Fig. 1.1
The human cortical cytoarchtectonic regions (From Brodmann 1909). The olfactory region is not indicated
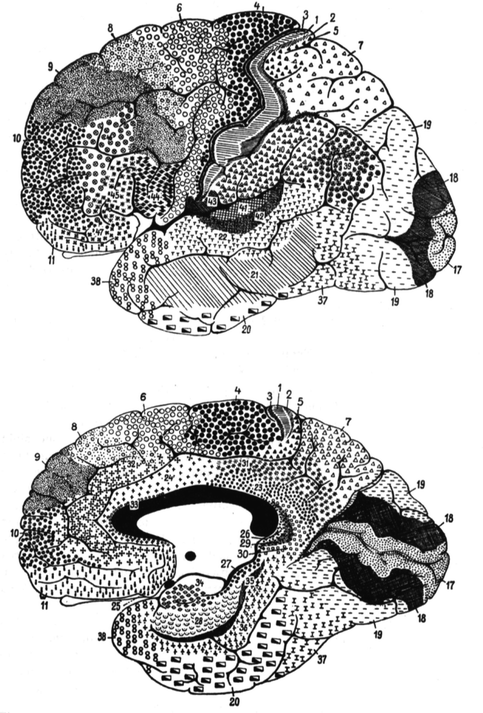
Fig. 1.2
Cortical areas of the lateral and medial aspects of the human cerebral hemispheres (From Brodmann 1909)
The postcentral region lies directly posterior to the central sulcus and consists mainly of the postcentral gyrus. It is subdivided into four areas: 1, 2, 3 and 43.
Area 1 – the intermediate postcentral area – a strip in the middle of the postcentral region between areas 2 and 3, along the apex of the postcentral gyrus and onto the medial surface, encroaching on the cortex of the central and postcentral sulci.
Area 2 – the caudal postcentral area – a narrow strip, mainly on the posterior aspect of the postcentral gyrus (the anterior bank of the postcentral sulcus). Its borders are not always sharp or constant. There are individual differences, as in the sulcal pattern.
Area 3 – the rostral postcentral area – covers the anterior of the postcentral gyrus (the posterior bank of the central sulcus). It has a variable width along the central sulcus. Its border with giant pyramidal area 4 anteriorly is sharp but in some brains its other borders can be less clear. At its medial and lateral ends, area 3 encroaches on the precentral gyrus, pushing area 4 anteriorly.
Area 43 – the subcentral area – is at the junction of the pre- and postcentral gyri at the inferior end of the central sulcus. Its anterior border is sharp and coincides with the anterior subcentral sulcus. It extends into the depths of the Sylvian fissure where it has a distinct boundary with the insular cortex.
The precentral region lies directly anterior to the central sulcus and is characterised by the lack of an inner granular layer. Dorsally, its anterior border crosses the precentral gyrus and encroaches on the superior and middle frontal gyri. Its anterior borders are clear but vary between individuals. Its posterior border is well demarcated from the postcentral region, and particularly from area 3, in the central sulcus. Areas 4 and 6 are characterised by the lack of an inner granular layer, and area 4 by the Betz giant cells.
“Area 4 – the giant pyramidal area – is one of the most strikingly differentiated and cytoarchitectonically delimitable structural regions of the whole human cerebral cortex.” It is wedge-shaped, along the central sulcus, narrowing from superior to inferior on the precentral gyrus and the adjacent part of the paracentral lobule. Superiorly it includes the whole width of the precentral gyrus but ventrally is restricted to the posterior half of this gyrus. Its borders are variable, especially in the paracentral lobule. There are local and individual differences in the number, size and distribution of giant pyramids: their size and number decrease from superior to inferior. “I must definitely classify as erroneous the idea, proposed by Elliot Smith, that the anterior subcentral sulcus is “a limiting furrow” for area 4”.
Area 6 – the agranular frontal area – is broad superiorly, narrowing inferiorly and laterally, and covers the whole vertical extent of the frontal lobe. Medially it occupies the anterior part of the paracentral lobule and the superior frontal gyrus. Laterally, it is on the superior and middle frontal gyri, and further inferiorly the whole precentral gyrus except where it is occupied by area 4.
