FIGURE 25.1 A lateral view of some of the clinically relevant cortical areas from the Brodmann cytoarchitectonic map. (Modified from Kiernan JA. Barr’s The Human Nervous System: An Anatomical Viewpoint. 7th ed. Philadelphia: Lippincott Williams & Wilkins, 1998, with permission.)
FIGURE 25.2 A medial view of some of the clinically relevant cortical areas from the Brodmann cytoarchitectonic map. (Modified from Kiernan JA. Barr’s The Human Nervous System: An Anatomical Viewpoint. 7th ed. Philadelphia: Lippincott Williams & Wilkins, 1998, with permission.)
The premotor region (area 6), located just rostral to area 4, is closely related to the motor cortex, both anatomically and functionally. The premotor cortex is similar histologically to the motor cortex but lacks the giant pyramidal cells. Some fibers from area 6 pass to area 4 and then downward with the CST; others descend with the pyramidal fibers. There is probably less complete crossing of the fibers from the premotor cortex than of those from the motor cortex. In addition, the premotor region communicates with the basal ganglia and other portions of the extrapyramidal system, including the subthalamic nucleus, red nucleus, superior colliculus, vestibular nuclei, inferior olive, and brainstem reticular formation (Figure 25.3).
FIGURE 25.3 Diagrammatic sketch of the major components of the corticospinal and extrapyramidal systems. (Modified from Benda CE, Cobb S. On the pathogenesis of paralysis agitans (Parkinson’s disease). Medicine 1942;21:95–142.)
The CST is important in controlling discrete, isolated motor responses, especially fine voluntary movement of individual digits. The CST provides speed and agility of distal extremity movements. The premotor cortex and its pathways are concerned with larger coordinated responses, with more stereotyped movements that are partly automatic and involve the trunk and the proximal limbs, and with postural mechanisms. It is the principal cortical component of the extrapyramidal system. Stimulation of area 6 causes contraversive movements of the head and trunk. The premotor region is involved in movements guided by visual, auditory, and somatosensory stimuli. The supplementary motor area (M-II) is part of area 6 that lies on the medial aspect of the frontal lobe just anterior to the paracentral lobule (area 6ab). It communicates with the primary motor cortex as well as with the supplementary motor cortex in the opposite hemisphere. M-II seems to be involved particularly in planning and integrating bilateral body movements. M-II is also somatotopically organized, but the homunculus is cruder and less detailed than the one in M-I. The cingulate motor area in the anterior half of the cingulate gyrus projects to the primary motor cortex and also contributes descending fibers to the corticobulbar and CSTs. There is also a secondary motor area in the depths of the central sulcus where the precentral and postcentral gyri merge.
Axons from the motor neurons of the precentral gyrus descend through the corona radiata and the posterior limb of the internal capsule where corticobulbar fibers are anterior, followed posteriorly by those to the upper extremity, trunk, and lower extremity. About 90% of the CST fibers are small myelinated axons with a diameter of 1 to 4 μm, and most of the remaining fibers are 5 to 10 μm in diameter. The small number of fibers that arise from Betz cells are very large, with diameters of 10 to 22 μm. The posterior limb of the internal capsule is that portion of the capsule between the lenticular nucleus and the thalamus. The retrolenticular part lies posterior to the lenticular nucleus. In the rostral part of the internal capsule, the corticospinal fibers lie in the anterior portion of the posterior limb. As the capsules descend, the corticospinal fibers move posteriorly and come to occupy a position in the posterior third to posterior quarter of the posterior limb. In normal individuals, the CST can often be visualized as a subtle hyperintensity in the posterior portion of the posterior limb of the internal capsule on T2-weighted axial magnetic resonance images. These areas of hyperintensity are found near the junction of the posterior limb and the retrolenticular portion of the capsule. The signal change is thought to mark the very large heavily myelinated, rapidly conducting fibers that constitute the Betz cell component of the pyramidal tract. Degeneration of the CST, as occurs in amyotrophic lateral sclerosis or primary lateral sclerosis, accentuates this signal change and may be useful diagnostically (Figure 25.4).
