© Springer International Publishing Switzerland 2017
Gin S. Malhi, Marc Masson and Frank Bellivier (eds.)The Science and Practice of Lithium Therapy10.1007/978-3-319-45923-3_44. The Effect of Lithium on Gene Expression Modulation
(1)
Department of Biomedical Sciences, University of Cagliari, Cagliari, Italy
(2)
Department of Psychiatry, Dalhousie University, Halifax, NS, Canada
Abstract
Lithium has been shown to influence the expression of hundreds of genes. Through this regulation, lithium interferes with a large number of cellular functions, including inositol metabolism, circadian rhythms, apoptosis and those neuroprotective pathways that are thought to play a role in the pathophysiology of bipolar disorder. The mechanisms through which lithium regulates gene expression are still not completely understood. However, converging data suggest that transcription factors and microRNAs, as well as epigenetic factors, may constitute key targets of lithium. In this chapter, we present and discuss the most compelling findings on lithium’s effects on gene expression modulation, emphasizing those studies carried out in patient-derived cell lines, as these have the potential to highlight genes that may constitute biomarkers of the clinical efficacy of lithium.
Keywords
Gene transcriptionmicroRNAEpigeneticsNeuroprotectionApoptosisCellular modelsPharmacogenomicsKey Points
The mood-stabilizing properties of lithium have largely been proved, but its mechanism of action is still not completely known.
Lithium interferes with a large number of cellular processes and modulates the expression of hundreds of genes.
The inositol pathway is one of the best-studied targets of lithium, and in vivo and in vitro studies show that lithium directly inhibits inositol enzymes.
It has been demonstrated that lithium is neuroprotective, a feature that partly depends on its effects on the expression of neurotrophic factors and pro- and anti-apoptotic genes.
A growing body of evidence also shows that lithium modulates the expression of genes of the circadian system, the polyamine system and genes involved in neuronal plasticity.
In their entirety, findings show that lithium modulates gene expression, and while the underlying mechanisms are still under investigation, interaction with epigenetics and non-coding RNAs seems to play a key role in lithium’s mechanism of action.
4.1 Introduction
Lithium has been used in the management of bipolar disorder for over 50 years, being effective in the acute phases of illness (manic and depressive) and in the prevention of manic and depressive recurrences (Gitlin and Frye 2012; Malhi et al. 2012). Lithium is also effective in preventing suicidal behaviour, an effect of high importance in bipolar disorder in which suicide represents one of the leading causes of death (Baldessarini et al. 2006). We have yet to discover whether these clinical effects share the same pharmacological mechanisms.
Response to long-term lithium treatment is heritable, and a large body of evidence suggests that genetic determinants play a key role in modulating the degree of response. The first studies exploring the genetics of lithium response in bipolar disorder used the candidate gene approach. These studies explored specific biological hypotheses by genotyping polymorphisms located within or nearby genes potentially involved in the mechanism of action of lithium, or in the pathophysiology of bipolar disorder, using populations of patients with different responses to lithium and controls or families of affected individuals. The assumption of candidate gene association studies is that the polymorphisms selected for investigation could be directly or indirectly responsible for altered transcription activity or protein structure, therefore negatively affecting cell functioning. These alterations could ultimately be involved in the predisposition to the disorder or in the modulation of response to medications. Besides gene polymorphisms, candidate gene studies have also focused on the role of differences in gene expression levels in lithium response. Altered expression of a gene, or set of genes, could either be the cause or the consequence of dysregulations involved in modulating different responses to lithium treatment. Identification of genes differentially expressed between different groups of subjects acquires different biological meaning depending on the tissue used. For instance, studies of brain tissue provide information that is highly relevant for the understanding of the biological basis of a disorder, but these studies are usually not applicable for pharmacogenomics. On the other hand, peripheral tissues and patient-derived cell lines allow investigation of the effects of drugs in vivo or in vitro, and findings from these studies may lead to the identification of peripheral correlates of the phenotype, the so-called peripheral biomarkers.