The frontal region is the most extensive of the human cerebral cortex; it includes the frontal lobe anterior to the precentral region, around 20 % of the total cortical area. All its areas contain an inner granular layer. Posteriorly it has a clear boundary with the agranular frontal cortex, and anteriorly extends round the frontal pole. There are eight areas in the human frontal region. Areas 44, 45 and 47 on the inferior frontal gyrus have similarities, and can be termed subfrontal subregion. The differences between the others are sometimes difficult to determine.
Area 8 – the intermediate frontal area – is a strip, wide superiorly and narrowing laterally which, like the agranular frontal area (6), crosses from the callosomarginal sulcus on the medial surface onto the lateral surface.
Area 9 – the granular frontal area – is similar to, but more extensive than, area 8. On the lateral surface it stops ventrally near the inferior frontal sulcus.
Area 10 – the frontopolar area – covers the frontal pole. Inferomedially it is demarcated by the superior rostral sulcus.
Area 11 – the prefrontal area – forms the anteroventral part of the frontal lobe on its orbital and medial surfaces, thus including most of the straight gyrus, the rostral gyrus and the anterior end of the superior frontal gyrus.
Area 44 – the opercular area – is a well-differentiated area of the inferior frontal gyrus – Broca’s area. There is much variability of the sulci within it.
Area 45 – the triangular area – forms the triangular part of the inferior frontal gyrus. Its caudal border lies in the ascending ramus of the Sylvian fissure, its dorsal border in the inferior frontal sulcus and its rostral border near the radiate sulcus.
Area 47 – the orbital area – shares architectonic affinities with areas 44 and 45 and can be combined with them to form a subfrontal subregion.
Area 46 – the middle frontal area – is not clearly distinguishable from neighbouring areas. It includes about the middle third of the middle and the most anterior part of the inferior frontal gyri.
The parietal region coincides essentially with the parietal lobe, but inferiorly is difficult to differentiate from temporal and even occipital cortex; it is better distinguishable from the postcentral region at the postcentral sulcus.
Area 5 – the preparietal area – is delimited from neighbouring areas by the presence in layer V of large pyramidal cells almost the size of Betz giant cells, and a thick inner granular layer. Its thickness exceeds that of postcentral cortex. Its structure varies in individual cases, but its position is relatively constant. It begins in the caudal portion of the paracentral lobule, and narrows in the depths of the terminal branch of the callosomarginal sulcus on its rostral bank, extending to the lateral surface of the hemisphere.
Area 7 – the superior parietal area – extends from, medially the subparietal sulcus, laterally the intraparietal sulcus, posteriorly the parieto-occipital sulcus, and anteriorly the superior postcentral sulcus. One can distinguish a division into an anterior and posterior superior parietal area.
Area 40 – the supramarginal area – is ventral to the intraparietal sulcus around the posterior ramus of the Sylvian fissure, on the supramarginal gyrus. Anteriorly it borders the postcentral areas 2 and 43, separated from them by the inferior postcentral sulcus and the posterior subcentral sulcus. It has no sharp boundary with the temporal region (area 22).
Area 39 – the angular area – corresponds to the angular gyrus, widening around the posterior end of the superior temporal sulcus. Its boundaries with the occipital and temporal regions (areas 19 and 37) are ill-defined; its border with the parietal area is formed approximately by the intraparietal sulcus.
The occipital region includes the whole occipital lobe, and is divided into three structurally very different areas.
Area 17 – the striate area – is characterised by the calcarine type of cortex which is easily recognisable macroscopically. It is around the calcarine sulcus, and posteriorly extends a little round the occipital pole onto the lateral surface. Its individual borders are variable, with no relationships to “limiting sulci”. The cuneus and the lingual gyrus form part of the striate area to variable extents, depending on the folding of the calcarine sulcus: usually the striate area extends further ventrally from the calcarine sulcus than dorsally. The dorsal striate area retreats entirely from the surface into the depths of the sulcus.