FIGURE 25.4 Wallerian degeneration of the pyramidal tract following intracerebral hemorrhage. A long band of increased signal intensity traces the anatomical course of the pyramidal tract from the lesion in the internal capsule (left panel, solid arrow) down to the left cerebral peduncle (open arrow), reaching the pons (arrowhead) and the medulla oblongata (right panel, curved arrow). (Reprinted from Dol JA, Louwerse ES. Images in clinical medicine. Wallerian degeneration of the pyramidal tract on magnetic resonance imaging. N Engl J Med 1994;331:88, with permission.)
At the midbrain level, pyramidal fibers traverse the middle three-fifths of the cerebral peduncle, with corticobulbar fibers most medial (Figure 25.5). The majority of corticobulbar fibers decussate before synapsing with the specific cranial nerve nuclei, but most of the cortical innervation of brainstem centers is both crossed and uncrossed. The descending fibers then pass through the basilar portion of the pons as disjointed fascicles and enter the medulla. In the caudal medulla, the CSTs gather into the discrete twin columns of the medullary pyramids that make up the base of the medulla (Figure 11.11). Approximately 85% to 90% of the fibers cross in the decussation of the pyramids, with those destined for the upper extremity decussating more rostrally than those for the lower extremity (Figure 11.12). There is considerable variation in the proportion of crossed and uncrossed CST fibers in man. Instances of ipsilateral hemiplegia due to an uncrossed pyramidal tract have been reported. Terakama et al. used functional magnetic resonance imaging, motor evoked potentials, and somatosensory evoked potentials to investigate a patient with an ipsilateral hemiplegia following a cerebral hemorrhage. They were able to demonstrate that the affected limbs were controlled by the ipsilateral cerebral cortex. In some instances, an uncrossed CST is associated with congenital anomalies of various sorts.
FIGURE 25.5 The corticobulbar and corticospinal pathways.
The fibers that decussate descend in the lateral funiculus of the spinal cord in the lateral CST, lying anterolateral to the posterior gray horn, medial to the posterior spinocerebellar tract, and posterior to the plane of the denticulate ligaments to supply the muscles of the opposite side of the body. In the lumbosacral cord, where there is no posterior spinocerebellar tract, the lateral CST abuts the surface of the cord. The lateral CSTs may also contain other corticofugal fibers as well as some ascending ones. About 50% of the fibers of the lateral CST terminate in the cervical region, 20% in the thoracic area, and 30% in the lumbosacral portion of the cord. The tract ends at about the S4 level. The smaller anterior CST usually contains about 10% to 15% of the corticospinal fibers; it descends uncrossed in the ipsilateral anterior funiculus and usually does not extend below the midthoracic region. These fibers cross in the anterior white commissure at the segmental spinal cord level before terminating; they primarily supply axial muscles.
The axons of the corticobulbar and CSTs terminate on motor nuclei of the cranial nerves and the anterior horn cells of the spinal cord. Those traveling to the cord terminate primarily in laminae IV to VI, lamina VII, lamina VIII, and lamina IX on the side opposite the hemisphere of origin. Fibers from areas 4 and 6 end more ventrally, mainly in laminae VII and VIII, and are concentrated in the cervical and lumbosacral enlargements. The majority of CST fibers synapse on an interneuron, but about 10% end directly on alpha motor neurons in lamina IX. Those fibers that project directly from the cortex to anterior horn cells mediate discrete, skilled, fine motor movements of the distal extremities. Impulses then travel from the brainstem motor nuclei and anterior horn cells to the neuromuscular junctions of striated muscles (final common pathway). A single corticospinal fiber innervates more than one neuron in the spinal cord, and some probably innervate many. The pyramidal tract affects the activity of both alpha and gamma motor neurons. Alpha gamma coactivation serves to maintain a consistent level of stretch on the intrafusal muscle fibers during contraction and relaxation of the extrafusal fibers.