Regardless of the tissue used, differential expression of genes between patients with different responses could be determined by different causes, such as the presence of polymorphisms in regulatory regions of the gene, or differences in epigenetic regulation of gene transcription. For the most part, the effects of lithium on gene expression have been investigated using animals, usually rats or mice, or human-derived cell lines. However, studies performed on patient-derived tissues represent the most valuable resource in this field, as they have the potential to identify markers of clinical response and drug targets. To date, these studies have provided intriguing findings, but a clear understanding of lithium’s effects on gene expression, and how this correlates with clinical outcome, is still far from complete. A significant contribution to the field has been the advent of high-throughput technologies. These allow hypothesis-free approaches to explore the entire genome, generate new hypotheses and identify new genetic candidates for further investigation. Nowadays, DNA arrays allow the genotyping of several millions of single nucleotide polymorphisms (SNP) in one single array, providing a large set of genetic information with high coverage of the genome. On the other hand, RNA arrays (for messenger RNA or non-coding RNA) are designed to measure the expression of hundreds of thousands of genes in one single array and can be used with RNA extracted from any tissue. The number of studies applying these technologies on populations of patients characterized for lithium response is still scarce, but several international efforts are ongoing, and findings will soon be available.
In their entirety, data from candidate gene and hypothesis-generating studies in both animals and humans have provided evidence for the involvement of a number of genes and pathways in lithium’s mechanism of action and in lithium response. It is well documented that lithium modulates the expression of hundreds of genes involved in key cellular processes, such as second messengers, transcription factors, elements involved in cell signalling, oncogenes, tumour-suppressor genes and many others. However, a proportion of these modifications are likely unspecific and not related to those mechanisms involved in the mood-stabilizing effects of lithium. It is therefore of crucial importance to identify which genes are key modulators of lithium’s effects and how changes in their expression correlate with the clinical response. In this chapter, we outline the concepts of gene expression regulation and focus on genes and pathways for which decades of research have provided the most compelling findings. We give particular emphasis to findings from studies carried out on patient-derived tissues, as these studies have the potential to identify those genes and proteins involved in modulating the clinical efficacy of lithium.
4.2 Genes of the Inositol Phosphate Pathway
Early findings show that several enzymes of the inositol pathway are directly inhibited by lithium. As a consequence of this evidence, different strategies have been used over the years to explore and extend our understanding of the interaction between lithium and inositol and how this may correlate with clinical response to lithium treatment. To date, only a few hypothesis-based studies have investigated lithium’s effects on the expression of inositol genes in patient populations, reporting for the most part negative findings. In this section we will discuss the inositol pathway and present findings from animal and human studies showing lithium’s effects on inositol homeostasis, as elements of this pathway are also key up- or downstream effectors of other lithium targets, as discussed in later sections of this chapter.
Lithium directly interacts with enzymes of the inositol pathway. This knowledge led to the development of the ‘inositol-depletion hypothesis’ as a key component of the mechanism of action of lithium (Berridge 1989). The inositol phosphate pathway is a second messenger system with a crucial role in signal transduction. It comprises the lipid phosphatidylinositol 4,5-bisphosphate (PIP2) (a minor component of the cell membrane) and two second messengers derived from its hydrolysis: diacylglycerol (DAG) and inositol trisphosphate (IP3). Hydrolysis of PIP2 is catalyzed by the membrane enzyme phospholipase C (PLC) and is regulated by neurotransmitters such as serotonin and acetylcholine. DAG is located in the cell membrane, where it activates protein kinase C (PKC), while IP3 is a soluble molecule able to diffuse into the cell and increase intracellular calcium concentration. IP3 is metabolized to myo-inositol, which is recycled to synthesize new PIP2. Lithium at therapeutic concentrations directly inhibits two key enzymes of inositol metabolism: inositol monophosphatase (IMPase) and phosphatase inositol-1,4 bisphosphate 1-phosphatase (IPPase). IMPase inhibition causes a reduction of myo-inositol concentration (Allison and Stewart 1971) and, as a result, decreases inositol recycling.
