Fig. 3.1
Definitions for key terms and concepts
Family studies suggest that the risk of recurrence among siblings is between 2 and 6 %, which represents a 20–75 times greater prevalence than in the general population (Levy et al. 2009; Newschaffer et al. 2007) . The high heritability (around 80–90 % in most studies) indicates a marked genetic influence in ASD etiology. Studies involving twins have shown concordance rates ranging from 60 to 92 % among monozygotic twins and 0–10 % among dizygotic twins (Ronald and Hoekstra 2011) . However, Hallmayer et al. (2011) suggested that autism heritability is only moderate (around 38 %) and that environmental factors have a significant influence on disease etiology. In this chapter, we will discuss the main genetic conditions and mechanisms involved in the etiology of autism.
3.1.1 Genetic Models
The study of the influence of different genetic factors (including common and rare variants) on multifactorial disorders like ASD follows different genetic models. The most commonly applied model is the multifactorial polygenic model that involves the contribution of several genes and environmental factors with small effects (Abrahams and Geschwind 2008) . However, the oligogenic model could also be applied to explain the origin of ASD in some of the cases in which a few genes with moderate effects act together. The assessment of ASD domains (e.g., social, communication, and restricted and repetitive activities) shows only a modest covariation among them: individuals with extreme scores for the domains do not necessarily have similar scores in other areas. Nonetheless, these data suggest that the genetic susceptibility to ASD and to its phenotypic presentation results from the influence of several genes and their interactions, as well as the involvement of environmental factors (Ronald et al. 2006) .
Family studies can also help the identification of unique genes with strong effects related to various aspects of the disease. Analysis of data from these studies indicated that some cases are associated with dominant de novo mutations (Abrahams and Geschwind 2008). These mutations appear to have a high penetrance in boys and low penetrance in girls, and also would act primarily in no familiar or sporadic ASD cases (Zhao et al. 2007) . The identification of rare variants with a strong impact is extremely relevant to understanding ASD, and their phenotypic effect could be dependent on the genetic background in which rare variants occur (McClellan and King 2010) .
The varied clinical manifestations present in ASD indicate that both common and rare genetic variants have an influence on its etiology and development. Based on current knowledge, the best genetic model for ASD should involve genetic analysis of more homogeneous subgroups sharing similar clinical characteristics. Additionally, the independent heritability in autism central areas is also important and should not be ignored (Anney et al. 2012) .
3.2 Syndromes
Some genetic abnormalities are identified and associated with known genetic disorders, especially monogenic or chromosomal syndromes. However, until now, only around 10–15 % of ASD cases have been associated with known genetic factors. It is known that common biological bases, such as the disruption of molecular pathways and brain circuits, underlie different disorders, and this could explain the comorbidity of these syndromes with autism. Fragile X syndrome (FXS) is the most common, being identified in 3–5 % of ASD patients. Cytogenetic analyses may indicate the presence of known syndromes, transpositions, deletions, insertions, or other chromosomal rearrangements. Studies have estimated the frequency of these abnormalities in an additional 10 % of ASD sufferers (Zafeiriou et al. 2013) .
3.2.1 Fragile X Syndrome (FXS)
FXS is considered to be the most common known condition of a single gene associated with ASD, affecting up to 5 % of cases. FXS is caused by expansion of the CGG trinucleotide repetition in the 5ʹ-UTR region of the fragile X mental retardation (FMR1) gene (Wheeler et al. 2014; Steinberg and Webber 2013) . The largest expansion of the CGG region—greater than 200 repetitions—is called a full mutation and it corresponds to FXS. Individuals with the FMR1 pre-mutation have a CGG expansion ranging between 55 and 200 repetitions (McCary et al. 2013) . FXS is characterized by intellectual disorder, anxiety, hyperactivity/impulsivity, and high rates of autism symptoms. Approximately 90 % of male individuals with FXS display at least one autistic behavioral feature (Brock and Hatton 2010) . The prevalence of autism in FXS patients ranges from 15 to 52 % in different studies, depending on the diagnostic methods used (Zafeiriou et al. 2013) .