The CST preferentially innervates certain muscles, and this “pyramidal distribution” is important clinically (see next section on p. 332). Lateral vestibulospinal tract effects are largely the opposite of CST effects. Glutamate or aspartate may be the excitatory neurotransmitters in some corticospinal neurons. The fibers from the parietal lobe that descend in the pyramidal tract terminate on sensory neurons in the dorsal horn and play a role in modulating sensory impulses in the long, ascending sensory pathways.
Neuronal discharge of the primary motor cortex, as from electrical stimulation or seizure activity, causes muscular contractions on the opposite side of the body. The response is one of groups of muscles rather than simple contraction of isolated muscles, but individual muscles as well as movements may be finely represented in the motor cortex. Stimulation of area 4 can cause discrete movements of the digits and the muscles supplied by the cranial nerves. With some overlap, areas controlling movements of the thumb, index finger, hallux, and face have the widest distribution and the lowest threshold. Stimulation of area 6 also causes a contralateral motor response, but a stronger stimulus is necessary than when area 4 is stimulated. The resulting movements are more complex and consist of slow, synergistic, postural, or patterned contractions of a generalized type that involve large muscle groups.
The CST lesions induced by ablation experiments in animals cause various deficits, depending on the animal and the area ablated. From a phylogenetic standpoint, voluntary motor control is extrapyramidal in submammalian species, mixed pyramidal/extrapyramidal in nonhuman mammals, and essentially pyramidal in humans. The effects of medullary pyramid transection (pyramidotomy) illustrate the differences in the function of the CST in different species. In the chimpanzee, it causes paralysis of the whole limb. In the rhesus monkey, the same lesion causes paralysis of distal extremity muscles with permanent loss of independent hand and finger movements. In the cat, it causes minimal deficits. Because of differences between the effects of lesions in man and those in experimental animals, such experiments have been of only limited usefulness in understanding human CST pathophysiology.
Questions remain about the relationship of the CST to spasticity. Primarily because of animal experimentation, it has been thought that lesions restricted to the pyramidal tract may cause weakness but not spasticity or hyperreflexia. The increase in tone that follows CST lesions may be related more closely to dysfunction of the extrapyramidal rather than the pyramidal system or to interruption of corticofugal fibers other than pyramidal in the corticospinal pathway. Although experimental pyramidotomies in animals may not lead to spasticity, in humans the evidence is that spasticity does eventually develop in the majority of cases. This is true even after very restricted lesions involving the medullary pyramids, although after a longer interval than is typical of other pyramidal lesions. It is likely that early flaccidity followed by the development of spasticity occurs with infarction of the CST at any level. More slowly developing lesions are likely to manifest spasticity at the time of initial presentation. Spasticity probably results from imbalance of the facilitatory and inhibitory centers in the midbrain and brainstem reticular formations, as well as altered balance between the alpha and gamma motor systems in the spinal cord. The reduction in the threshold and exaggeration of the stretch reflexes, an essential aspect of spasticity, may be mediated by the reticulospinal and vestibulospinal rather than the CSTs.
Hemiplegia in man is often produced by combined lesions of the motor and premotor components of the upper motor neuron. Various pathologic responses—such as the Babinski, Chaddock, and Hoffmann signs—appear. The affected extremities may at first be flaccid with depressed reflexes, but spasticity and reflex exaggeration typically develop within a few days. The pathologic reflexes may remain permanently.