According to the inositol-depletion hypothesis, lithium may exert its therapeutic effect in pathological states (leaving subjects with normal behaviour unaffected) via its uncompetitive inhibition of IMPase, which is more pronounced with high substrate concentration, as in the case of a pathologically overactivated system (Fauroux and Freeman 1999). Another hypothesis is that lithium-induced inositol depletion could be mediated by inhibition of glycogen synthase kinase 3 beta (GSK3B). Using the yeast Saccharomyces cerevisiae with a null mutation for GSK3B, Azab and coworkers showed that GSK3B is required for optimal inositol synthesis and loss of GSK3B activity leads to inositol depletion (Azab et al. 2007).
Interestingly, inhibition of the inositol pathway by lithium was also suggested by a gene expression study carried out in lymphoblasts from bipolar patients responsive to lithium and from healthy controls. Using a hypothesis-free approach, the study by Sun and coworkers (Sun et al. 2004) measured the expression of 2,400 cDNAs in lymphoblasts cultured with or without lithium chloride. Alpha1B-adrenoceptor (a1B-AR) was one of the genes most significantly downregulated by lithium exclusively in the bipolar sample. A1 adrenergic receptors are members of the G protein-coupled receptor superfamily. These receptors activate mitogenic responses and regulate growth and proliferation through the Gq/11 family of G proteins coupled with phospholipase C (PLC). Activation of PLC hydrolyses phosphatidylinositol 4,5-biphosphate (PIP2) to yield DAG and IP3, resulting in activation of PKC and release of intracellular calcium. The lithium-induced reduction of a1B-AR reported by Sun and colleagues may lead to a depletion of inositol levels, as it could be responsible for a reduction of inositol-generated second messengers. These findings suggest that lithium may regulate the inositol pathway not only via a direct inhibition of IMPase and IPPase but also by inhibition of a1B-AR expression.
In clinical studies, findings so far have been inconclusive: lithium has been reported to decrease brain myo-inositol levels in the right frontal lobe of bipolar patients (Moore et al. 1999), but other studies do not support this finding (Silverstone and McGrath 2009).
In summary, the interaction of lithium with the inositol pathway is one of the most corroborated hypotheses of lithium’s mechanism of action, but to date there has been no compelling evidence that this system is implicated in modulating the clinical response to lithium treatment in bipolar patients.
4.3 Genes Involved in Apoptosis and Neuroprotection
The neuroprotective effects of lithium have been widely reported. These effects are mediated by the prevention of apoptotic-dependent cellular death through the regulation of neurotrophic factors and different signalling pathways. Data from animal and human studies show that modulation of the expression of genes implicated in neuronal growth is a key mechanism in lithium-induced neuroprotection. In this section we present converging evidence of the neuroprotective effect of lithium, focusing on studies showing lithium regulation of the expression of genes involved in neuroprotection, apoptosis and other neuronal functions.
It has been shown that lithium treatment interferes with glutamate activity in neurons.
Modulation of glutamate activity is an important mechanism in neuroprotection, as excessive release of glutamate induces excitotoxicity. Glutamate-induced excitotoxicity has been implicated in many neurodegenerative diseases. In this process, stimulation of N-methyl-D-aspartate (NMDA) receptors by glutamate causes an excessive influx of calcium into the cells, thus activating enzymes that damage cell structure. To test the hypothesis that lithium is protective against glutamate-induced excitotoxicity, Jakopec and colleagues explored the effect of lithium pretreatment on human glioblastoma cells exposed to cytotoxic concentrations of glutamate (Jakopec et al. 2008). Findings showed that lithium reverted glutamate effects through the modulation of the expression of genes with a critical role in cell survival and signalling, such as survivin and p21, two proteins with anti-apoptotic function.
