The Development and Neuropsychology of Visual Impairment
Rick O. Gilmore
Joel M. Weinstein
Rebecca Von Der Heide
Introduction
At least half of the human brain responds to visual stimulation, and so the study of vision has dominated the field of behavioral neuroscience since the 1950s. Moreover, whereas many scientists studying vision do not focus on how visual perception changes over development, most clinicians do share these concerns, in large part because the most common diseases of vision tend to occur early (e.g., strabismus) or late (e.g., glaucoma) in life. In this chapter, we summarize what is known about the normal development of the visual system and its disorders, with an emphasis on infancy and childhood. The reader interested in more-thorough summaries of the issues we discuss should consult volumes by Daw (1), Simons (2), Atkinson (3), or Rakic (4).
The chapter is divided into three sections, including this introduction. Section 1 describes the visual system and its normal developmental patterns; Section 2 describes the most common diseases and disorders of visual development; Section 3 provides a brief conclusion.
Normal Development
In comparison to the auditory or somatosensory systems, the visual system is relatively immature at birth. Newborns do not see details well, are insensitive to low levels of contrast, and are sensitive to color and motion over much narrower ranges than are adults. Vision develops rapidly over the first 6 months after birth, followed by a somewhat slower rate of change into the preschool years. It was once thought that visual capacities were largely mature by the time children entered school at 5 or 6 years of age, but recent tests with very sensitive measures have shown that changes in basic visual processing continue into adolescence. In this section, we briefly review some of the essential facts concerning normal development of the visual system.
ANATOMY AND PHYSIOLOGY OF THE DEVELOPING VISUAL SYSTEM
The eye
The formation of a focused, undistorted visual image on the retinal surface is the first step in the process of visual perception. The eye consists of a family of structures that contribute
to the formation of this image: the cornea, lens, and iris/pupillary system (Fig. 16.1). The retina itself is functionally segregated and composed of two broad classes of photoreceptors: rods and cones. Rods subserve achromatic vision at low light levels and are concentrated in the peripheral portions of the retina. Cones subserve color or chromatic perception and are the only photoreceptors in the central portion of the fovea, the 1- to 2-degree region of the visual field where daytime visual acuity is best. Both classes of photoreceptors synapse on retinal bipolar cells, which signal information from photoreceptors to ganglion cells. Approximately 1 million ganglion cells in each eye receive input from this retinal network and send action potentials along the optic nerve and tract to the brain.
to the formation of this image: the cornea, lens, and iris/pupillary system (Fig. 16.1). The retina itself is functionally segregated and composed of two broad classes of photoreceptors: rods and cones. Rods subserve achromatic vision at low light levels and are concentrated in the peripheral portions of the retina. Cones subserve color or chromatic perception and are the only photoreceptors in the central portion of the fovea, the 1- to 2-degree region of the visual field where daytime visual acuity is best. Both classes of photoreceptors synapse on retinal bipolar cells, which signal information from photoreceptors to ganglion cells. Approximately 1 million ganglion cells in each eye receive input from this retinal network and send action potentials along the optic nerve and tract to the brain.
The eye and retina undergo prolonged patterns of pre- and postnatal development. Retinal cells appear to be generated during an extended period in embryonic development that begins in the first half of gestation and continues up to the time of birth, even though cell formation has long ended in the downstream structures, such as visual cortex. Roughly 60% of the initially produced retinal ganglion cells are lost by the time of birth because of programmed cell death.
Many features of the retinal architecture appear to develop early are largely independent of functional activity (but see 9). For example, the distribution of rods and cones in the fetal retina appears roughly adult-like before the onset of functional synaptic contacts with horizontal or bipolar cells. Conversely, the newborn retina does not have the dense packing of photoreceptors in the central foveal region characteristic of adults, and the outer, light sensitive segments of photoreceptors do not reach their mature length for several months after birth. Large-scale photoreceptor migration toward the fovea and increases in outer segment length occur during the first year of life (5), but other subtle morphological changes occur up to the age of 4 years.
Furthermore, the eye itself undergoes changes in size and shape. Although proportionately large at birth relative to head size, considerable growth occurs in the size of the globe. The distance from the cornea to the retina increases by about 25% by 1 year of age and increases another 25% by adolescence (6).
The visual brain
Despite the complexity of its circuitry, the retina is only the first stage in visual processing in the human. We now turn to a discussion of the subcortical and cortical structures that support visual functions (Fig. 16.2).