The FMR1 gene encodes the fragile X mental retardation protein (FMRP), which has an essential role in synaptic plasticity because it regulates the transport of mRNA and the translation in the brain, as well as regulates different pathways associated with autism (Liu and Takumi 2014) . Previous studies have suggested that almost half of the genes involved in autism may be target genes of FMRP, leading to the hypothesis of the important role of FMRP in synaptic plasticity and in the development of autistic characteristics (Iossifov et al. 2012; Darnell et al. 2011) . The absence of FMRP leads to significant changes in cognitive functions, dendritic spine morphology, and intracellular signaling. Studies have shown that children with FXS and ASD concomitantly exhibit weaker social and communicative skills, lower adaptive behavior, greater behavioral problems, and greater cognitive impairment than boys with FXS or idiopathic autism alone (Wheeler et al. 2014; Wolff et al. 2012) .
3.2.2 Tuberous Sclerosis
Tuberous sclerosis complex (TSC) is an autosomal dominant disorder caused by inherited loss of function (30 %) or de novo (70 %) mutations in the TSC1 or TSC2 genes (Ghosh et al. 2013) . Both these genes act as inhibitors in the target pathway of rapamycin (mTOR), a key pathway that controls the local translation at the synapse (Ghosh et al. 2013; Liu and Takumi 2014) . The mTOR enzyme is an essential component of two complexes, mTORC1 and mTORC2, which play important roles in gene transcription , protein translation, and cell proliferation. Mutations in the TSC1 or TSC2 genes lead to excessive mTOR activity, which may cause disruption of the synaptic plasticity, characterized by excessive glutamate activity (Gipson et al. 2013) .
TSC, one of the main causes of syndromic autism, is characterized by multisystemic hamartomas and debilitating neurological deficits, including seizures, intellectual disability, and ASD (Kothare et al. 2014) . Autism has been reported in 26–61 % of individuals with TSC, with an average prevalence of 32 % (Gipson et al. 2013). Conversely, the prevalence of TSC among people with autism is 0–4 % (Fombonne 2003) . People with TSC who develop ASD, display higher rates of intellectual disability, infantile spasms, and temporal lobe epilepsy (Gipson et al. 2013) .
3.2.3 Rett Syndrome
Rett syndrome (RTT, OMIM 312750) is a neurodevelopmental disorder that occurs in about 1 in every 10,000 girls born. Up until the publication of DSM-IV, it had been classified as a pervasive developmental disorder. With the clear identification of the mutation causing RTT, it was excluded as an ASD in DSM-5.
RTT occurs most frequently in girls, although a small number of boys with the syndrome have been identified. Although approximately 95 % of RTT cases are caused by mutations in the methyl CpG-binding protein 2 (MECP2) gene, there is a well-established phenotypic heterogeneity. Approximately 80 % of the clinical cases of RTT present the typical clinical picture. After a seemingly normal development, at around 6–18 months of age, girls with RTT show neurological regression, lose their acquired cognitive, social, and motor skills, and develop autistic behavior accompanied by characteristic hand movements (Signorini et al. 2013). The autistic characteristics are typically temporary in RTT (Castro et al. 2013) .
The nuclear protein MECP2 was initially associated with methylated DNA due to the binding of CpG islands (regions rich in guanines and cytosines). Additionally, a transcriptional repression domain that blocks the transcription of mRNA was identified in MECP2, which raised the initial hypothesis that MECP2 would only act as a regulating silencer of chromatin (Castro et al. 2013; Kishi and Macklis 2005) . However, subsequent evidence has demonstrated a range of complex roles performed by MECP2. Besides also operating as a splicing modulator of RNA, MECP2 acts as a transcriptional activator by binding to the cAMP response element-binding (CREB) protein (Young et al. 2005; Chahrour et al. 2008) and playing a role in regulating the expression of microRNAs that are important for brain development and brain plasticity (Wu et al. 2010; Urdinguio et al. 2010) . Studies have shown that through its different means of regulation, MECP2 is capable of indirectly controlling a range of molecules responsible for local protein synthesis and synaptic plasticity, which would be involved in RTT (Castro et al. 2013) .