Area 18 – the occipital area – is a ring-like area that surrounds the striate area, more extensively laterally.
Area 19 – the preoccipital area – further surrounds occipital area 18, again especially laterally. Its boundaries are not related to sulci.
The temporal region is well delimited and homogeneous, stretching from the posterior margin of the insula over the whole vertical extent of the temporal lobe to the rhinal sulcus or the temporal incisura. It contains several clearly different areas of which certain, such as the transverse gyri, form important subregions of great functional importance.
Area 36 – the ectorhinal area – lies lateral to the rhinal sulcus and represents the first area of the neopallium adjacent to the archipallium, to which area 35 belongs. It is heterotypical with relatively few cells but a massive development of those of layers V and VI. It is the rostral extension of the lingual gyrus.
Area 37 – occipitotemporal area. – is a wide, but poorly circumscribed, transition zone between the adjacent occipital and temporal regions, distinct from preoccipital area 19 and temporal area 20.
Area 38 – the temporopolar area – forms the tip of the temporal lobe, without any clear external delimitation; it fuses laterally with areas 20, 21 and 22, and medially with area 36, and is characterised by its great depth.
Area 20 – the inferior temporal area – corresponds to the inferior temporal gyrus and blends rostrally and caudally with areas 37 and 38 without sharp borders.
Area 21 – the middle temporal area – is situated in the middle temporal gyrus, although not precisely.
Area 22 – the superior temporal area – is well differentiated from areas 20 and 21. Together with the cortex of the transverse gyri of Heschl (1878) (areas 41 and 42) it forms a homogeneous structural region. It was known that the transverse temporal gyri of Heschl had a different structure from most of the temporal lobe. Campbell (1905) differentiated a field within these gyri, his “audito-sensory area”, contrasting it with the other temporal gyri, or “audito-psychic area”. Elliot Smith (1907), in agreement with this, wrote: “The two transverse gyri of Heschl represent a sharply-defined anatomical area of this cortex”, but gave no precise topographical description. The superior temporal area is superficial in the posterior two-thirds of the superior temporal gyrus, the deep part of which is partially occupied by areas 41, 42 and 52. Anteriorly it climbs onto the medial surface of the superior temporal gyrus; posteriorly it reaches the level of the vertical terminal branch of the Sylvian sulcus and blends with the supramarginal area.
Area 42 – the lateral (posterior) transverse temporal area – is medial to area 22, extending obliquely over the superior bank of the superior temporal gyrus, but partly on the free surface of the gyrus. It forms a well-demarcated crescent along the lateral edge of area 41.
Area 41 – the medial (anterior) transverse temporal area – corresponds to the anterior transverse gyrus. It is bordered medially by the parainsular area 52 from which it is sharply demarcated.
Area 52 – the parainsular area – forms a narrow band on the superior bank of the superior temporal gyrus along the posterior margin of the insula and represents a transitional area between the temporal cortex and the insula.
The insular region is distinguishable from surrounding regions by its easily recognisable laminar pattern, including the claustrum. It coincides approximately with the Sylvian fossa, but may encroach on the under surface of the frontal and temporal opercula. Brodmann divided the insula into two halves along the prolongation of the central sulcus, one posterior and granular, the other anterior and agranular, but without attributing numbers to them. Thus, like the central region, the insula is divisible according to the presence or absence of an inner granular layer.
The cingulate region: The crescent-shaped cingulate gyrus bordering the corpus callosum is divisible at the level of the central sulcus, like the insula, into a postcingulate and a precingulate subregion, the former with a distinct inner granular layer, while the latter (except area 32) does not have an inner granular layer. Thus the human cortical surface is divided at the level of the central sulcus into structurally different halves, an anterior agranular and a posterior granular, a trend that is also found in lower mammals.
Area 23 – the ventral posterior cingulate area – is in the ventral part of the posterior half of the cingulate gyrus and lies directly above the corpus callosum. It forms an arc around the splenium as far as the anterior bank of the parieto-occipital sulcus with which it gradually blends. Rostrally it fuses with the agranular precingulate subregion.