Subcortical structures
The critical subcortical components of the visual system consist of the optic nerve and tract, and its associated structures, the superior colliculus (SC) and associated structures, the hypothalamus, and the lateral geniculate nucleus (LGN) of the thalamus. Projections from the retina to the SC mature early and may form the main basis of neonatal visual perception and visually guided action, according to one model of visual development (3,7,8). Conversely, projections from the retina to the hypothalamus may not make functional connections until 3 to 4 months after birth. This could account, at least in part, for the difficulties parents of young infants have in helping their children keep a consistent schedule of nighttime sleep because the hypothalamus helps maintain the body’s circadian rhythm by means of projections to the pineal gland, among other structures.
![]() ▪ FIGURE 16.2 Projection patterns from the retinae, through the optic chiasm, to the lateral geniculate nucleus (LGN), via the optic radiations to visual cortex in the occipital lobe. (Reprinted with permission from Weber J, Kelley J. Health assessment in nursing, 2nd ed. Philadelphia: Lippincott Williams & Wilkins, 2003.) |
Cortical structures
Visual information activates as much as half of the primate cerebral cortex, but the most crucial structures are located in the occipital lobe, the site of primary visual cortex (V1) and associated regions V2 to V4, with additional higher-order systems located in the parietal, temporal, and frontal lobes (11,12). The best available evidence suggests that visual cortex undergoes substantial postnatal development in the human (10,13, 14, 15, 16).
The development of visual behavior
Much of what is known about the development of the human visual system early in life comes from systematic studies of visual behavior. In this section, we briefly discuss changes in the size of the visual field, increases in visual acuity, and changes in visual behaviors thought to be specifically related to the dorsal and ventral visual-processing streams.
Field size
Neonates have binocular visual fields that are only 15% to 25% of the 160- to 170-degree values typical of the average human adult (17), and field size does not reach adult values until 12 to 24 months. Monocular field size has a somewhat more rapid developmental time course, approaching adult levels by approximately four months (18). Moreover, field size for static targets develops more rapidly in the temporal visual field than in the nasal field. Field sizes to moving targets grow more slowly; adult levels are not reached until approximately the end of the first year of life (19). Differences between the nasal and temporal hemifields have been attributed both to peripheral factors, such as differential timing in retinal maturation, and to central factors, such as the anatomy of crossed versus uncrossed projections.
Visual acuity
The ability to resolve detailed features of the visual image is critical for the recognition of form. In the adult, visual acuity is close to the theoretic limit imposed by the optics of the eye, sampling density of photoreceptors in the retina, and processing in the cortex. Visual acuity in infants and young children is less than that in adults, and is even below the theoretic limit, but both grating and vernier acuity develop rapidly in the first 6 to 8 months of life (20, 21, 22).
Binocular vision
Humans depend on mechanisms that compare the disparity or offset of images between the two eyes to estimate distance from the observer. For binocular visual mechanisms to work properly, both eyes must align on a single point in visual space, and circuits in V1 and higher-order systems must be able to compute retinal disparity. These systems are immature in the neonate but develop rapidly. Infants show visual evoked potentials (VEPs or brain electrical patterns evoked by visual stimulation) activated by binocular correlation between 8 and 20 weeks (23) and a rather abrupt onset of disparity sensitivity between 12 and 20 weeks (24). Thereafter, the minimal retinal disparity that can be detected appears to increase rapidly. Some authors have speculated that the formation of ocular dominance columns allows stereoacuity (25).
Motion perception
Motion perception is critical both for perceiving objects moving relative to the environment and for determining where and how fast the observer is moving (26,27). Primitive
capacities to detect and respond to visual motion have been observed in newborns. By 7 to 8 weeks, direction-selective VEPs are observed (28), and behavioral measures show gradual development in direction selectivity up to 18 to 20 weeks (27). Development in motion sensitivity outside the infancy period is poorly understood, but recent evidence suggests that it has a prolonged developmental time course, continuing well into the early school-age years.
capacities to detect and respond to visual motion have been observed in newborns. By 7 to 8 weeks, direction-selective VEPs are observed (28), and behavioral measures show gradual development in direction selectivity up to 18 to 20 weeks (27). Development in motion sensitivity outside the infancy period is poorly understood, but recent evidence suggests that it has a prolonged developmental time course, continuing well into the early school-age years.