3.2.4 PTEN Hamartoma Tumor Syndrome (PHTS)
PTEN hamartoma tumor syndrome (PHTS) refers to a spectrum of disorders related to germline mutations in the homologous gene of phosphatase and tensin (PTEN), which include Cowden syndrome, Bannayan–Riley–Ruvalcaba syndrome, Lhermitte–Duclos disease, and the ASD associated with macrocephaly (Pilarski et al. 2013) . The PTEN gene, which is located on chromosome 10, plays an important role in brain development. Studies indicate that germline mutations in this gene result in macrocephaly, seizures, and mental retardation (Lv et al. 2013) . Subsequent biochemical studies have demonstrated that PTEN acts as a negative regulator of the phosphatidylinositol-3-kinase (PI3K)/AKT signaling pathway, which is an essential pathway for regulating the growth, survival, and proliferation of cells. PTEN acts by offsetting the action of PI3K, thereby inhibiting the activation of AKT and indirectly suppressing the mTOR pathway . Abnormalities in the PI3K/AKT/mTOR pathway lead to neurological and psychiatric disorders such as brain tumors, autism, and schizophrenia (Lv et al. 2013).
Studies on the frequency of PTEN mutations in patients with ASD indicate a variation of 1–17 %. It is possible that such discrepancies occur due to genetic variations among the populations of each study (Lv et al. 2013). Studies in mice have confirmed that deletions in PTEN result in long-term changes in social behavior, repetitive behavior, locomotor activity, and anxiety, resulting in many behavioral characteristics of autism (Lugo et al. 2014) .
Besides the aforementioned syndromes, many others can also occur concomitantly with ASD. Table 3.1 presents a summary of the main syndromes associated with ASD.
Syndrome | Gene/chromosomal region | Clinical characteristics | Prevalence in the population | Prevalence of the syndrome in the ASDs | Prevalence of ASD in the syndrome |
---|---|---|---|---|---|
Fragile X | FMR1 | Intellectual disability, anxiety, hyperactivity/impulsiveness, autistic features | 1/5160 boys | 0–5 % | 15–52 % |
Tuberous sclerosis | TSC1, TSC2 | Benign tumors in various organs; seizures, delayed development, behavioral problems | 1/25,000 | 0–4 % | 26–61 % |
Rett | MECP2 | Neurological regression, loss of acquired cognitive, social and motor abilities, autistic behaviors, stereotypical hand movements | 1/10,000 girls | < 1 % | 100 % |
PTEN Hamartoma tumor | PTEN | Macrocephaly, seizures intellectual disability | 1/200,000–1/250,000 | 1–17 % | 1–5 % |
2q37 deletion | 2q37.1–2q37.3 | Mild to moderate intellectual disability, distinctive facial features, short stature, hypotonia, obesity, cardiac defects | Unknown | 1.2 % | 17–50 % |
Angelman | UBE3A (15q11.2) maternal allele | Microcephaly, intellectual disability, severe impairment, movement and sleep disorders, characteristic behavior | 1/40,000 | < 1 % | 50–60 % |
Prader–Willi | 15q11–q13 paternal chromosome | Hypotonia, hyperphagia, obesity, short stature, motor and speech developmental delay, behavioral problems | 1/25,000–1/45,000 | < 1 % | 19–36, 5 % |
22q11.2 deletion DiGeorge | 22q11.2 microdeletion | Congenital cardiac defect, cleft palate, immune deficiency, learning disabilities, distinctive facial features | 1/1600–1/2000 | < 1 % | 14–50 % |
22q13 deletion Phelan-McDermid | 22q13.3 deletion | Developmental delay, moderate to profound intellectual disability, hypotonia, and absent or delayed speech | Unknown | < 1 % | 50–70 % |
Down | Chromosome 21 trisomy | Intellectual disabilities, motor developmental delay, characteristic facial features | 1/1000 | 3.7 % | 15.6–19 % |
Neurofibromatosis type 1 | NF1 | Changes in skin pigmentation, benign tumors along nerves in the skin, brain, and other parts of the body | 1/2500–1/3000 | 1.2 % | 4 % |
Turner | Chromosome X monosomy | Short stature, premature ovarian failure, webbed neck, lymphedema, coarctation of the aorta, normal intelligence | 25–50/100,000 girls | Unknown | 3 % |
Klinefelter | Extra X chromosome in males (47,XXY) | Small testes, low production of testosterone cryptorchidism, delayed or incomplete puberty, gynecomastia, infertility | 40–172/100,000 boys | Unknown | 11–27 % |
Smith–Lemli–Opitz | DHCR7 | Craniofacial abnormalities, intellectual disabilities, behavior abnormalities | 1/10,000–1/60,000 | < 1 % | 46–52 % |
Cohen | COH-1 | Hypotonia, intellectual disabilities characteristic facial features, microcephaly | 1/105,000 | < 1 % | 48 % |
Cornelia de Lange | NIPBL, SMC1A, SMC3 | Slow growth before and after birth, intellectual disability that is usually severe to profound, skeletal abnormalities involving the arms and hands, and distinctive facial features | 1/10,000 | < 1 % | 46–67 % |