Area 31 – the dorsal posterior cingulate area – is in the dorsal portion of the posterior half of the cingulate gyrus and forms an arc around area 23 as far as the parieto-occipital sulcus. There is no clear outer border with area 23 or with the parietal cortex (area 7).
Area 24 – the ventral anterior cingulate area – in the ventral part of the anterior half of the cingulate gyrus next to the corpus callosum. Posteriorly it fuses gradually with a weakly granular transitional zone over the middle of the corpus callosum; anteriorly it extends as far as the rostrum. Its structure changes gradually from posterior to anterior.
Area 32 – the dorsal anterior cingulate area – forms a semicircle around the anterior end of the corpus callosum.
Area 33 – the pregenual area – is formed by a narrow strip of rudimentary cortex hidden in the callosal sulcus. Anteroinferiorly it stretches round the end of the rostrum of the corpus callosum, while posterosuperiorly it extends over the corpus callosum.
Area 25 – the subgenual area – is a small area of cortex inferior to the genu of the corpus callosum. Like the pregenual area it has a rudimentary (heterogenetic) laminar pattern.
The retrosplenial region consists of three crescent-shaped areas around the splenium of the corpus callosum. The retrosplenial cortex is partly heterogenetic.
Area 26 – the ectosplenial area – is apposed to the posterior end of the corpus callosum, hidden in the callosal sulcus. It has rudimentary lamination. Laterally it merges without a clear border with Area 29.
In Area 29 – the granular retrolimbic area – there is a unique development of the inner granular layer (IV) and degeneration of layers II and III. It is a narrow semicircular area around the ectosplenial area and lies to a great extent in the depths of the callosal sulcus.
Area 30 – the agranular retrolimbic area – covers the edge of the isthmus of the cingulate gyrus, but also extends a short distance over the anterior bank of the calcarine sulcus. It forms a sort of arc around the other retrosplenial areas. The inner granular layer is degenerated while layers III and V are relatively well developed.
The hippocampal region includes the (heterogenetic) cortex between the hippocampal and rhinal sulci.
Area 27 – the presubicular area – lies lateral to the subiculum, separated by a sharp border, as a long, narrow zone along the hippocampal sulcus from the uncus to the tail of the hippocampus just under the corpus callosum.
Area 28 – the entorhinal area – is heterogenetic and lies medial to the rhinal sulcus and covers most of the head of the parahippocampal gyrus.
Area 34 – the dorsal entorhinal area lies mainly medial to the inferior rhinencephalic sulcus.
Area 35 – the perirhinal area – is a narrow band along the rhinal sulcus. The inner granular layer is missing. It forms the border between the archipallium and the neopallium, and it is difficult to decide whether it should be attributed to the one or the other.
Area 48 – the retrosubicular area – is at the caudal end of the perirhinal area (35) and lateral to the presubicular area (27).
1.1.5 Brodmann’s Arealisation and Circuit Specialisation: The Pyramidal Cell
Pyramidal neurons are distinguished by their prominent apical dendrite and basal dendritic tree (Fig. 1.3). They comprise some 70–90 % of all neurons in cerebral neocortex (DeFelipe and Fariñas 1992). Pyramidal cells form rich plexuses of connections, often forming intrinsic lattices or patches, within cortical areas. They form nearly all cortico-cortical connections, both ipsi- and contralateral, as well as most subcortical connections. Pyramidal cells contain the excitatory neurotransmitter glutamate: their discharge directly facilitates cortical activity, rather than inhibiting it. Arguably, pyramidal cells are the principal neurons of the cerebral cortex, generating nearly all cortically initiated excitation. Different subtypes of pyramidal cells have been reported based on aspects of their morphology, for example, inverted pyramidal cells, those whose axons project into the white matter, and those whose axons are restricted to the grey matter (see Feldman 1984; Nieuwenhuys 1994; Elston and DeFelipe 2002; Valverde 2002 for reviews). In addition, pyramidal cells have been subdivided according to their neurochemical content and receptor subunit profiles (Gabernet et al. 1999; González-Albo et al. 2001; Hof et al. 2001). It has also been proposed that pyramidal cells are not genetically fated to have their characteristic morphology. According to this theory, all variants of spiny neurons, from the typical pyramidal cell to the typical spiny stellate cell, are derived from a common precursor (Valverde 1988). Here we focus on the “typical” pyramidal cell of unknown neurochemical content observed in mature primate cortex. As a prelude to studying specialisations in pyramidal cell structure that have occurred during the evolution of different primate species we first set out how pyramidal cell structure varies among Brodmann’s areas in a single species of macaque monkey (Macaca fasicularis), and outline how specialisation in cell structure may influence functional capabilities and, in turn, behavioural complexity.