Color, shape, and form
In general, young infants do not discriminate colors well, and evidence indicates that sensitivity to shorter (i.e., blue) wavelengths may be especially impaired. Sensitivity to red-green differences appears to emerge in the second month after birth, and discrimination of blue-yellow differences sometime later (29).
However, most vision scientists agree that the detection of an object’s overall shape is an important first step in the complex, and poorly understood, process of form perception. Selectivity for the orientation of static or moving-line segments, a characteristic of neurons in V1, undoubtedly plays an important role. Newborns can discriminate large changes in orientation (30), and sensitivity to smaller changes in orientation appears to develop gradually over the first several months after birth (3). Thus although considerable postnatal development occurs, the neonatal visual system is well disposed to extracting crude information about shape and form.
Abnormal Development
We now turn to a discussion about disorders and diseases of the eye and visual system. When dealing with visual disorders in children, it is important to recognize the plasticity of the developing visual system. Children who do not receive appropriate visual stimulation early in life will not develop the neural circuitry required for normal vision and will have some form of visual developmental delay. These defects may or may not be remediable, depending on the child’s chronological age, the intensity of the visual rehabilitation program, and unknown factors that govern the “critical period” for the development of neurovisual circuitry. A large body of clinical and experimental evidence suggests that different critical periods exist for the development of various components of visual perception (1). As discussed earlier in this chapter, motion detection, two-dimensional binocular vision, and three-dimensional depth perception (stereopsis) all begin and end their maturation at different ages. Although the clinical and experimental literature refer to “critical periods” for the development of these cognitive faculties, in practice, neither the duration of the critical period nor the extent of plasticity has been precisely defined for any of these functions. Undoubtedly both the duration and extent of the critical periods are dependent on both genetic factors and prior environmental conditioning.
Because of the neural plasticity inherent in the infant visual system, the distinction between “congenital” and “acquired” visual defects may be less important in the first 2 years of life than the nature of the visual impediment. For example, severe unilateral congenital cataracts (an opacity in one or both ocular lenses), which are not removed in the first 2 months of life, are likely to result in severe and irreversible visual loss, despite heroic effects at rehabilitation at a later age. Infantile esotropia (eye misalignment), conversely, is compatible with excellent vision and some degree of stereopsis after surgical alignment in the first year or even 18 months of life. Nevertheless, it is safe to state that, for most pediatric visual disorders, the earlier the treatment, the better the outcome.
DISORDERS OF THE EYE
Congenital and developmental cataracts
Cataracts may occur as an isolated disorder or as part of a multisystem genetic or metabolic disease. The association between cataract and various systemic diseases has been reviewed elsewhere (32). This discussion is limited to the diagnosis and visual rehabilitation of children with cataracts, irrespective of the etiology of the cataracts.
Isolated cataracts in childhood are usually genetically determined and are most commonly inherited in an autosomal-dominant pattern. As with other autosomal-dominant conditions, penetrance may be incomplete (i.e., not all carriers may show the phenotype and, when present, its severity may vary). Cataracts occurring in documented autosomal-dominant pedigrees are almost always bilateral. However, sporadic unilateral cataracts occur quite commonly.
Early diagnosis of congenital and juvenile cataracts is imperative to avoid severe amblyopia (impaired or dim vision without obvious defect or change in the eye, see later). Surgery after the age of 3 months rarely results in vision better than 20/200. Early diagnosis and treatment, conversely, is compatible with an excellent visual outcome if no other ocular anomalies are present, such as microphthalmos, corneal opacity, or retinal or optic nerve dysplasia. The visual outcome in children with cataracts has improved greatly over the past 15 to 20 years because of advances in surgical technique and technology, better contact lenses, and, in selected cases, the use of intraocular lenses (33). Nevertheless, parents of children with congenital or developmental cataracts must understand that surgery is only the first step in a long process that will require a major expenditure of time and energy on the part of parents and patients. Amblyopia is common, and intensive patching is the rule rather than the exception. Eye examinations under anesthesia may be needed several times annually to check refraction, rule out retinal abnormalities, and monitor for glaucoma, which occurs in about 30% of children after cataract surgery.
Although in most children with congenital cataracts, useful vision develops, acuity is rarely at the norm for the age, and a few patients, especially those who have delayed surgery, never develop good vision. The psychosocial impact of poor vision in infancy is protean. Many aspects of development—gross and fine motor, cognitive, psychosocial, and interpersonal skills—depend on vision. An approach to children with severe visual disability is discussed in greater detail later. In addition to the psychosocial impact of visual impairment, quality of life in children with congenital cataracts is affected by the necessity for recurrent surgeries, amblyopia therapy, and frequent medical visits. Quality-of-life scores, according to one report, may be comparable to those reported in children with severe systemic diseases, such as cancer (34).