3.3 Genetic Approaches
3.3.1 Linkage Studies
Linkage studies were a pioneering approach to the investigation of genetic factors in ASD. These studies evaluated families with individuals from different generations. The transmission of a chromosomal segment (locus) was verified from one generation to another, and cosegregation was sought between selected loci and the phenotype. This methodology allows identification of chromosomal regions, which may have more than one specific locus, thus suggesting the influence of different genes. The studies mainly identified regions in chromosomes 2 and 7, and also other regions in chromosomes 1, 5, 16, and 17 (Gupta and State 2006) . More recently, other studies have indicated positive results in different regions in chromosomes 4, 6, 11, 15, 20, and 22 (Freitag et al. 2010; Fradin et al. 2010; Liu et al. 2008; Weiss et al. 2009) . The 7q region is an area of particular interest, since many chromosomal rearrangements have been identified and some genes have been related to brain expression and other known physiological functions, possibly involved in the pathology of ASD (Gupta and State 2006) .
3.3.2 Association Studies
Genetic association studies aim to identify genes or genomic regions that could influence a specific phenotype or disease. There are two main approaches that involve the study of: (1) candidate genes or (2) genome scans.
The candidate gene approach investigates common genetic variants located in genes associated with metabolic pathways and biological processes that are possibly related to phenotypes or diseases. There are two main methods to research: case control and family based. Case-control studies compare the allelic and genotypic frequencies in two groups of individuals: affected or unaffected by a given disease. Family-based studies observe the allele transmission from parents to affected offspring, comparing the data observed in the sample with the data expected by chance (Eley and Rijsdijk 2005) .
Using these approaches, more than 100 genes have been evaluated, with both positive and negative results. Usually, the genes analyzed are those encoding components related to neurotransmitter metabolism (e.g., serotonin and glutamate), neural migration, neurodevelopmental control, neuronal cell adhesion, as well as genes involved in speech and language disorders, cognition, social interaction, and behavior. Also, chromosome break points in structural arrangements can be useful in identifying genes that have a major effect on ASD.
Some of the key candidate genes and common variants investigated in ASD studies are discussed below. They were chosen based on the AutismKB database (http://autismkb.cbi.pku.edu.cn/association_gene.php), considering the association score and the number of published studies.
3.3.2.1 Genes Involved in Neurotransmission
Serotonin is an essential neurotransmitter in brain functions such as sensory processing, cognition, imitation, communication, emotional and autonomic responses, regulation of motor activity, and it is also involved in the hormonal control of the hypothalamic and pituitary gland. The evaluation of serotonin in ASD is based on at least three major factors: its importance in neurodevelopment, its role in the treatment of symptoms, and the changes in blood/plasma levels in patients (Cook and Leventhal 1996; Whitaker-Azmitia 2005; Hollander et al. 2005) . Neuroimaging studies have revealed that the peak capacity of brain serotonin synthesis occurs at around 2 years of age in children with typical development; however, this peak is not observed in children with autism (Chandana et al. 2005) . The serotonin transporter (5HTT) is considered to be one of the main regulators of serotonergic function, and it is widely studied in ASD. The gene encoding the 5HTT (SLC6A4) is located on chromosome 17q11.1–q12. The 5HTTLPR (serotonin transporter-linked polymorphic region) is the most investigated variant among all described in the SLC6A4 gene. The findings in several studies have been inconsistent—there were positive results for different alleles, and also negative data were observed. A more recent meta-analysis found no association between 5HTTLPR and ASD (Huang and Santangelo 2008) . The assessment of clinical subgroups (endophenotypes) has been described in some studies, with different alleles being associated with deficits in communication and social interaction, as well as repetitive movements and aggressiveness (Brune et al. 2006) .