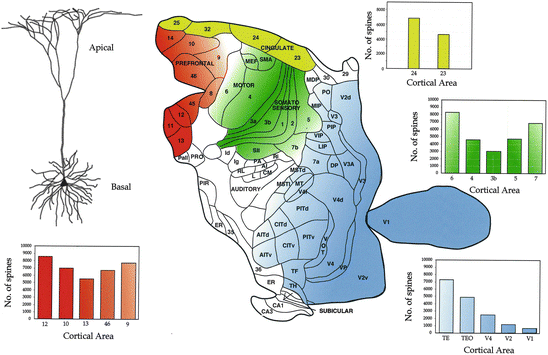
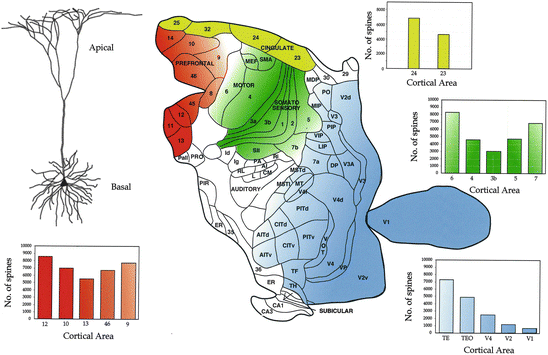
Fig. 1.3
Top left: a typical neocortical pyramidal cell. Centre: locations from which neurons were sampled in granular prefrontal (red), cingulate (yellow), sensorimotor (green) and visual (blue) cortex of the macaque monkey. Right: corresponding bar graphs of estimates of the total number of spines in the basal dendritic trees of layer III pyramidal cells in these areas. As each spine in mature cortex receives at least one excitatory input, differences in the estimates of the number of spines in different populations of cells may be taken as an approximation of numbers of excitatory inputs to these cells. Differences the complexity of the dendritic tree (size, number of branches) may also reflect differences in the integrative abilities of neurons (see Elston 2007 for a review). Note the trend towards more spinous cells with anterior progression from primary sensory areas such as Brodmann’s areas 17 (visual) and 3 (somatosensory) into adjacent functionally related cortical areas. In visual cortex, in particular, there is a striking systematic increase in the number of spines on pyramidal cells with progression through a hierarchical series of visual areas, including Brodmann’s area 18 (V2), into the temporal lobe. Note also the consistent trend for progressively more spinous cells with progression through somatosensory areas from the central sulcus to the angular gyrus (Data from Elston and Rosa 1998a; Elston et al. 1999a, 2001, 2005a, 2006, 2011; Elston 2000; Elston and Rockland 2002)
1.1.6 Visual Cortex
The areas of the cerebral cortex that contain neurons involved in some form of visual processing are perhaps the most thoroughly explored in the macaque brain. Prior to the 1970s, most studies were restricted to Brodmann’s area 17 (the striate, or primary (V1), visual cortex). Since then there has been an explosion in the number of studies in, and our understanding of, extrastriate visual cortex (Zeki 1969, 1978a) see (Kaas 1995; Rosa 1997; Kaas and Lyon 2001; Kaas and Preuss 2003; Zeki 2003; Rosa and Manger 2005) for reviews. Application of techniques such as electrophysiological mapping and imaging, and the development of specialised tracers, have revealed that visual processing is much more complex than previously thought, involving up to half the cortex and as many as 30 different areas (Fig. 1.3). Various theories have been presented for the existence of so many visual cortical areas, and how visual stimuli are processed by neurons in these areas (see Kaas 1987; Weller 1988; Felleman and Van Essen 1991; Rosa 1997; Kaas 2000 for reviews). In addition, many theories have been proposed regarding the recruitment and interaction of neurons in these different cortical areas during particular visual tasks. Two of the most popular models include the quasi-hierarchical model and the distributed processing model see (Mountcastle 1995) for a review. In the quasi-hierarchical model, visual inputs to cortex are processed through a series of cortical areas. These areas are not necessarily organised into a strict hierarchy, but there is some form of serial processing through select visual areas. In the distributed processing model, visual processing occurs in multiple cortical areas, but not necessarily in any form of hierarchy. That is not to say however, that the two theories are mutually exclusive. Mountcastle (1995), for example, highlighted how hierarchies may exist within a distributed system.