It has also been suggested that lithium regulates mitochondrial function. In a study by Chen and coworkers, lithium doubled levels of B-cell lymphoma 2 (Bcl-2) in the frontal cortex of rats (Chen et al. 1999). Bcl-2 is a key member of the best characterized family of regulatory proteins involved in inducing or inhibiting apoptosis. Bcl-2 has a key role in promoting cell survival by preventing the mitochondrial release of cytochrome C. After being released into the cytoplasm in response to pro-apoptotic stimuli, cytochrome C triggers cellular death by activating caspases. Bcl-2 family members regulate this signalling pathway through the formation of complexes that stabilize the mitochondrial membrane (anti-apoptotic members) or increase its permeability (pro-apoptotic members). The exact mechanism by which lithium increases Bcl-2 expression is not completely understood. However, the study by Chen and colleagues suggested that the increase in Bcl-2 levels might be determined by an increased expression and DNA-binding activity of a Bcl-2 regulator, the transcription factor polyomavirus enhancer-binding protein (PEBP) 2B. Another study showed that lithium not only increases Bcl-2 levels but also prevents their reduction induced by 1-methyl-4-phenyl-1,2,3,6-tetrahydropyridine (MPTP) in mice (Youdim and Arraf 2004). Lithium treatment has also been shown to decrease the expression of the pro-apoptotic gene Bcl-2-associated X protein (Bax) (Sugawara et al. 2010) and protect against methamphetamine-induced toxicity, limiting the decrease of Bcl-2/Bax ratio in the prefrontal cortex of rats (Bachmann et al. 2009). Bax encodes for a member of the Bcl-2 protein family that promotes apoptosis by inducing cytochrome C release, caspase activation and DNA fragmentation through loss of integrity of the mitochondrial membrane. Bax forms a heterodimer with Bcl-2, inhibiting its anti-apoptotic activity (Yang and Korsmeyer 1996). Bax expression is induced by tumour protein p53 (p53), one of the most studied tumour-suppressor genes. p53 is a key regulator of cell survival, being involved in transcriptional activation of genes involved in arrest of the cell cycle, activation of DNA repair enzymes and, in the case of irreparable DNA damage, apoptosis. Interestingly, chronic treatment with lithium increases Bcl-2 expression and decreases mRNA and protein levels of Bax and p53 in cultured rat cerebellar granule cells (CGC), suggesting that the neuroprotective effect of lithium may be partially mediated by p53 inhibition (Chen and Chuang 1999).
Another element that could contribute to increased Bcl-2 levels after lithium treatment is the cAMP-responsive element-binding protein (CREB). The phosphorylated form of CREB (pCREB) acts in the nucleus as a transcription factor affecting the expression of a number of genes and has a critical role in promoting cell survival, as supported by the fact that genetically engineered mice with CREB deletion die immediately after birth (Rudolph et al. 1998). Effects of lithium on CREB expression were reported in a hypothesis-free study, where authors used microarrays to measure genome-wide expression levels in substantia nigra pars compacta of mice fed with an ordinary diet or a lithium diet (Farah et al. 2013). Mice were treated with saline or MPTP in a total of four groups. Results showed that levels of CREB were upregulated by lithium, downregulated by MPTP and maintained in mice treated with both MPTP and lithium.
As regards human studies, Grimes and Jope conducted several experiments in human neuroblastoma SH-SY5Y cells in order to understand the role of CREB in the mechanism of action of lithium (Grimes and Jope 2001). The results of these studies suggested that lithium could enhance CREB DNA-binding activity through inhibition of glycogen synthase kinase 3 beta (GSK3B), a serine/threonine kinase involved in neuronal cell development, apoptosis, Wnt signalling and regulation of transcription factors. To date, only one study has investigated the effect of lithium on CREB and pCREB in cell lines derived from bipolar patients (Alda et al. 2013). This study showed that pCREB levels were increased in lymphoblasts from bipolar patient responders to lithium and in their relatives, as compared to controls. However, culturing lymphoblasts with lithium did not affect CREB and pCREB protein levels.