The GABRB3 gene is located in the 15q11–q13 region and it encodes one of the gamma-aminobutyric acid (GABA) receptors (Tierney et al. 2012) , which is one of the largest inhibitory neurotransmitters in the mammalian nervous system. In this chromosomal region, there are two other genes that also encode GABA receptor subunits (GABRA5 and GABRG3). According to linkage studies, such a region is considered to be a candidate for autism. Maternal or paternal deletions in the region are related to Angelman or Prader–Willi syndrome, respectively, which are diseases whose symptoms are associated with ASD (Buxbaum et al. 2002) . The influence of the GABRB3 gene in ASD has been evaluated in some studies, with some positive results already being detected (Buxbaum et al. 2002; McCauley et al. 2004; Curran et al. 2006) .
The SLC25A12 gene is located at 2q24, a region that is susceptible to ASD. It encodes a mitochondrial calcium-binding carrier protein, which is involved in the exchange of aspartate for glutamate via the inner mitochondrial membrane. It also regulates the cytosolic redox state and is an important supplier of energy to the neurons in the central nervous system (Durdiakova et al. 2014) . Due to having an important role in pathways that are altered in autism, the SLC25A12 gene has been indicated as one of the candidates for the disorder. Different common variants of the gene have been evaluated in several studies and some positive associations with autism have been shown (Silverman et al. 2008; Ramoz et al. 2004; Segurado et al. 2005) .
The GRIK2 gene encodes the glutamate 6 receptor and is considered to be a candidate because of its location at 6q21, a linkage region for autism. Glutamate receptors are the predominant excitatory neurotransmitter receptors in the brain and they are activated in a variety of normal neuropsychological processes. Due to their involvement in cognitive functions such as memory and learning, studies on the association of GRIK2 with ASD were performed and some gene variants were positively associated (Shuang et al. 2004; Jamain et al. 2002; Kim et al. 2007) .
3.3.2.2 Genes Involved in Cell Adhesion Molecules (CAMs)
Cell adhesion molecules (CAMs) mediate cell–cell and cell–extracellular matrix communication. They have an essential role in neurodevelopment, especially with regard to the formation and functioning of synapses, and they display specific expression patterns.
The β3 integrin (ITGB3) is the most investigated molecule in genetic studies of ASD, specifically involving synapses (Chavis and Westbrook 2001) . Moreover, through different approaches, it was possible to infer that ITGB3 is associated with serotoninergic neurotransmission, due to its interaction with the serotonin transporter (Whyte et al. 2013; Ye et al. 2010) . The ITGB3 gene is located on chromosome 17q21.3. Different variants have been associated with ASD, either isolated or in gene–gene interactions, but mostly with the SLC6A4 gene. The positive results involve different common variants across the studies (Weiss et al. 2006a; Schuch et al. 2014; Coutinho et al. 2007; Ma et al. 2010; Singh et al. 2013; Weiss et al. 2006b) .
The gene from the neuronal CAM (NRCAM) is located at 7q31, a candidate region for autism, and it encodes a member of the CAM immunoglobulin superfamily. The NRCAM protein is present at high levels in the brain and in the spinal cord during development of the nervous system, and it interacts with other molecules to promote directional signaling during growth of the axonal cone (Marui et al. 2009) . Some authors have investigated and found associations between variants in the NRCAM gene and ASD (Marui et al. 2009; Bonora et al. 2005) .
3.3.2.3 Other Candidate Genes
The gene of the oxytocin receptor (OXTR) is located on the 3p25 chromosome and it encodes a protein from the family of receptors coupled to G-proteins. OXTR regulates the activity of the neuropeptide oxytocin (OXT), and both have been associated with the establishment of maternal and social behavior (Feldman et al. 2007; Ebstein et al. 2009; Di Napoli et al. 2014) . Due to its role in social and emotional characteristics, the OXTR gene has been the target of several studies on the association with ASD, many of which have shown positive associations with different variants of the gene (LoParo and Waldman 2014) .