Congenital/juvenile glaucoma
Glaucoma, an excessive intraocular pressure causing retinal degeneration, may occur in childhood as an isolated anomaly (primary congenital or juvenile glaucoma), in association with systemic abnormalities, such as Sturge-Weber and Lowe syndromes or the mucopolysaccharidoses, or may be associated with other ocular abnormalities, such as aniridia (congenital underdevelopment of the iris). Ho and Walton (35) reviewed the systemic associations. Most cases of isolated primary congenital and juvenile glaucoma are inherited, and several gene loci have been isolated. However, genotype-phenotype correlation has not been close (i.e., the same gene defect may result in widely disparate severity and manifestation within the same family) (35).
The typical patient with primary congenital glaucoma (PCG) is first seen sometime during the first year of life with symptoms related to corneal edema, including tearing, photophobia, and discomfort, often manifested as eye rubbing. The cornea is usually
cloudy and enlarged, giving rise to the term “buphthalmus” (ox eye). The most severe form of primary congenital glaucoma is seen at birth with diffuse corneal opacification and enlargement. If corneal enlargement is not appreciated and the corneal clouding is mild, the child may be incorrectly diagnosed with nasolacrimal duct obstruction, and definitive surgery may be delayed.
cloudy and enlarged, giving rise to the term “buphthalmus” (ox eye). The most severe form of primary congenital glaucoma is seen at birth with diffuse corneal opacification and enlargement. If corneal enlargement is not appreciated and the corneal clouding is mild, the child may be incorrectly diagnosed with nasolacrimal duct obstruction, and definitive surgery may be delayed.
Surgery is the primary treatment of choice for congenital glaucoma, though supplemental medication may be useful (35,36). After surgery, children with PCG require repeated eye examinations under anesthesia to check intraocular pressure and monitor for optic disc damage. Myopia is frequent due to elongation of the globe. Asymmetric myopia may lead to amblyopia and the need for patching therapy. With early diagnosis and intervention, visual prognosis is very good. However, at least 20% of children will require more than one surgical procedure (36).
The visual outcome in children with congenital glaucoma is variable. Multiple surgeries are not infrequent, and prolonged intensive therapy for amblyopia may be necessary. In children with a poor visual outcome, cognitive, psychosocial, and motor development may all be delayed because of visual impairment. Children with severe visual impairment require an extensive support network, including psychological support for both child and parents. These considerations are discussed at greater length later in the chapter. In addition, children with recurrent surgeries, hospitalizations, and visits to doctors may have the same quality-of-life issues that have been well documented in children with rheumatologic disease and cancer (33). Psychiatric support is essential in helping these children to overcome the handicaps of low vision and of multiple medical and surgical procedures.
Strabismus and amblyopia
Strabismus, a misalignment of the eyes, and amblyopia, an acquired loss of monocular function, are major public health problems, occurring in 2% to 5% of children even in developed countries (37). These disorders begin in infancy or early childhood and have a profound effect on subsequent visual development, including both monocular and binocular vision. In addition, the psychosocial stigma of “crossed eyes” and of wearing an occlusive eye patch may damage self-esteem and other aspects of emotional development. Early recognition of strabismus and amblyopia are important for minimizing their visual and psychosocial consequences. The alternative to early diagnosis and treatment is frequently a scenario involving prolonged patching, multiple surgeries, amblyopia, impaired binocular vision (depth perception), and a life-long suboptimal cosmetic outcome. Childhood strabismus may cause exotropia (one eye turning outward toward the ear), esotropia (one eye turning inward toward the nose), or, more rarely, hypertropia (one eye turning upward).
A number of studies demonstrated the negative psychosocial impact of strabismus in both adults and children (38, 39, 40, 41, 42, 43, 44). A negative attitude toward strabismus seems to emerge in nonstrabismic children at approximately 5 to 6 years of age (40). Therefore both children and adults with strabismus may have poor self-esteem, a higher incidence of dysfunctional interpersonal relationships, and poorer prospects for employment (42). It appears that negative attitudes toward strabismus are directed more frequently toward girls and women than toward boys and men. In addition, mothers of children with strabismus express more dissatisfaction with their maternal role and may become depressed and dysfunctional (43). The psychiatrist can actively assist parents and children in overcoming these obstacles.