New methods of quantification (Elston and Rosa 1997; Elston 2001) have revealed marked, systematic differences in pyramidal cell structure (and cortical circuitry) in these different visual areas. Briefly, there is a trend for increasingly more complex pyramidal cells with progression from V1 to the second visual area (V2) and parietal visual areas (the lateral intraparietal area, LIP, and cytoarchitectonic area 7a), and temporal areas (V4, the middle temporal area, MT, cytoarchitectonic areas TEO and TE, and the superior temporal polysensory area, STP) (Elston and Rosa 1997, 2000; Elston et al. 1999a). The increase in the size of the dendritic tree, coupled with a concomitant increase in the number of dendritic branches and spine density, results in a progressive doubling in our estimates of the total number of spines in the basal dendritic trees of pyramidal cells through V1, V2, V4, TEO and TE. The functional implications of these specialisations in pyramidal cell structure in functionally related cortical areas are discussed in detail in the works of Elston (2002, 2007), Jacobs and Scheibel (2002), Spruston (2008) and DeFelipe (2011).
1.1.7 Somatosensory and Motor Cortex
Based on patterns of connectivity, neuronal response properties and, more recently, imaging studies, several theories have been put forward regarding normal function across, and cooperation between, Brodmann’s sensorimotor areas (Mishkin 1979; Pons et al. 1987, 1992; Passingham 1997; Geyer et al. 2000). By injecting large numbers of pyramidal cells in some of these different cortical areas it has been possible to demonstrate marked and systematic differences in their size, branching complexity and spine density. More specifically, two trends of increasing morphological complexity have been revealed with progression from the central sulcus to adjacent cortical areas. There is a systematic increase in the size of pyramidal dendritic trees, their branching complexity, and spine density within their basal dendritic trees, with caudal progression from the primary somatosensory area on the posterior wall of the central sulcus (Brodmann’s area 3; 3b) to the rostral bank of the intraparietal sulcus (Brodmann’s area 5) and the exposed rostral portion of the inferior parietal lobule (Brodmann’s area 7; 7b). There is also an increase in the size of the dendritic trees of pyramidal cells, their branching complexity, and the total number of spines within their basal dendritic trees, with rostral progression from the primary motor area on the anterior wall of the central sulcus (Brodmann’s area 4) to the exposed lateral portion of the precentral gyrus (Brodmann’s area 6 or premotor cortex) (Elston and Rockland 2002). These differences in size, branching complexity and spine density result in appreciable differences in our estimates of the total number of spines in the basal dendritic tree of the average cell in each cortical area (Fig. 1.3).
1.1.8 Cingulate Cortex
A study of the literature reveals little agreement regarding the functions performed in cingulate cortex. Some have attributed higher cognitive and emotional functions to the anterior cingulate cortex and vegetative functions to posterior cingulate cortex (Allman et al. 2001) whereas others have claimed the reverse (eg Baleydier and Mauguiere 1980). In a series of studies in which cortical activity was recorded in awake behaving monkeys by functional magnetic resonance imaging (fMRI), Dreher and colleagues revealed that neurons in anterior cingulate cortex, unlike those in posterior cingulate cortex, are often co-activated with granular prefrontal cortex (gPFC) during cognitive tasks (Dreher and Berman 2002; Dreher and Grafman 2003). Various other studies have also demonstrated “executive” or cognitive function in the anterior cingulate, including error detection and reward-based decision-making (Gemba et al. 1986; Carter et al. 1998; Bush et al. 2002; Shidara and Richmond 2002; Hadland et al. 2003).