As mentioned earlier, lithium interaction with CREB is in part mediated by GSK3B. GSK3B is a key component of numerous signalling pathways and an important regulator of neuronal plasticity and cell survival. Its role in inducing apoptosis has been investigated in a large number of models of neuronal death (Hetman and Xia 2000; Li et al. 2000). GSK3B also represents one of the most extensively studied lithium targets. Lithium inhibits this enzyme directly, through competition with magnesium ions, and indirectly, increasing phosphorylation of a serine residue in the N-terminal domain. GSK3B is a critical activator of apoptosis through multiple mechanisms. In particular, GSK3B directly phosphorylates Bax (Linseman et al. 2004), thus activating caspases and apoptosis through the mechanism explained earlier. Moreover, GSK3B phosphorylates p53, therefore increasing its apoptotic action (Turenne and Price 2001). A recent study carried out in neuroblastoma SH-SY5Y cells showed that lithium and SB216763, an inhibitor of GSK3B, reduce mitochondrial translocation of p53 and block the mitochondrial apoptosis pathway induced by DNA-damaging agents doxorubicin, etoposide and camptothecin (Ngok-Ngam et al. 2013). These results suggest that inhibition of GSK3B could have a critical role in mediating lithium’s neuroprotective effects. Lithium has also been shown to modulate the expression of other members of the Bcl-2 family. Chronic but not acute treatment with lithium or valproate increases Bcl-2-associated athanogene (BAG-1) expression in the rat hippocampus (Zhou et al. 2005). BAG-1 binds to Bcl-2, increasing its anti-apoptotic function and activating the extracellular signal-regulated kinase/mitogen-activated protein kinase (ERK/MAPK) cascade. Upregulation of BAG-1 is not induced by chronic treatment with amphetamine, haloperidol or fluvoxamine, suggesting a specific effect of the two mood stabilizers.
A significant regulation of the expression of pro- and anti-apoptotic genes has also been shown by a microarray expression study carried out in whole blood from 20 depressed bipolar patients treated with lithium in an 8-week open-label protocol (Lowthert et al. 2012). In this study, 127 genes showed differential expression in responders versus nonresponders, with significant upregulation of anti-apoptotic genes and downregulation of pro-apoptotic genes. Among the anti-apoptotic genes were Bcl-2 and insulin receptor substrate 2 (IRS2), while pro-apoptotic genes included Bcl-2-antagonist/killer 1 (BAK1) and Bcl-2-associated agonist of cell death (BAD).
Another element that has been suggested as being involved in mediating the neuroprotective effect of both lithium and valproate is SIX homeobox 1 (SIX1). The SIX1 gene codifies for a transcription factor involved in the regulation of cell proliferation, apoptosis and embryonic development, playing an important role in the development of organs. A recent study showed that lithium and valproate increase the expression of SIX1 in neuroblastoma SH-SY5Y cells and that pre-exposure to both drugs provides protection against staurosporine (STS)-induced apoptosis. Moreover, removal of the SIX1 protein via siRNA antagonizes the ability of lithium and valproate to protect neuroblastoma cells from STS-induced apoptosis.
A large body of evidence supports the neuroprotective effects of lithium. Several potential effectors have been proposed and explored, but only a small number of these are supported by compelling findings. Bcl-2, CREB and GSK3B represent the most promising genes, as converging data from animal and human studies support an interaction between lithium and their expression and activity as well as their possible role in lithium-induced neuroprotection. Considering that these genes are members of a large number of pathways, through their regulation, lithium could interfere with many cellular functions involved in apoptosis and neuroprotection.
4.4 Genes Involved in Neuronal Plasticity
A large number of studies suggest that bipolar disorder is associated with impairments in neuronal plasticity, neurotrophic factors and glial and neuronal death in several brain areas, including those involved in mood regulation. Neurotrophic factors are critical regulators of plasticity and cell resilience in adult neurons and glia. Lithium has been shown to interfere with the expression of the key member of the nerve growth factor family, brain-derived neurotrophic factor (BDNF), a protein involved in promoting neuronal differentiation in the peripheral and central nervous systems. BDNF participates in axonal growth, in path finding and in the modulation of dendritic growth and morphology. BDNF activates tyrosine kinase receptor B (TrkB), by which it regulates intracellular signalling pathways involved in neuronal survival, synaptic plasticity and cognitive functions. A number of human studies have tested the hypothesis of the involvement of BDNF in the mechanism of action of lithium. Croce and coworkers showed that lithium abolished the excitotoxic effect of high concentrations of glutamate and that this effect was associated with increased BDNF protein and mRNA levels in human neuroblastoma cell lines (Croce et al. 2014). However, the most compelling findings supporting a lithium-BDNF interaction come from studies carried out in patient-derived cells. Tseng and colleagues (2008) explored the effect of lithium on BDNF levels in lymphoblasts derived from 12 lithium-responsive bipolar patients and 13 healthy controls. Bipolar subjects showed lower protein levels compared to controls. Interestingly, lithium treatment reduced these levels, which remained lower in the bipolar group.