CLINICAL MANIFESTATIONS OF DISEASE OF THE CORTICOSPINAL LEVEL
The corticospinal pathways may be involved in diverse disease processes, including vascular disease, neoplasm, degeneration, trauma, and others. The essential manifestations of a CST lesion consist of loss of skilled voluntary movements, or impairment of integration of movements, along with an overactivity of lower segmental centers due to disinhibition. The loss of voluntary movement is accompanied by increased tone in the involved muscles. The paresis from a lesion involving the pyramidal tract tends to involve entire extremities or certain muscle groups. Pyramidal lesions disrupt movements; any muscle that participates in the movement will be weakened, regardless of its specific lower motor neuron innervation. In contrast, lower motor neuron lesions involve muscles innervated by a specific structure, such as a nerve root or peripheral nerve. For instance, a CST lesion causes weakness of external rotation of the shoulder. This movement is carried out by both the infraspinatus and teres minor muscles. The teres minor is innervated by the axillary nerve, and the infraspinatus by the suprascapular nerve; both are weakened despite their different segmental innervations.
Pyramidal tract lesions do not cause the sort of severe, early focal muscle atrophy seen in lower motor neuron lesions, but there may be some mild, late atrophy of the involved part due to disuse (see Chapter 29). With lesions that are congenital or occur early in life, the involved limbs may fail to grow normally, resulting in hemiatrophy to varying degrees in adulthood. Such hemiatrophy may be difficult to detect; comparing the size of the thumbnails is a traditional technique for detecting subtle hemiatrophy. When atrophy does occur with CST disorders, it usually affects the small muscles of the hand. There are no fasciculations.
Deep tendon (muscle stretch) reflexes—rather than being lost as is the usual case with neurogenic atrophy due to a lower motor neuron lesion—are increased, and clonus may be elicited (see Chapter 38). The superficial reflexes are diminished or absent (see Chapter 39). Various pathologic reflexes, such as the Babinski sign, often termed pyramidal reflexes or upper motor neuron signs, often appear (see Chapter 40). Normal associated movements may be lost, and abnormal associated movements may be present (see Chapter 42). Trophic changes are uncommon, but occasionally, there is edema, desquamation, pigmentary changes, or glossy skin.
A pyramidal tract lesion causes weakness in a characteristic distribution. Facial weakness is limited to the lower face, although occasionally eyelid closure may be slightly weak. Voluntary facial movements are affected more than emotional ones, and movement in response to emotional stimuli may be normal (dissociated facial palsy). There is slight, if any, detectable involvement of the muscles innervated by the spinal accessory nerve. There may be slight weakness of the affected side of the tongue, but the throat and jaw muscles function normally. Deglutition, articulation, movements of the trunk, and other functions with bilateral supranuclear innervation are little affected. Voluntary, skilled, and learned actions are most impaired, and there is loss of the ability to carry out fine, independent, fractionated movements, especially with the distal portions of the extremities, with precision and delicacy. Gross movements and those that are habitual or have little voluntary control are relatively spared.
The extremity weakness reflects the preferential CST innervation of certain muscle groups and has a characteristic pattern: the pyramidal or corticospinal distribution. When weakness is mild, it may be detectable only in the corticospinal distribution. Distal muscles, especially hand muscles, receive more pyramidal innervation than proximal muscles and are particularly affected. In the upper extremity, weakness preferentially involves the wrist, finger and elbow extensors, supinators, and external rotators and abductors of the shoulder; there is relative sparing of the flexor, pronator, and internal rotation muscles. In the lower extremity, weakness is most marked in the foot and toe dorsiflexors, knee flexors, and flexors and internal rotators of the hip, with relative sparing of the extensors, external rotators, and plantar flexors. When weakness is severe, the strong, non-CST innervated muscles overcome the weak muscles, producing the characteristic posture of a spastic hemiplegia (Figure 25.6). The arm is held in adduction, with slight internal rotation at the shoulder, flexion and pronation at the elbow, and flexion of the wrist and fingers. Additional flexion may still be carried out, but there is marked weakness of extension. There is loss of isolated movements of the wrist and fingers; movements at the elbow and shoulder are less affected. In the lower extremity, there is weakness of flexion at the hip and knee; the hip is extended, adducted, and often externally rotated, and the knee is extended. There is weakness of the dorsiflexors and everters of the foot, often with a pes equinovarus deformity causing plantar flexion and inversion of the foot and toes.