While it is well known that these two regions of cingulate cortex can be distinguished by their cytoarchitecture, particularly the granular layer, relatively little is known of possible differences in their microcircuitry. In order to investigate this, layer III pyramidal cells were injected in the posterior cingulate gyrus (Brodmann’s area 23) and their structure compared with that of cells injected in the anterior cingulate gyrus (Brodmann’s area 24) (Elston et al. 2005a). These investigations revealed that pyramidal cells in area 24 were considerably larger, more branched and more spiny than those in area 23. Estimates of the total number of spines in the basal dendritic tree of the average cell reveal a two-fold difference between cells in areas 23 and 24 (Fig. 1.3). Moreover, pyramidal cell structure in area 24 more closely approximates that of cells in the gPFC, than does that of cells in area 23 (see below).
1.1.9 Prefrontal Cortex
Exactly what constitutes prefrontal cortex (PFC) has been interpreted in many ways. Some classify it as cortex that receives projections from the medial dorsal nucleus of the thalamus whereas others distinguish the PFC by cytoarchitecture (see Fuster 1997 for a review). Here, as intended by Brodmann (1913), the term is used to only include granular cortex anterior to the central sulcus (see Elston and Garey 2004). To avoid confusion here this region is referred to as the gPFC. Brodmann’s original maps of the gPFC have been further refined by others by cytoarchitecture and patterns of corticocortical connectivity (Vogt and Vogt 1919; Walker 1940; Barbas and Pandya 1989; Petrides 1991; Preuss and Goldman-Rakic 1991a, b, 1991c; Petrides 1998; Pandya and Yeterian 2000; Petrides and Pandya 2001). Broadly speaking, these different areas have been grouped into the lateral, medial and orbital regions.
Prefrontal cortex has been the focus of intensive investigation in recent times because of its involvement in executive functions such as conceptual thinking, prioritising and planning (see Goldman-Rakic 1996; Fuster 1997; Barbas 2000; Petrides 2000; Miller and Cohen 2001 for reviews). Our understanding of the functions performed by neurons in the different regions within gPFC, and how other cortical regions participate in specific tasks, is growing rapidly with the advent of new methodologies (see Quintana and Fuster 1999; Passingham et al. 2000; Rolls 2000; Fuster 2001; Funahashi and Takeda 2002 for reviews). Orbital and medial gPFC are now believed to be involved in emotional behaviour and the processing of taste, reward, memory, affect and motivation. The lateral gPFC provides cognitive support to the temporal organisation of behaviour, speech and reasoning and is involved in executive control of voluntary motor movements. Caudo-rostral gradients characterised by different patterns of connectivity and functions have also been reported within each of these gross subdivisions (Goldman-Rakic 1987; Petrides 1987, 1991; Barbas 1992; Wilson et al. 1993; Barbas et al. 1999; Pandya and Yeterian 2000). What is clear from these studies is that because of the complexity of functions performed by neurons in gPFC, and the difficulty in quantifying neuronal responses to specific executive tasks, our understanding of prefrontal function is likely to lag behind that of sensory cortex for some time to come.