The reelin (RELN) gene is located at chromosome 7q22 and it encodes a large protein from the extracellular matrix involved in the control of cell–cell interactions essential for cellular positioning and neuronal migration during brain development. The RELN gene is involved in different neurogenetic diseases such as autism, schizophrenia, bipolar disorder, and lissencephaly syndrome (Wang et al. 2014; Fatemi et al. 2000; Hong et al. 2000) . Genetic variants have been positively associated with occurrences of autism in different studies (Serajee et al. 2006; Li et al. 2008; Ashley-Koch et al. 2007; Sharma et al. 2013) .
The MET gene is located at 7q31 and it encodes the tyrosine kinase MET receptor, which is activated by the hepatocyte growth factor (HGF). Besides its initially established role as a proto-oncogene that operates in the development of various human cancers, MET also has a key role in early brain development, in the autoimmune system, and in gastrointestinal repair (Hedrick et al. 2012) . The MET gene has been indicated as a candidate gene for autism due to its involvement in pathways related to the development of the brain cortex and cerebellum cortex (Zhou et al. 2011) . Different common variants were evaluated in association studies, which showed SNP rs1858830 (C/G) in the MET promoter region as being one of the main gene variants associated with autism (Zhou et al. 2011).
The Engrailed-2 (EN2) gene is a homeobox transcription factor with a key role in the development of the midbrain and cerebellum. EN2 was identified as a candidate gene for ASD, due to it being located at chromosome 7q36.3, a locus of susceptibility to ASD, and also because EN2 mutant mice have exhibited morphological abnormalities of the cerebellum, similar to autistic individuals (Baader et al. 1998; Kuemerle et al. 1997; Wang et al. 2008) .
However, most associations with candidate genes are not conclusive, and the data analysis and meta-analyses in various studies show conflicting and inconsistent findings. Although there are many studies showing positive associations, the majority was not replicated in different studies or populations.
The genome scan studies or genome-wide association studies (GWAS) evaluate, on a large scale, different genome variants, with no a priori definition of a biological hypothesis. This approach is very interesting for suggesting new genes and metabolic pathways in research on psychiatric disorders (like ASD), distinct from investigations of candidate genes. However, the common disease–common variant genetic approach that supports this kind of study may hinder the replication of results in many heterogeneous disorders. Few positive findings have been replicated independently across the studies—there is always a small effect associated with the risk of developing the disorder. The large number of markers analyzed in GWAS studies, which can surpass 500,000, increases the number of tests, resulting in the need for very strict data correction. The cutoff points used for statistical significance are low (e.g., P < 5 × 10 − 8) (Wang et al. 2009) and are very difficult to achieve in genes that have little effect.
Considering the latest published works, only the 5p14.1 region was associated with ASD in more than one investigation. This region encompasses the genes CDH10 (cadherin 10, type 2) and CDH9 (cadherin 9, type 2), which encode the cadherins responsible for cell–cell adhesion (Wang et al. 2009; Ma et al. 2009) . Other GWAS performed so far only found suggestive or significant results that have not been replicated. The main findings of these studies involve genes related to CAMs, synaptogenesis, apoptosis, cell proliferation, coagulation, cancer, the neurotransmitter glutamate, and some intergenic regions (Anney et al. 2010; Jones et al. 2013; Ronald et al. 2010; Shi et al. 2013) .
Common variants addressed by association studies generally have little effect on the disease etiology or characterization. The large complexity observed in heterogeneous populations and disorders is intimately connected to the size of the variable effect and the sample size investigated, sometimes hindering the study’s findings. Moreover, the lack of functional evidence in some variants analyzed may also be contributing to this scenario. The use of other methodologies could, therefore, provide additional candidate genes for investigation.
3.3.3 Exome Studies
Exoma analysis involves the sequencing of all exons in the genome and it has been widely employed over the past few years. Its goal is to search for rare genetic variants associated with diseases. In ASD, several mutations have been described and associated with different biological mechanisms, such as chromatin structure, development of the nervous system, serotonin and glutamate neurotransmission, cell migration and organization, sodium channels, and ubiquitination (Bi et al. 2012; O’Roak et al. 2012; Neale et al. 2012; Toma et al. 2013; Sanders et al. 2012) . Exoma analyses, like GWAS, have also been helpful in suggesting the involvement of new genes in the etiology of ASD. The results have also allowed, through use of bioinformatic tools, the description of complex biological pathways, integrating the new findings with the “classical” genes analyzed in studies with candidate genes, thus underscoring the importance of these studies.