Mounting evidence indicates that surgery for strabismus can result in significant improvement in both psychological and visual function (44). Parents may be unaware of this benefit and may have been told by friends, family, or the primary physician that surgery would have none. Although it is not the psychiatrist’s role to recommend strabismus surgery, he or she can help the parent and child explore all issues that may affect the child’s self-esteem.
Infantile esotropia
Formerly called “congenital esotropia,” infantile esotropia is first seen with one eye that deviates inwardly from the other. It has its onset during the first 6 months of life. In one half to two thirds of these children, the esotropia is alternating. For example, the child alternates from minute to minute between fixation with the right eye (left esotropia) and fixation with the left eye (right esotropia). Although many normal infants have a small-angle intermittent deviation in the first 3 months of life, a constant or large-angle deviation beyond the fourth month of life will almost always persist indefinitely in the absence of treatment (45).
The majority of children with infantile esotropia are otherwise developmentally normal. Groups at higher risk for infantile esotropia include children with Down syndrome (trisomy 21), intraventricular hemorrhage, hydrocephalus, cerebral palsy, or prematurity of less than 32 weeks of gestation.
As discussed earlier, the cortical circuits for binocular vision develop rapidly between the ages of 3 and 6 months. Children with infantile esotropia will not develop any form of binocular vision without intervention to realign the visual axes (46). These children function in an essentially monocular mode. Information from the fixating eye (the eye that is targeted on the object of interest) is processed normally, whereas information from the deviated eye, which presents conflicting visual information, is largely suppressed and ignored by the visual cortex. Precise depth perception, which requires binocular information, is not possible if either eye is misaligned. In addition, if the child does not alternate—if he has a strong preference for fixation with one eye and never, or rarely, uses the other eye—the visual cortex will not develop the circuits required to decode the incoming message from the chronically deviated eye. The result will be poor vision in that eye (i.e., amblyopia).
Several retrospective longitudinal studies have demonstrated that the long-term outcome in children with infantile esotropia strongly correlates with duration of misalignment (47,48). The eyes of children who undergo surgical realignment after less than 3 months of constant esotropia have a lower incidence of amblyopia, better binocular vision, and better long-term stability of alignment; fewer require repeated surgery for recurrent strabismus. A consensus exists among pediatric ophthalmologists that children with infantile esotropia should have surgery before the age of 9 months (49).
Accommodative esotropia
Accommodative esotropia usually is first seen between the ages of 18 months and 5 years and is associated with moderate to severe farsightedness (hyperopia) (50). This type of esotropia is caused by excessive use of the “near reflex,” which consists of the triad of accommodation, convergence, and miosis. In children who are excessively farsighted (hyperopic), parallel light rays from infinity do not converge on the retina, and additional focusing activity (accommodation) is needed (Fig. 16.3). In turn, accommodation is accomplished
by invoking the near reflex, which results in inappropriate convergence. The child then has clear vision, because of accommodation, but misaligned visual axes because of inappropriate convergence. In children, this misalignment leads to suppression of information from the deviated eye and lack of binocular vision.
by invoking the near reflex, which results in inappropriate convergence. The child then has clear vision, because of accommodation, but misaligned visual axes because of inappropriate convergence. In children, this misalignment leads to suppression of information from the deviated eye and lack of binocular vision.
If the child does not alternate fixation—if the same eye always turns inward and the deviation is chronic—amblyopia will result (see later). In this situation, circuits for monocular vision will fail to develop or, if present, will be actively disassembled.
The treatment for accommodative esotropia is glasses, which provide focusing power and substitute for the near reflex with its inappropriate convergence (Fig. 16.4). Contact lenses may be used in lieu of glasses in older children and teenagers. Like infantile
esotropia, accommodative esotropia requires early intervention. Duration of misalignment is negatively correlated with quality of both monocular and binocular vision (stereopsis), as well as with risk for future deterioration of alignment, which may require surgery (50).
esotropia, accommodative esotropia requires early intervention. Duration of misalignment is negatively correlated with quality of both monocular and binocular vision (stereopsis), as well as with risk for future deterioration of alignment, which may require surgery (50).