In another study, Pandey and coworkers (2010) investigated differences in the BDNF gene and protein levels in blood cells from bipolar patients and controls and tested the effect of lithium. BDNF levels were lower in fresh lymphocytes and platelets from 26 paediatric bipolar patients compared to 21 healthy controls. Moreover, gene and protein levels were increased in lymphocytes sampled after 8 weeks of treatment with lithium, VPA or second-generation antipsychotics compared to lymphocytes sampled before lithium treatment. The studies by Tseng and Pandey show contrasting findings in respect to the effect of treatments on BDNF levels. However, the two studies present important differences. Tseng and colleagues explored the effect of lithium alone in lymphoblasts, whereas Pandey and colleagues investigated the effect of several mood stabilizers in fresh blood without testing treatment-specific effects. Another recent study reported higher BDNF plasma levels after 28 days of lithium monotherapy in bipolar patients (de Sousa et al. 2011). Even considering the discrepancies among studies, these findings converge towards a significant lithium-mediated modulation of BDNF expression. More data on the effect of lithium on BDNF are presented in Sect. 4.8, where we discuss lithium modulation of epigenetic regulation of BDNF transcription.
Other players in neuronal plasticity have been investigated in lithium response and the mechanism of action of lithium. Following an earlier linkage study suggesting the involvement of synapsin II (SYN2) in bipolar disorder and lithium response (Lopez et al. 2010), Cruceanu and colleagues (2012) explored the effects of in vitro lithium treatment on the SYN2 gene in lymphoblasts from bipolar patients, responders and nonresponders to lithium and healthy controls. SYN2 is a neuronal phosphoprotein involved in vesicle trafficking and synaptic plasticity. In the Cruceanu study, lithium treatment upregulated the expression of the two isoforms SYN2a and SYN2b in some lithium responders, while it downregulated it in others. Interestingly, lithium treatment in neuroblastoma cells increased SYN2a expression. These findings provide evidence for an involvement of SYN and regulation of neurotransmission and synaptogenesis in the mechanism of action of lithium.
Taken together, in vitro and in vivo studies suggest that genes involved in plasticity and neuronal growth, and in particular BDNF, could represent key players in lithium’s mechanism of action.
4.5 Genes of the Circadian Rhythm System
Alteration of circadian rhythms, such as sleep disturbances and abnormalities of the sleep-wake cycle, has been reported in bipolar disorder (McCarthy and Welsh 2012). An extensive overview of the role of circadian rhythm dysregulation in bipolar disorder and lithium effects on this system is provided in Chapter 6 of this book. Here we focus on the most compelling findings supporting lithium effects on the expression of genes of the circadian system. Genes of this system have been studied to verify the hypothesis of a role for their altered expression level in the circadian rhythm disturbances observed in bipolar patients.
One of the most widely studied mechanisms regulating the expression of circadian clock genes is the feedback loop involving circadian locomotor output cycles kaput (CLOCK) protein, which plays a crucial role in promoting expression of downstream circadian genes (Takahashi et al. 2008). CLOCK encodes for a transcription factor that forms a heterodimer with the protein encoded by aryl hydrocarbon receptor nuclear translocator-like (Bmal1). During the day, the heterodimer CLOCK/Bmal1 promotes the expression of a number of genes such as period 1 (Per1), period 2 (Per2), period 3 (Per3), cryptochrome1 (Cry1) and cryptochrome2 (Cry2). PER and CRY proteins translocate into the nucleus where they inhibit their own transcription by interacting with the CLOCK/Bmal1 dimer. During the night, PER and CRY products are degraded, and the CLOCK/Bmal1 dimer is no longer inhibited. Moreover, Bmal1 expression is repressed by the nuclear hormone receptor Rev-Erb-α, which is involved in another feedback loop together with the RAR-related orphan receptor A (RORA) gene.
Evidence for an effect of lithium on genes of this system comes from both animal and human studies. In 2007, Roybal and colleagues (2007) showed that lithium reverted mania-like behaviours in mice with a CLOCK gene mutation. In another study, expression of Per2 and Cry1 was increased by lithium in murine fibroblasts, while Per3, Cry2, Bmal1 and Rev-Erb-α were reduced (Osland et al. 2011).