3.3.4 Copy Number Variation (CNV) Studies
The evaluation of copy number variations (CNVs) involves the detection of rare variations in some regions on the genome. It has been widely used in psychiatric genetics. These variations may be duplications, or insertions or deletions (inherited or not), ranging in size from 1 kilobase (kb) to several megabases (Mb). Studies with different samples suggest that CNVs are associated with the normal variability of the human genome (Abecasis et al. 2012) . However, they are considered to be a risk factor for some complex diseases, including ASD, and it is suggested that genes involved in behavioral aspects have a dose-dependent effect in accordance with the number of copies that are present.
In ASD, the CNVs are located throughout the genome at different chromosomes. The frequency of CNVs varies between 5 and 10 % in ASD case subjects, and 1 and 2 % in control subjects. Levy et al. (2011) analyzed the presence of CNVs in 28 ASD children and 62 healthy adults—they observed 38 CNVs. In all case subjects, at least one CNV was found, whereas in the control group the presence of such variants was found in only 11.3 % of people. Moreover, 14 CNVs were found in both groups, 19 were observed only in ASD case subjects, and 5 were observed only in the control group. Even with the remarkable difference in the CNV frequencies between the case and control groups, it is important to note that there are common results in healthy and unhealthy individuals. Furthermore, similar results have recently been described in different psychiatric disorders—mostly in autism, schizophrenia, and cognitive deficits, including the de novo mutations (Stefansson et al. 2013) .
Often, the duplication in the 15q11–13 region has been associated with ASD, mental deficiency, seizures, and behavioral and developmental abnormalities. Another alteration observed in approximately 1 % of ASD cases is related to the 16p11.2 region, involving both duplications and deletions. Nonetheless, in both cases, these CNVs are not exclusive to ASD and can be observed in individuals diagnosed with other diseases. Also, the CNVs appear to not have complete penetrance and their pathogenic effect can vary according to the presence or absence of other genetic and environmental alterations (Stefansson et al. 2013). Some results have previously been associated with genetic syndromes, like Prader–Willi and DiGeorge (Glessner et al. 2009; Marshall et al. 2008; Pinto et al. 2010; Sanders et al. 2011; Sebat et al. 2007; Shishido et al. 2013) . These studies are important to confirm or suggest new metabolic pathways such as neuronal cell adhesion and ubiquitin (Glessner et al. 2009).
The development of new techniques and methods in molecular and genetic research has resulted in an increase in information and knowledge regarding ASD, allowing new approaches to elucidate the etiology. It is known that the biological mechanisms involved in different phenotypes do not act in an isolated way, but interact with each other. The gene–gene interaction is a way of evaluating these epistatic effects. These interactions often involve prior biological hypotheses, constructed from biochemical and molecular studies. However, this approach has been little used in association studies (candidate gene or genome scan), and little solid evidence has been obtained. These more complex analyses may help us unravel the etiology of disorders like ASD, thus clarifying the biological processes involved.
Furthermore, an interesting study conducted by Poelmans et al. (2013) compiled the data obtained by studies using these different approaches in an attempt to make a more complete, comprehensive, and accurate interpretation of these bioinformatic analysis data. The authors evaluated the results of GWAS, CNV, exoma studies, and other genetic data. Through the enrichment of networks, important results involving the regulation of steroids, neurite outgrowth, and glutamatergic synapses were described. These analyses will be of great value, since the gathering and reviewing of results from different studies can highlight the most relevant information.