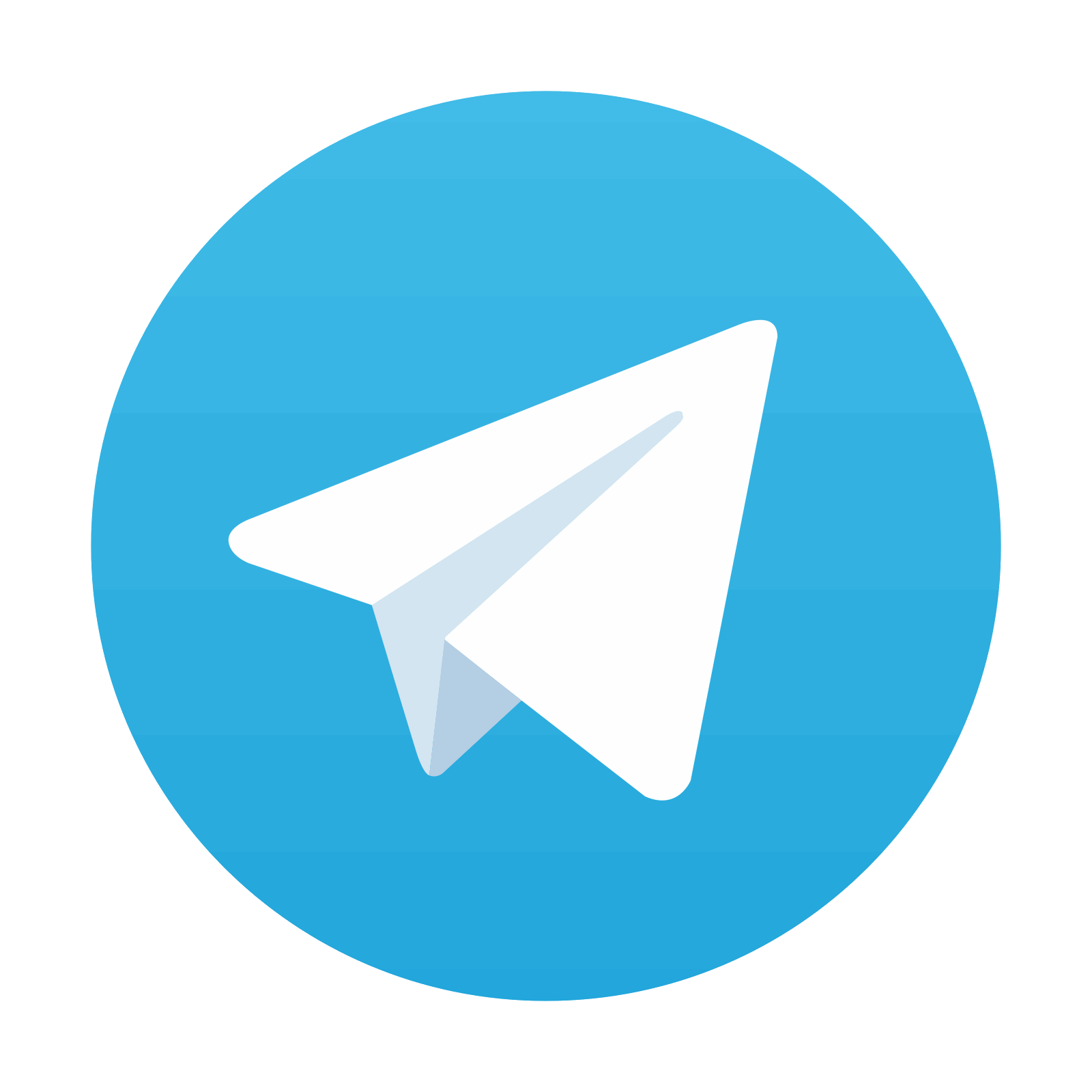
Stay updated, free articles. Join our Telegram channel

Full access? Get Clinical Tree
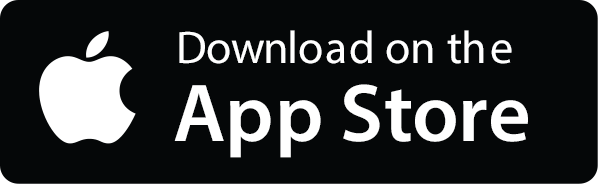
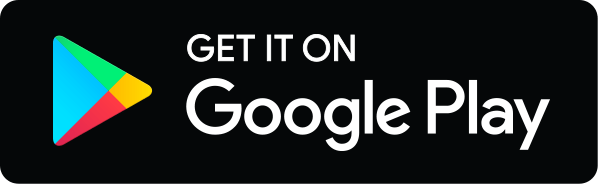