In regard to human studies, lithium was shown to reduce the expression of Bmal1 and Rev-Erb-α in fibroblasts of bipolar patients but not in healthy controls (Yang et al. 2009). One of the most accredited hypotheses is that lithium could interfere with circadian clock genes through the inhibition of GSK3B, although this hypothesis has yet to be confirmed (Mohawk et al. 2009; Kozikowski et al. 2011; Kinoshita et al. 2012; Li et al. 2012). In a recent study, lithium 1 mM increased Per2 rhythm amplitude in skin fibroblasts from healthy controls but not from bipolar patients, while lithium 10 mM increased period length in both bipolar cases and controls (McCarthy et al. 2013). PER3 and RORA genotypes predicted lithium period lengthening, whereas GSK3B genotype predicted rhythm effects of lithium, specifically among bipolar patients. Finally, lithium enhanced the resynchronization of damped rhythms, supporting the hypothesis that the effect on circadian period could mediate part of lithium’s therapeutic effect in bipolar disorder.
To summarize, an altered circadian system has been widely reported in bipolar patients. Studies exploring the effects of lithium on the expression of genes of this system are scarce, but findings suggest that, through GSK3B and Rev-Erb-α, lithium could interfere with the circadian system.
4.6 Genes of the Polyamine System
Systems involved in modulating the response to stress stimuli have been widely studied in bipolar disorder. However, the effects of lithium on the genes of these systems have been scarcely investigated or reported. The polyamine system has been suggested to be dysfunctional in mood disorders and suicide, and altered levels of polyamines have been reported in these disorders (Fiori and Turecki 2008). Polyamines (PAs) are ubiquitous molecules responsible for the polyamine stress response (PSR), a physiological cascade of intracellular events activated by stressful stimuli involved in the restoring and maintenance of the organism’s homeostatic equilibrium (Gilad and Gilad 1996). PAs are implicated in a variety of cell processes, including modulation of DNA, RNA and protein functions and ultimately neurotransmission (Igarashi and Kashiwagi 2010). A possible involvement of altered expression of genes of the PA system in suicide was first suggested by a microarray study exploring differences in genome-wide expression levels between suicide victims and controls. This study showed that the spermidine/spermine N1-acetyltransferase (SAT1) gene, encoding the rate-limiting enzyme of the catabolic and interconversion pathway of PAs, is downregulated in the cerebral cortex of suicide completers with and without major depression as compared to healthy controls, a finding that was replicated by independent investigations (Sequeira et al. 2006, 2007; Guipponi et al. 2009; Klempan et al. 2009). Interestingly, earlier studies showed that persistent PSR is blocked by chronic lithium treatment in the rat brain. This block results from an inhibition of the activity of the two PA enzymes, ornithine decarboxylase (ODC) and SAT1 (Gilad and Gilad 1996, 2003). However, the interaction between lithium and the PA system remains incompletely understood. A recent study (Squassina et al. 2013) showed that lithium treatment in vitro is able to increase SAT1 expression in lymphoblasts from bipolar patients with low and high genetic risk of suicide, but not in bipolar suicide completers. This study suggests that SAT1 expression is influenced by lithium and that its transcription regulation may be defective in suicide completers with bipolar disorder. Importantly, this study was carried out in peripheral cells derived from patients prior to a suicide attempt or completion. Taken together, the expression data published so far suggest that an altered expression of SAT1 may be a feature of the suicide brain and a potential target of lithium.
