites, which in turn branch into many tertiary dendrites. Synaptic contacts are formed mainly on short terminal branchlets that emerge primarily from the secondary and tertiary dendrites (Figs. 27-9 and 27-10). There are two types of branchlets. Smooth branchlets emerge from secondary and tertiary dendrites, whereas spiny branchlets (gemmules) arise mainly from tertiary dendrites.
Figure 27-10. Examples of cells of the cerebellar cortex (see Fig. 27-9). The Purkinje cells are shown in sagittal (A; note the beaded appearance of the dendrites) and transverse (B; note the many parallel fibers) planes. Granule cell (C and D) dendrites end as a cluster of short, claw-like processes. Dendrites of Golgi cells (D) branch into molecular and granular layers, whereas their axons (D, beaded structures) ramify in only the granular layer. Mossy fibers (E) branch profusely and have many rosettes; synaptic contacts between mossy fibers and granule cell dendrites take place at the rosette in the cerebellar glomerulus (see Fig. 27-9). At the ultrastructural level (F), the Purkinje cell dendrite is surrounded by the numerous small profiles of parallel fibers. BF, Bergmann fiber, a type of glial cell process. (A to D Courtesy of Dr. José Rafols, Wayne State University.)
Purkinje cells are the only efferent neurons of the cerebellar cortex. Axons of Purkinje cells arise from the basal aspect of its pear-shaped cell body and may give rise to recurrent collaterals. These axons traverse the granular layer and the subcortical white matter to eventually terminate in either the cerebellar or the vestibular nuclei. Purkinje cells projecting into the cerebellar nuclei (as cerebellar corticonuclear fibers) arise from all areas of the cortex, whereas those projecting into the vestibular nuclei (as cerebellar corticovestibular fibers) originate primarily from parts of the vermis and the flocculonodular lobe. Purkinje cells release γ-aminobutyric acid (GABA) at their synaptic terminals and inhibit target neurons in the cerebellar and vestibular nuclei.
Granule Cell Layer
There are three types of neuron cell bodies within the granule layer. These are granular cells, which are extraordinarily numerous and found in all areas of the cerebellar cortex; Golgi cells, which are larger and also widely distributed; and unipolar brush cells, which are small neurons that have a restricted geographic distribution within the cortex.
The most numerous neuron of the granule cell layer is the small (5 to 8 µm in diameter) granule cell (Figs. 27-8, 27-9, and 27-10C). The dendrites of these cells form claw-like endings (dendritic digits) that ramify in the vicinity of the cell body. Their axons ascend into the molecular layer, where they bifurcate to form parallel fibers. As indicated by their name, parallel fibers run parallel to the long axis of the folium. Consequently, these fibers pass through the fan-like dendritic trees of the Purkinje cell and, when doing so, make synaptic contacts with spiny branchlets (Figs. 27-9 and 27-10B, F). They also synapse with the cells intrinsic to the molecular layer, such as basket and stellate cells. The distance spanned by the parallel fibers of a granule cell ranges from 0.3 to 5.0 mm, and the number of Purkinje and other cells contacted varies accordingly. Granule cells use glutamate (or perhaps aspartate) as their neurotransmitter and thus have an excitatory effect on their target cells. In fact, the granule cells are the primary excitatory neurons of the cerebellar cortex. The unipolar brush cell is also excitatory but is found in strikingly fewer numbers and is restricted in its distribution within the cortex. All of the other neurons of the cerebellar cortex, as we shall see, are inhibitory.
The second cell type of the granular layer is the Golgi cell. The soma of this neuron is larger (at 18 to 25 µm in diameter) than that of the granule cell and is usually found in the granular layer adjacent to the Purkinje cells (Figs. 27-9 and 27-10D). Dendrites of Golgi cells branch in the granular layer but extend primarily into the molecular layer without regard to plane of orientation. Axons of Golgi neurons branch in the granular layer and form synaptic contacts on granule cell dendrites (Fig. 27-9). The Golgi cell uses GABA as a neurotransmitter and is an inhibitory interneuron in the cerebellar cortex.