FIGURE 25.6 Left hemiparesis of 15 years’ duration. The patient circumducts his left leg as he begins walking.
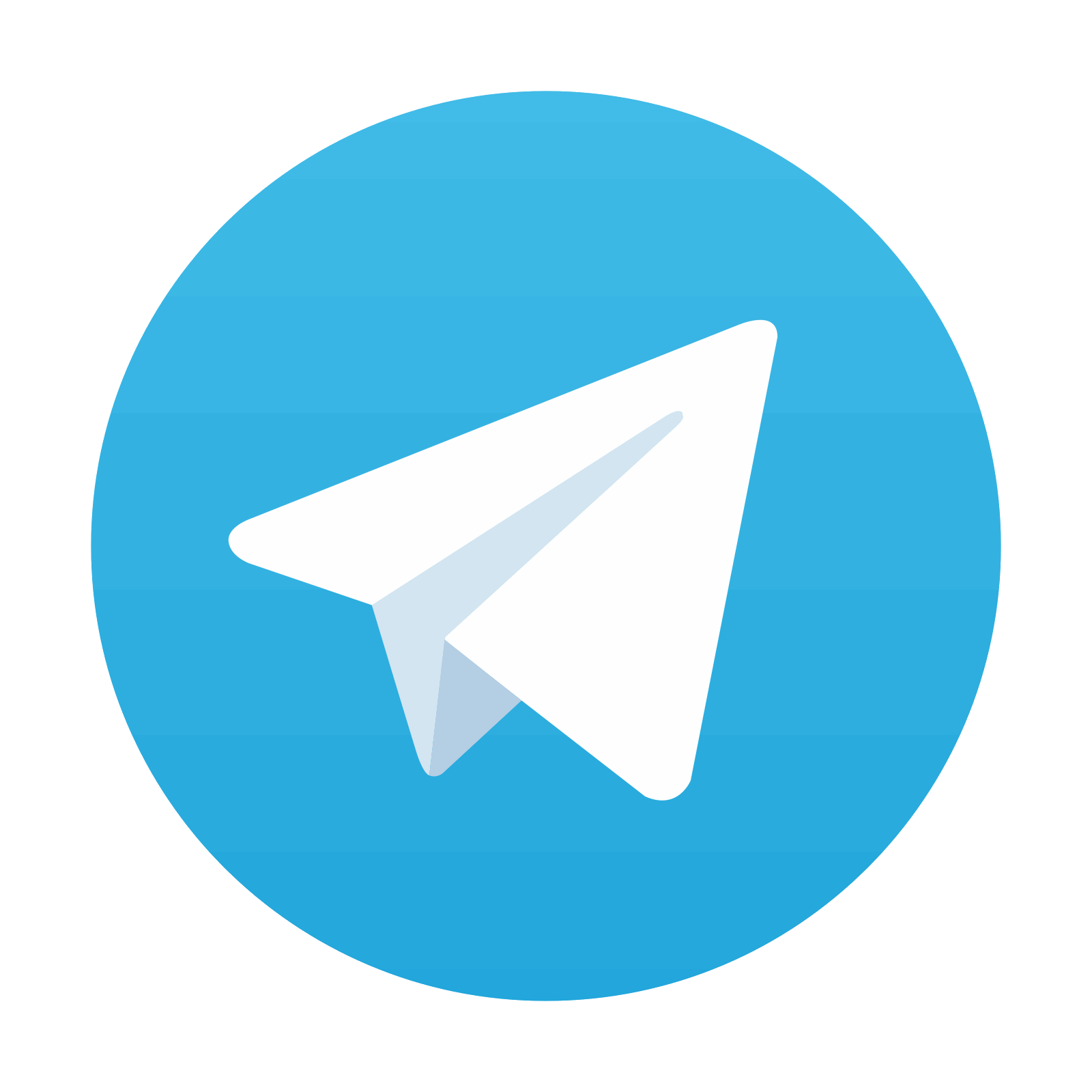