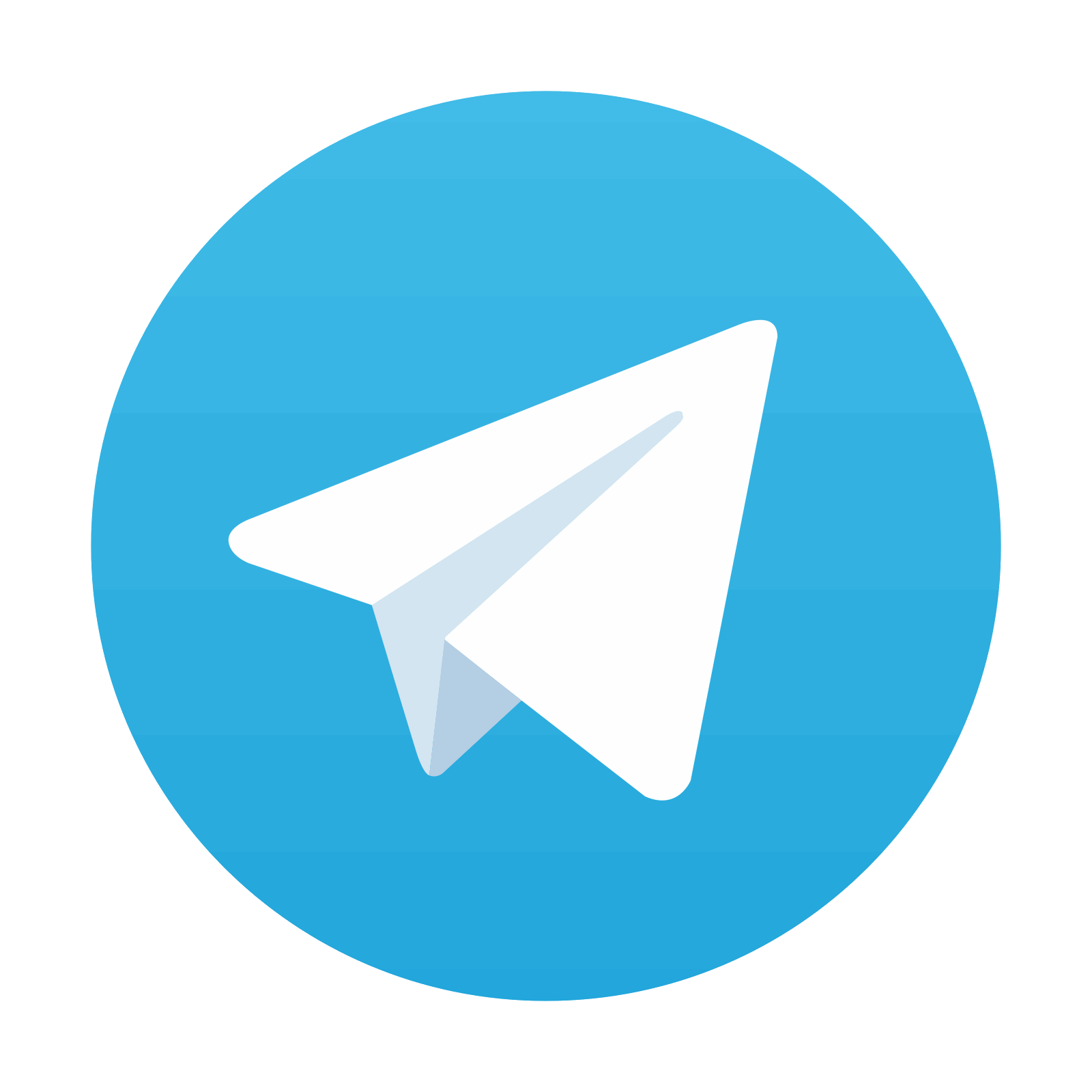
Stay updated, free articles. Join our Telegram channel

Full access? Get Clinical Tree
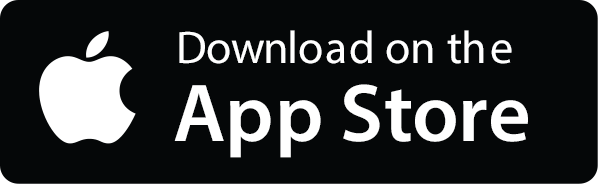
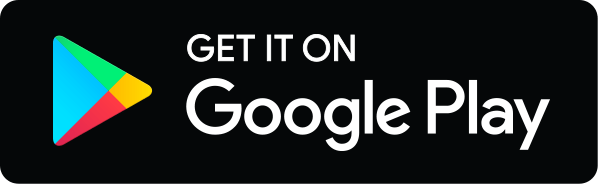