The third neuronal type found within the granule layer is the unipolar brush cell. This neuron has a slightly oval cell body, measuring 9 to 14 µm in diameter at the equator, and a single stout dendritic structure arising from the soma (Fig. 27-11; see p. 379). This dendritic process ends in a brush-like configuration made up of a cluster of dendrioles. The axon of the unipolar brush cell arises from the cell body, ramifies within the granular layer, and ends as a series of two to four synaptic structures called unipolar brush cell rosettes (Fig. 27-11). In contrast to granule cells, which are distributed throughout the cerebellar cortex, the unipolar brush cells are found primarily in the flocculonodular lobe, the adjacent areas of the paraflocculus, the vermis, and (in very sparse numbers) in the hemisphere lobules HVI to HVIII. Mossy fibers arising from primary and secondary vestibulocerebellar fibers and Golgi cell axons contact the dendrioles (Fig. 27-11B). The unipolar brush cell rosette is contacted by granule cell dendrites, dendrioles of the unipolar brush cell, Golgi cell axons, and an occasional Golgi cell dendrite (Fig. 27-11B). Unipolar brush cells use glutamate as their neurotransmitter and are therefore excitatory to their targets.
Figure 27-11. The unipolar brush cell of the granular layer of the cerebellar cortex. This cell has a comparatively small soma compared with Purkinje and granule cells (A). The cell body, dendrioles, and axon all ramify within the granular layer (B) and establish synaptic contact with mossy fibers, granule cells, and Golgi cells. This cell is restricted to vestibulocerebellar structures. (A Courtesy of Dr. Enrico Mugnaini, Northwestern University Institute for Neuroscience.)
The classic cerebellar glomerulus is a synaptic complex found throughout all portions of the cerebellar cortex within the neuropil of the granular layer. The glomerulus includes granule cell and Golgi cell dendrites and Golgi cell axons and a specialized synaptic segment (the mossy fiber rosette) of a mossy fiber, one type of cerebellar afferent axon (Figs. 27-9 and 27-10C–E). The mossy fiber rosette is centrally located and forms synapses with several granule cell dendrites. Golgi cell axons contact granule cell dendrites in the glomerulus, and the entire complex is encapsulated by glial cell processes.
Within the flocculonodular lobe and some adjacent cortical areas, there are many cerebellar glomeruli of the classic arrangement described before. In addition, this cortical area also contains glomeruli that are based on the unipolar brush cell rosette, in which case they may be called, in these specific cortical areas, unipolar brush cell glomeruli (Fig. 27-11B). This specific type of glomerulus is composed of the brush cell rosette, small contacts made by granular cell dendrites, larger contacts made by brush cell dendrioles, and small contacts by Golgi cell axons at the periphery of the glomerulus (Fig. 27-11B). Because unipolar brush cells and their synaptic interactions are located primarily in the flocculonodular lobe and vermis and because these cells receive vestibular inputs, their function is most likely to be related to cerebellar (and vestibular) control of eye movements, vestibuloocular reflexes, and various postural mechanisms.
Molecular Layer
The molecular layer has considerably fewer cell bodies than the granule cell layer (Fig. 27-8) but has proportionately more cell processes. These processes include parallel fibers, Purkinje cell dendrites, Golgi cell dendrites, climbing fibers, and the processes of cells intrinsic to the molecular layer (Fig. 27-9).