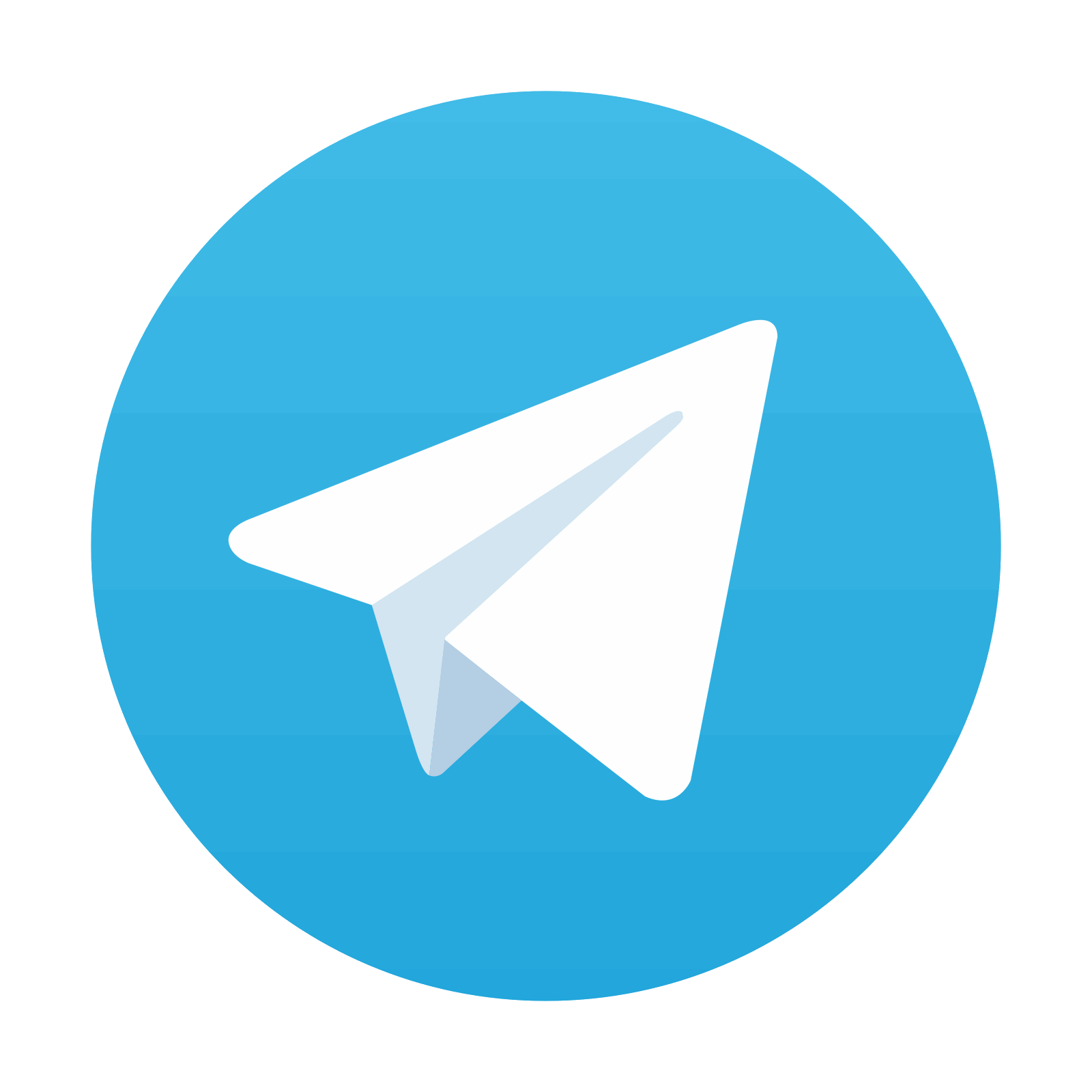
Stay updated, free articles. Join our Telegram channel

Full access? Get Clinical Tree
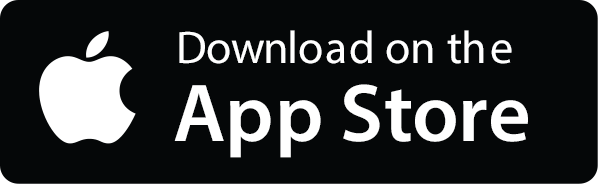
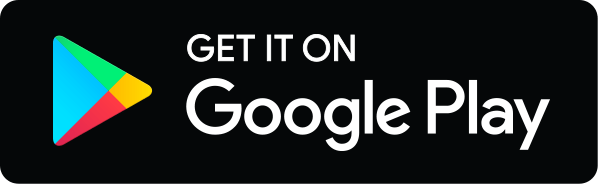