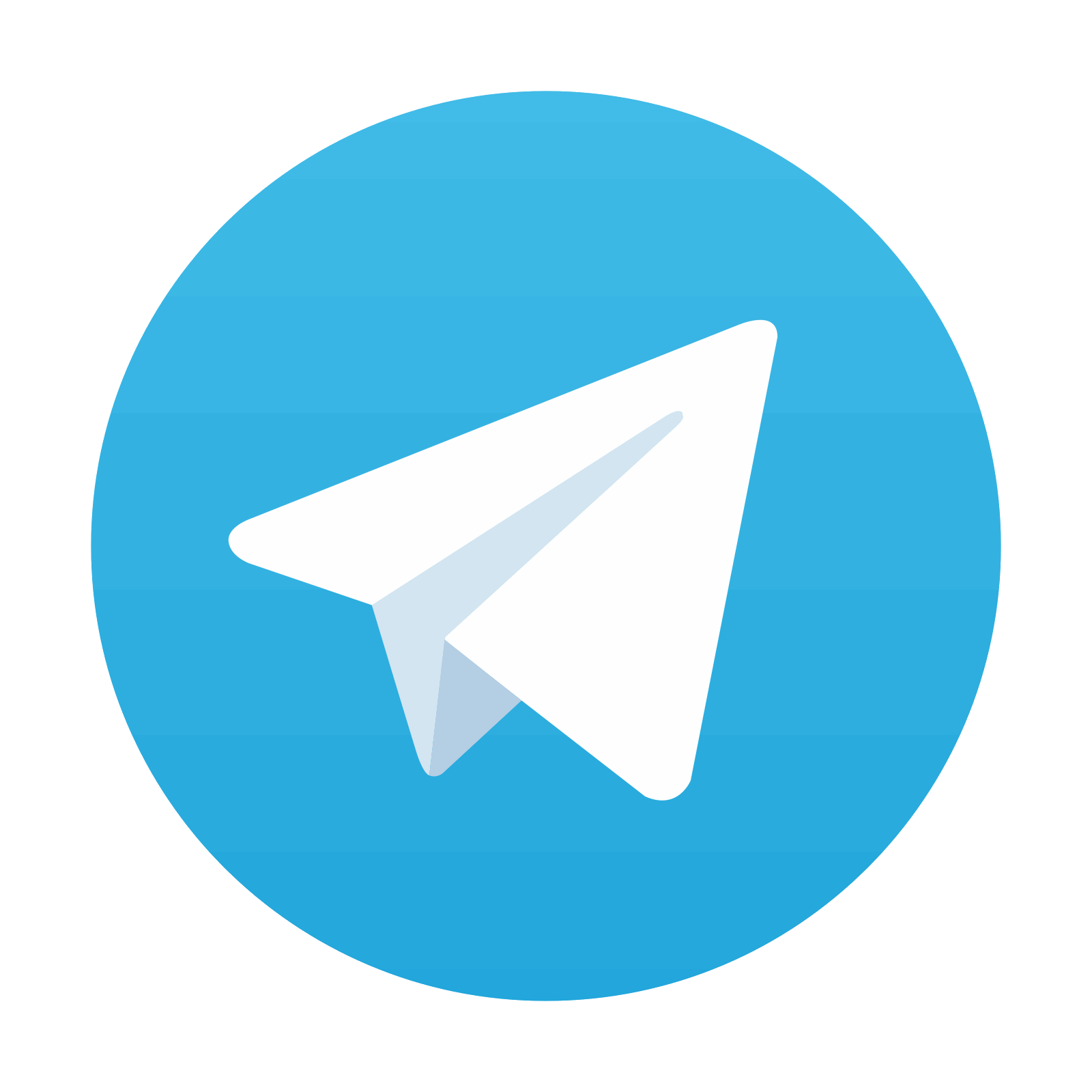
Stay updated, free articles. Join our Telegram channel

Full access? Get Clinical Tree
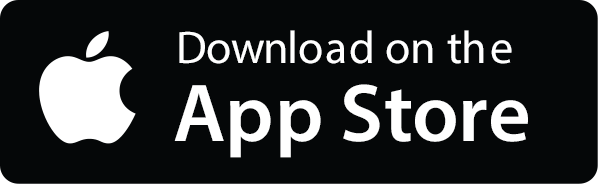
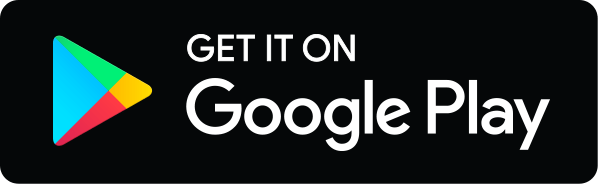