The intrinsic cell types of the molecular layer are stellate cells and basket cells (Fig. 27-9
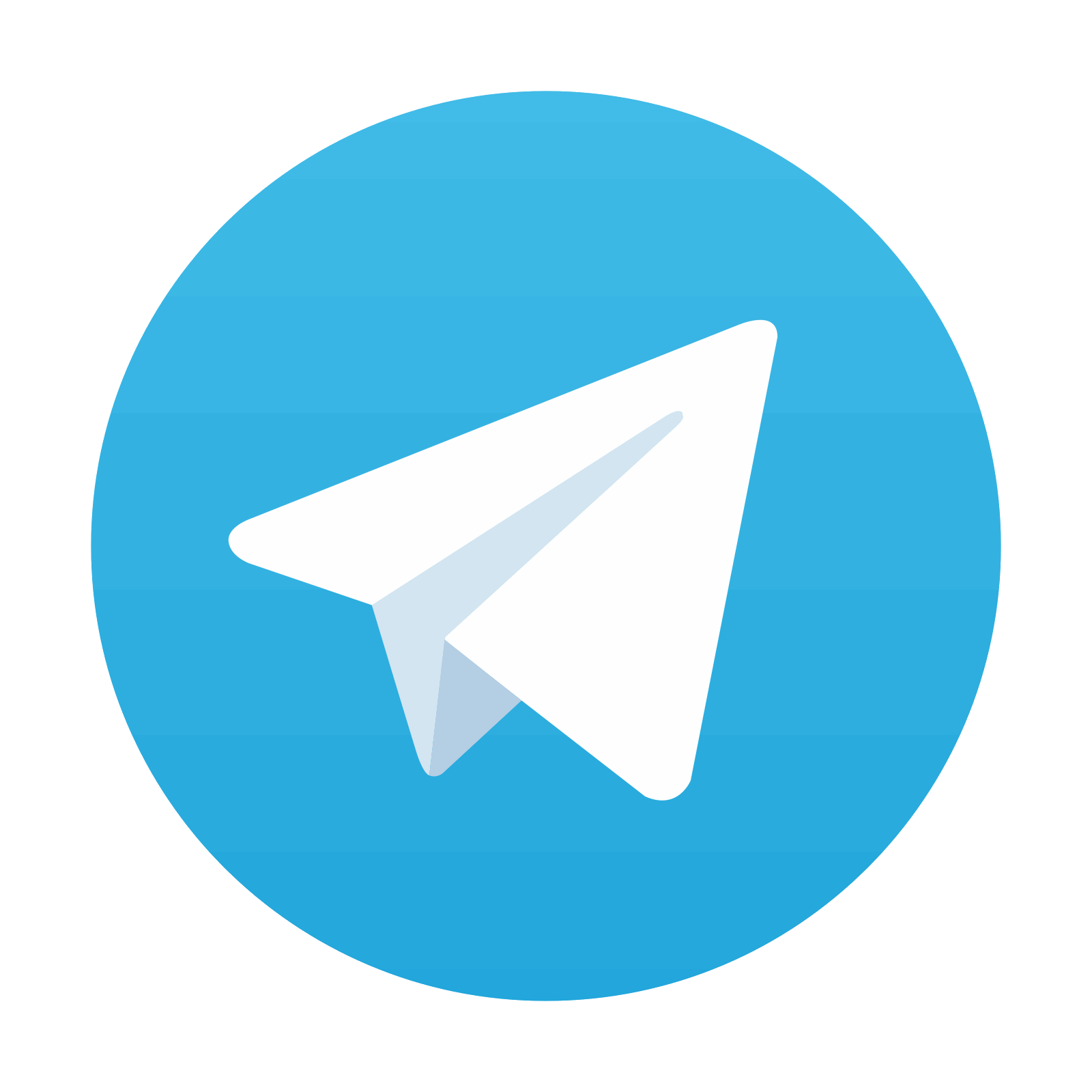
Stay updated, free articles. Join our Telegram channel

Full access? Get Clinical Tree
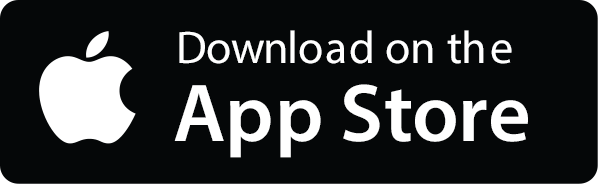
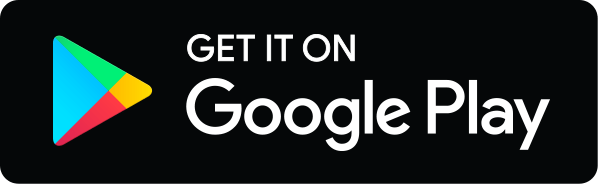